Developmental Biology Lesson 25 PDF
Document Details
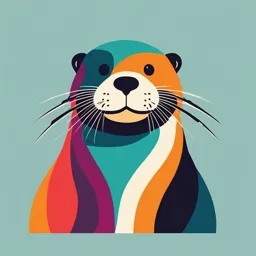
Uploaded by LustrousBongos5217
Augustana University
Tags
Summary
This document provides a detailed lecture on developmental biology, specifically focusing on the intermediate and lateral plate mesoderm, and its role in heart, blood, and kidney development. Diagrams and figures illustrate the different stages and processes involved in this complex biological process.
Full Transcript
BIOL 346 Developmental Biology Lesson 25 Chapter 20: Intermediate and Lateral Plate Mesoderm: Heart, Blood, and Kidneys Fig 20.1: Major lineages of the amniote mesoderm Mesodermal lineages determined by amount of BMP4-low centrally/medially, hig...
BIOL 346 Developmental Biology Lesson 25 Chapter 20: Intermediate and Lateral Plate Mesoderm: Heart, Blood, and Kidneys Fig 20.1: Major lineages of the amniote mesoderm Mesodermal lineages determined by amount of BMP4-low centrally/medially, high laterally Intermediate Mesoderm Kidney Lateral plate mesoderm Heart, blood vessels, blood Fig 20.2: General scheme of development in the vertebrate kidney Stages of Kidney Development __Pronephros Development: Pronephros: Initial kidney tubules form Mesonephros Development: Pronephros undergoes apoptosis Mesonephros forms: Second set of kidney tubules Metanephros Development Mesonephros undergoes apoptosis Metanephros forms: Final kidney tubules Kidneys form through reciprocal interactions between metanephrogenic mesenchyme and the ureteric bud Fig. 20.4: Reciprocal induction in the development of the mammalian kidney Metanephric mesenchyme induces bud to branch Mesenchyme condenses around ureteric bud branch tips Mesenchyme aggregates, cavitates, forms epithelium Two epithelia come together to form nephron and collecting duct for urine Fig 20.5: Kidney branching seen in vitro Kidney tubule branching increases significantly over time. Molecular mechanisms of reciprocal induction: Fig. 20.7: Ureteric bud growth is dependent on GDNF and its receptors No Ret= green cells _Ret-expressing = blue cells (Ret is GDNF receptor) GDNF = Glial-derived neurotrophic factor Secreted from metanephric mesenchyme Causes outgrowth of ureteric bud from nephric duct cells express Ret (GDNF receptor) Fig. 20.8: Effect of glial-derived neurotrophic factor on branching of the ureteric epithelium _Ureteric bud and its branches (orange); Nephrons (green) GDNF signaling from metanephric mesenchyme induces branching of ureteric bud (A): A 13-day mouse kidney cultured with a control bead (dashed circle) shows a normal branching pattern. (B): A similar kidney cultured with a GDNF-soaked bead displays an altered branching pattern, with additional branches induced near the bead. Fig. 20.9: Wnts are critical for kidney development Multiple Ants are expressed in ureteric bud-in stalk or at tips Without Wnts, kidneys are absent Wnts promote condensation of mesenchyme around ureteric bud and mesenchymal to epithelial transition of mesenchyme to form nephron Circulatory System Development Circulatory system Formed from lateral plate mesoderm Includes heart, blood vessels, blood First working unit in embryo Heart development: First functional organ Heart progenitor cells migrate to form 2 heart fields (or cardiogenic mesoderm) Figure 20.13 Heart fields in the mouse embryo Figure 20.14 Model of inductive interactions involving the BMP and Wnt pathways that form the boundaries of the cardiogenic mesoderm Wnt signals: From neural tube, guide lateral plate mesoderm (LPM) to form blood and blood vessels. Wnt inhibitors: In the anterior, endoderm releases Dkk, Crescent, and Cerberus to block Wnt, allowing BMP and Fgf8 to turn LPM into cardiogenic mesoderm. BMP Role: Essential for differentiating both heart and blood cells. Noggin and Chordin: From notochord in the center, block BMP, preventing heart and blood field formation in the middle of the embryo. Fig. 20.15: Migration of heart primordia Cardiac progenitors migrate anteriorly and medially between ectoderm and endoderm to form heart fields. Fibronectin secreted by the endoderm guides cardiac precursors during migration. Initially, there are two separate heart fields on each side, which later fuse to form a single heart. (A) Chick embryo with cardia bifida: ventral midline cut prevents fusion, resulting in two separate hearts. (B) Wild-type zebrafish embryo showing normal heart primordia migration. (C) Miles apart mutant zebrafish lacks heart primordia migration. (D) Normal mouse embryo (13.5 days) with fused heart primordia. (E) Foxp4-deficient mouse embryo with cardia bifida: two hearts form with ventricles, atria, and correct left-right asymmetry, but remain unfused. Fig. 20.16: Retinoic acid regulates Hox gene expression to control cardiac cell identity _Posterior portions of heart fields express retinoid acid Retinoic acid gradient regulates expression of Hox genes Specific Hox genes promote regional identities within heart fields Raldh2 (orange): Marks retinoic acid-producing regions. Tbx5 (purple): Highlights early heart fields Fig. 20.18: Cardiac looping and chamber formation Early Heart Tube Development: By day 21, the human heart is a single- chambered tube with specified regions that will form different chambers. Cardiac Looping: By day 28, looping positions the future atria anterior to the ventricles, establishing the heart’s right-left orientation. Show specific gene expression prior to looping, after looping, further differentiation of chambers occurs Valve formation: Endocardial cushions develop at the atrioventricular canal and outflow tract, guided by Twist gene activation and neural crest cells. Birth Transition: First breath redirects blood flow from placenta to lungs, closing fetal shunts and completing heart maturation. Fig. 20.19: Vasculogenesis and angiogenesis _Vasculogenesis__: Initial formation of blood islands with hemangioblasts that create primary capillary networks. Amgiogenesis: Extends vasculogenesis by remodeling and expanding the network, forming new blood vessels from existing ones. Key factors: Paracrine signals guide both processes; specific receptors on vessel-forming cells respond to these signals to drive vessel formation and growth. Fig. 20.20: Vasculogenesis _Extraembryonic Vasculogenesis: Occurs in yolk sac blood islands, forming early vasculature and red blood cells to support the embryo. Yolk Sac Blood Islands: May also generate definitive (adult) blood stem cells (mouse studies). _Intraembryonic Vasculogenesis: Forms the dorsal aorta, connecting with capillary networks in each organ. Dorsal Aorta Scaffold: Derived from somite cells migrating ventrally. Fig. 20.21: VEGF and its receptors in mouse embryos _VEGF Signaling Regulates Angiogenesis_________________ ____________________________ __________: VEGF (vascular endothelial growth factor) drives the remodeling of capillary networks into veins and arteries. _VEGF Induces Endothelial Migration: Secreted in response to hypoxia or from organs, VEGF stimulates endothelial cells to expand capillary networks. Tip Cells Respond to VEGF: Endothelial tip cells with VEGF receptors use filopodia to sprout new vessels. Balance of VEGF is Crucial: Abnormal VEGF levels can lead to diseases, including pre-eclampsia Fig. 20.22: Pathway for hematopoietic stem cell formation Hemtopoiesis: The process of generating blood cells from stem cells. _Stem Cell Division: Hematopoietic stem cells (HSCs) divide to produce both new stem cells and progenitor cells, which mature into various blood cell types. _Hematopoietic Stem Cells __________________________Pluripotent cells capable of producing all types of blood cells and lymphocytes in the body. __Origin of HSCs__________________: Adult HSCs originate from the aorta-gonad-mesonephros (AGM) region (ventral aorta) and later migrate to the bone marrow. Fig. 20.23: The home for HSCs appears to be a niche where stem cell factor (SCF) can be made by the perivascular (subendothelial) cells as well as by the endothelial cells of the bone marrow sinusoids __HSC Migration: Hematopoietic stem cells (HSCs) migrate to the bone marrow. _Bone Marrow Niche_ Bone marrow serves as the specialized niche for HSCs. _Maintenance of HSC Fate_: Stem cell factor (SCF), secreted by perivascular and endothelial cells in the bone marrow, helps maintain HSCs in their undifferentiated state. Fig. 20.24: Hierarchy of hematopoietic lineages