Exercise Physiology Theory and Application PDF
Document Details
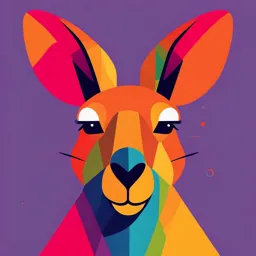
Uploaded by DeservingPoplar
University of Victoria
2018
Scott K. Powers and Edward T. Howley
Tags
Summary
Exercise Physiology: Theory and Application to Fitness and Performance, tenth edition, by Scott K. Powers and Edward T. Howley, published in 2018, covers exercise metabolism and its effects on the human body during various exercises. It discusses oxygen uptake, fuel selection, and the factors that regulate them, including diet, exercise intensity, and the effects of training and prolonged exercise.
Full Transcript
EXERCISE PHYSIOLOGY Theory and Application to Fitness and Performance TENTH EDITION Scott K. Powers University of Florida Edward T. Howley University of Tennessee, Knoxville EXERCISE PHYSIOLOGY: THEORY AND APPLICATION TO FITNESS AND PERFORMANCE, TENTH EDITION Published by McGraw-Hill Education,...
EXERCISE PHYSIOLOGY Theory and Application to Fitness and Performance TENTH EDITION Scott K. Powers University of Florida Edward T. Howley University of Tennessee, Knoxville EXERCISE PHYSIOLOGY: THEORY AND APPLICATION TO FITNESS AND PERFORMANCE, TENTH EDITION Published by McGraw-Hill Education, 2 Penn Plaza, New York, NY 10121. Copyright © 2018 by McGraw-Hill Education. All rights reserved. Previous editions © 2015, 2012, and 2009. No part of this publication may be reproduced or distributed in any form or by any means, or stored in a database or retrieval system, without the prior written consent of McGraw-Hill Education, including, but not limited to, in any network or other electronic storage or transmission, or broadcast for distance learning. Some ancillaries, including electronic and print components, may not be available to customers outside the United States. 1 2 3 4 5 6 7 8 9 LWI 21 20 19 18 17 ISBN 978-1-259-87045-3 MHID 1-259-87045-6 Chief Product Officer, SVP Products & Markets: G. Scott Virkler Vice President, General Manager, Products & Markets: Michael Ryan Vice President, Content Design & Delivery: Betsy Whalen Managing Director: Katie Stevens Brand Manager: Jamie Laferrera Director, Product Development: Meghan Campbell Product Developer: Francesca King Marketing Manager: Meredith Leo Director, Content Design & Delivery: Terri Schiesl Program Manager: Jennifer Shekleton Content Project Managers: Ryan Warczynski, George Theofanopoulos, Sandra Schnee, Rachel Townsend Buyer: Jennifer Pickel Design: Studio Montage Content Licensing Specialist: Melisa Seegmiller, Brianna Kirschbaum, Ann Marie Jannette Cover Image: © Erik Isakson/Blend Images LLC, © technotr/Getty Images, Ingram Publishing, monkeybusinessimages/iStockphoto/Getty Images Compositor: Lumina Datamatics, Inc. Printer: LSC Communications All credits appearing on page or at the end of the book are considered to be an extension of the copyright page. Library of Congress Cataloging-in-Publication Data Names: Powers, Scott K. (Scott Kline), 1950—author. | Howley, Edward T., 1943—author. Title: Exercise physiology : theory and application to fitness and performance / Scott K. Powers, Edward T. Howley. Description: Tenth edition. | New York, NY : McGraw-Hill Education, | Includes bibliographical references and index. Identifiers: LCCN 2016051329 | ISBN 9781259870453 (pbk. : alk. paper) | ISBN 9781259982651 (ebook) Subjects: MESH: Exercise—physiology | Physical Fitness Classification: LCC QP301 | NLM QT 256 | DDC 612/.044—dc23 LC record available at https://lccn.loc.gov/2016051329 The Internet addresses listed in the text were accurate at the time of publication. The inclusion of a website does not indicate an endorsement by the authors or McGraw-Hill Education, and McGraw-Hill Education does not guarantee the accuracy of the information presented at these sites. mheducation.com/highered 4 © Action Plus Sports Images/Alamy Stock Photo Exercise Metabolism Objectives By studying this chapter, you should be able to do the following: 1. Discuss the relationship between exercise (graded) exercise test and identify the lactate intensity/duration and the bioenergetic threshold. pathways that are most responsible for the 6. Discuss several possible explanations for the production of ATP during various types of sudden rise in blood-lactate concentration exercise. during incremental exercise. 2. Describe graphically the change in oxygen 7. List the factors that regulate fuel selection uptake during the transition from rest to during exercise. steady-state exercise and then recovery from 8. Describe the changes in the respiratory exercise. Identify the oxygen deficit, oxygen exchange ratio (R) with increasing intensities requirement, and oxygen debt (excess post- of exercise and during prolonged exercise of exercise oxygen uptake). moderate intensity. 3. Describe graphically the changes in oxygen 9. Describe the types of carbohydrates and fats uptake during an incremental (graded) used during increasing intensities of exercise exercise test and identify the maximal oxygen and during prolonged exercise of moderate uptake (V̇O2 max). intensity. 4. Describe the various criteria for having 10. Describe the lactate shuttle, with examples of achieved (V̇O2 max). how it is used during exercise. 5. Describe graphically the changes in the blood lactate concentration during an incremental Outline Key Terms Energy Requirements at Estimation of Fuel Utilization anaerobic threshold Rest 69 during Exercise 80 Cori cycle excess post-exercise oxygen Rest-to-Exercise Transitions 69 Factors Governing Fuel consumption (EPOC) Recovery from Exercise: Selection 81 free fatty acids (FFAs) Metabolic Responses 71 Exercise Intensity and Fuel gluconeogenesis Selection 81 graded (or incremental) Metabolic Responses to Exercise: exercise test Exercise Duration and Fuel Influence of Duration and incremental exercise test Selection 82 lactate threshold Intensity 75 Interaction of Fat/ lipase Short-Term, Intense Carbohydrate lipolysis Exercise 75 maximal oxygen uptake Metabolism 84 Prolonged Exercise 75 (V̇O2 max) Body Fuel Sources 84 Incremental Exercise 76 oxygen debt oxygen deficit respiratory exchange ratio (R) 68 E xercise poses a serious challenge to the bioenergetic pathways in the working muscle. For example, dur- ing heavy exercise, the body’s total energy expendi- to 7 mph, and in that one step your muscles increase their rate of ATP production from that required for standing to that required for running at 7 mph (see ture may increase 15 to 25 times above expenditure at Fig. 4.1(a) and (b)). If ATP production did not increase rest. Most of this increase in energy production is used instantaneously, you would have fallen off the back of to provide ATP for contracting skeletal muscles, which the treadmill! What metabolic changes must occur in may increase their energy utilization 200 times over skeletal muscle at the beginning of e xercise to provide utilization at rest (1). Clearly, skeletal muscles have a great capacity to produce and use large quantities (a) of ATP during exercise. This chapter describes (a) the Rest Exercise metabolic responses at the beginning of exercise and 8 during recovery from exercise; (b) the metabolic re- sponses to high-intensity, incremental, and prolonged 7 exercise; (c) the selection of fuels used to produce ATP; 6 and (d) how exercise metabolism is regulated. Speed (mph) 5 We begin with an overview of the energy re- quirements of the body at rest, followed by a discus- 4 sion of which bioenergetic pathways are activated at 3 the onset of exercise. 2 1 ENERGY REQUIREMENTS AT REST –2 –1 0 1 2 3 4 5 (b) Recall that homeostasis is defined as a steady and an unchanging internal environment (see Chap. 2). Dur- Rest Exercise 0.8 ATP requirement for 7 mph ing resting conditions, the healthy human body is in homeostasis, and therefore the body’s energy require- 0.7 ATP use (moles · min– 1) ment is also constant. At rest, almost 100% of the 0.6 energy (i.e., ATP) required to sustain bodily functions ATP supply = ATP demand 0.5 is produced by aerobic metabolism. It follows that resting blood lactate levels are also steady and low 0.4 (e.g., 1.0 millimole per liter). 0.3 Because the measurement of oxygen (O2) con- sumption (oxygen consumed by the body) is an in- 0.2 ATP for standing dex of aerobic ATP production, measurement of O2 0.1 consumption during rest provides an estimate of the body’s “baseline” energy requirement. At rest, the to- –2 –1 0 1 2 3 4 5 tal energy requirement of an individual is relatively low. For example, a 70-kilogram young adult would (c) consume approximately 0.25 liter of oxygen each Rest Exercise minute; this translates to a relative O2 consumption of 3.5 3.5 ml of O2 per kilogram (body weight) per minute. 3 As mentioned earlier, muscular exercise can greatly 2.5 O2 deficit ˙ 2 (L · min– 1) increase the body’s need for energy. Let’s begin our ˙ 2 Steady-state VO discussion of exercise metabolism by considering 2 which bioenergetic pathways are active during the 1.5 transition from rest to exercise. VO 1 ˙ 2 Standing VO 0.5 REST-TO-EXERCISE TRANSITIONS –2 –1 0 1 2 3 4 5 Time (min) Assume you are standing alongside a treadmill with Figure 4.1The time course of speed (a), rate of ATP use its belt moving at 7 mph, and you jump onto the belt (b), and oxygen uptake (V̇O2) (c) in the transition from and start running. Within one step, you go from zero rest to submaximal exercise. Chapter Four Exercise Metabolism 69 the necessary energy to continue movement? Similar (a) to the measurement of O2 consumption during rest, 50 Exercise the measurement of O2 consumption during exercise 40 can provide information about aerobic metabolism during exercise. For example, in the transition from PCr (mM) 30 rest to light or moderate exercise, O2 consumption increases rapidly and reaches a steady state within 20 one to four minutes (12, 22, 99) (Fig. 4.1(c)). The fact that O2 consumption does not in- 10 crease instantaneously to a steady-state value 0 means that anaerobic energy sources contribute 0 3 6 9 12 15 18 21 24 to the overall production of ATP at the begin- Time (min) ning of exercise. Indeed, much evidence suggests (b) that at the onset of exercise, the ATP-PC system 9 Rate of ATP production from PCr hydrolysis (mM/min/W) is the first active bioenergetic pathway, followed 8 7 by glycolysis and, finally, aerobic energy produc- 6 tion (4, 17, 41, 73, 104). This is shown in Figure 5 4.2(a), in which the PC concentration in muscle 4 falls dramatically during a three-minute bout of ex- 3 ercise. Figure 4.2(b) shows that the production of 2 ATP from PC was the highest in the first minute of 1 exercise and fell off after that, due in part to the 0 systematic reduction in the PC store. Figure 4.2(c) 1 2 3 shows that glycolysis was already contributing ATP Time (min) (c) during the first minute of exercise and increased 20 during the second minute (10). The effectiveness of Rate of glycogenolytic ATP 18 production (mM/min/W) these two anaerobic systems in the first minutes of 16 exercise is such that ATP levels in muscle are virtu- 14 ally unchanged, even though ATP is being used at 12 a much higher rate (120). However, as the steady- 10 state O2 consumption is reached, the body’s ATP re- 8 6 quirement is met via aerobic metabolism, indicating 4 that ATP is being produced aerobically as quickly as 2 it is being used. The major point to be emphasized 0 concerning the bioenergetics of rest-to-work transi- 1 2 3 Time (min) tions is that several energy systems are involved. In other words, the energy needed for exercise is not Figure 4.2 Figure (a) shows the rapid change of phos- provided by simply turning on a single bioenergetic phocreatine (PC) concentration in muscle during a pathway, but rather by a mixture of several meta- three-minute bout of exercise. Figures (b) and (c) show bolic systems operating with considerable overlap. the rate of ATP production during this three-minute The term oxygen deficit applies to the lag in bout from PC and glycolysis, respectively. oxygen uptake at the beginning of exercise. Specifi- cally, the oxygen deficit is defined as the difference between oxygen uptake in the first few minutes Trained adults (64, 99) and adolescents (88) reach of exercise and an equal time period after steady the steady-state V̇O2 faster than untrained subjects, state has been obtained (90, 97). During this time, resulting in a smaller oxygen deficit. What is the ex- much of the ATP is supplied anaerobically (ATP-PC planation for this difference? It seems likely that the system and glycolysis) since aerobic ATP produc- trained subjects have a better developed aerobic bio- tion is insufficient until the oxygen uptake reaches energetic capacity, resulting from either cardiovas- steady state. This is represented as the shaded area cular or muscular adaptations induced by endurance in the left portion of Figure 4.1(c). What causes this training (33, 64, 97, 99). Practically speaking, this lag in oxygen uptake at the onset of exercise? Is it means that aerobic ATP production is active earlier at due to inadequate oxygen delivery to the muscle, the beginning of exercise and results in less produc- or is it a failure of oxidative phosphorylation to in- tion of lactate and H+ in the trained individual when crease immediately when exercise begins (82)? Dr. compared to the untrained individual. Chapter 13 Bruce Gladden answers those questions in Ask the provides a detailed analysis of this adaptation to Expert 4.1. training. 70 Section One Physiology of Exercise ASK THE EXPERT 4.1 Oxygen Uptake Kinetics at the Onset of Constant Work-Rate Exercise: Questions and Answers with Dr. Bruce Gladden Bruce Gladden, Ph.D., anaerobic energy systems must also down, gradually providing additional a professor in the School be activated to supply the needed stimulation to “turn on” oxidative of Kinesiology at Auburn energy at the beginning of exercise; phosphorylation until this aerobic University, is an interna- that is, there is an oxygen deficit. pathway is providing essentially 100% tionally known expert in question: Why is oxidative phosphor- of the energy requirement of the ex- muscle metabolism during ylation not fully activated instan- ercise. The key point is that these exercise. Dr. Gladden’s taneously at the onset of exercise? stimulators of oxidative phosphory- Courtesy of research has addressed answer: Historically, two alternative lation rate do not instantaneously Bruce Gladden important questions rise from resting concentrations to hypotheses have been offered. First, relative to those factors it has been suggested that there is the steady-state concentration levels. that regulate oxygen consumption in skeletal an inadequate oxygen supply to the This has sometimes been referred to muscle during exercise. Examples of Dr. Glad- contracting muscles at the onset of as the “inertia of metabolism.” den’s work can be found in prestigious interna- exercise. What this means is that in question: Which of the two hypoth- tional physiology journals. In this feature, Dr. at least some mitochondria, at least eses is correct? Gladden answers questions about the time some of the time, there may not be answer: This is a difficult question be- course of oxygen consumption at the onset of molecules of oxygen available to ac- cause the two hypotheses are not submaximal exercise. cept electrons at the end of the elec- mutually exclusive. My research tron transport chains. Clearly, if this with numerous colleagues (espe- question: What is so important about is correct, the oxidative phosphory- cially Drs. Bruno Grassi, Mike Hogan, the oxygen consumption response lation rate, and therefore the whole- and Harry Rossiter) provides support at the onset of submaximal, con- body oxygen consumption, would for the second hypothesis (i.e., “slow- stant work-rate exercise? be restricted. The second hypothesis ness” in the stimuli required to fully answer: First, it is critical to realize holds that there is a delay because the activate oxidative phosphorylation). that O2 consumption is a reliable stimuli for oxidative phosphorylation In particular, there is evidence for a indicator of the energy supplied require some time to reach their final significant role of the creatine kinase by oxidative phosphorylation (i.e., levels and to have their full effects reaction in “buffering” some of the the oxidative or aerobic energy sys- for a given exercise intensity. As dis- most important signals (e.g., ADP con- tem). The fact that there is a “lag” cussed in Chap. 3, the electron trans- centration) for activating oxidative or delay before oxygen consump- port chain is stimulated by ADP and phosphorylation. Nevertheless, oxy- tion rises to a steady-state level Pi (e.g., Table 3.3). Shortly after the gen supply limitation can also play a tells us that aerobic metabolism onset of exercise, the concentrations role, a role that likely becomes more (i.e., oxidative phosphorylation in of ADP and Pi are barely above resting important at higher exercise intensi- the mitochondria) is not fully acti- levels because the ATP concentration ties. None of the possible regulators vated at the onset of exercise. The is being maintained by the creatine or controllers of oxidative phosphor- importance of this response is that kinase reaction (PC + ADP → ATP + C) ylation should be considered sepa- it can provide information about and accelerated glycolysis (with lac- rately. All of them interact with each the control or regulation of oxida- tate accumulation). However, the other to provide the overall stimulus tive phosphorylation. Further, this concentrations of ADP and Pi will to oxidative phosphorylation under delayed response tells us that the continue to rise as PC is broken any given exercise condition. IN SUMMARY RECOVERY FROM EXERCISE: In the transition from rest to light or moderate ex- METABOLIC RESPONSES ercise, oxygen uptake increases rapidly, generally reaching a steady state within one to four minutes. If a subject is running on the treadmill at a speed that The term oxygen deficit applies to the lag in can be maintained comfortably for 20 or 30 minutes, oxygen uptake at the beginning of exercise. and then jumps off to the side, the metabolic rate The failure of oxygen uptake to increase in- (V̇O2) does not fall instantaneously from the steady- stantly at the beginning of exercise means that state value measured during the exercise to the resting anaerobic pathways contribute to the overall V̇O2 value associated with standing by the treadmill. production of ATP early in exercise. After a The metabolic rate remains elevated for several min- steady state is reached, the body’s ATP require- utes immediately following exercise. This is shown in ment is met via aerobic metabolism. Figure 4.3(a), with a steady-state V̇O2 being achieved Chapter Four Exercise Metabolism 71 (a) 4.5 4 Subject’s highest attainable VO2 (VO˙ 2 max) 3.5 3 ˙ 2 Steady-state VO ˙ 2 (L · min−1) 2.5 O2 deficit Rapid 2 Aerobic ATP component VO supply meets 1.5 ATP demand Slow component 1 ˙ 2 Resting VO baseline EPOC 0.5 −4 −2 0 2 4 6 8 10 12 14 Time (min) (b) 5 O2 requirement 4.5 4 O2 deficit End of exercise 3.5 Rapid 3 component ˙ 2 (L · min−1) ˙ 2 Highest VO 2.5 attainable VO 2 1.5 Slow component Figure 4.3 Oxygen 1 ˙ 2 EPOC Resting VO deficit and the ex- baseline cess post-exercise O2 0.5 consumption (EPOC) during moderate exercise (a) and dur- −4 −2 0 2 4 6 8 10 12 14 16 18 20 22 ing heavy, exhausting Time (min) exercise (b). by minute 3 of submaximal exercise, and the oxygen is still not back to the resting level by 14 minutes after uptake returning to resting levels by five minutes after exercise. Clearly, the magnitude and duration of this exercise. This means that, for several minutes after ex- elevated post-exercise metabolic rate are influenced by ercise, the oxygen uptake is above the level needed to the intensity of the exercise (6, 53, 97). The reason(s) meet the demands of standing on the treadmill. In con- for this observation will be discussed shortly. trast, Figure 4.3(b) shows the subject doing nonsteady- Historically, the term oxygen debt has been state exercise that can be maintained for only six min- applied to the elevated oxygen uptake (above rest- utes before exhaustion occurs. This test is set at an ing levels) following exercise. The prominent Brit- intensity that exceeds the highest V̇O2 the subject can ish physiologist A. V. Hill (65) first used the term attain (V̇O2 max). In this case, the subject could not O2 debt and reasoned that the excess oxygen con- meet the oxygen requirement for the task as shown by sumed (above rest) following exercise was repayment the much larger oxygen deficit, and the subject’s V̇O2 for the O2 deficit incurred at the onset of exercise 72 Section One Physiology of Exercise A LOOK BACK—IMPORTANT PEOPLE IN SCIENCE A.V. Hill Was a Pioneer in Exercise Physiology Archibald Vivian (chemistry, physics, and physiology) muscle can produce energy anaerobi- (A.V.) Hill (1886– at Trinity College, Cambridge. cally. This work was important because 1977) received the After graduation, Hill was encour- the general view at the time was that Nobel Prize in Physi- aged to focus on physiology by one of all energy for muscular performance ology or Medicine his teachers, Dr. Walter Fletcher. Hill ini- was derived from aerobic metabolism. in 1922 for his re- tiated his physiology research career at A.V. Hill also introduced the concept of search on heat pro- Cambridge in 1909 and began to investi- the oxygen debt (i.e., oxygen consump- © AP Images duction in skeletal gate the nature of muscular contraction tion following exercise), and he was muscle. This work using frog muscle as a model. Hill later the first scientist to clearly describe the precisely described the magnitude of held faculty positions at Manchester concept of maximal oxygen consump- heat production in isolated skeletal University (1920–1923) and at Univer- tion in exercising humans. Other im- muscle during both contraction and sity College, London (1923–1951). portant scientific contributions of Hill relaxation. Professor Hill was born in In addition to his Nobel Prize win- include his discovery of heat produc- Bristol, England, and his family had ning research on heat production in tion in nerves and the force–velocity little money to support his educa- skeletal muscle, A.V. Hill made many equation in skeletal muscle. tion. Nonetheless, he was both mo- other contributions to our understand- For more information on A.V. Hill tivated and resourceful as he earned ing of exercise physiology. For exam- and his scientific contributions to ex- scholarships to support his studies ple, he was the first physiologist to ercise physiology, see the paper by in mathematics and natural sciences demonstrate that contracting skeletal Bassett in the Suggested Readings. (see A Look Back—Important People in Science). Evi- If the EPOC is not exclusively used to convert dence collected in the 1920s and 1930s by Hill and lactate to glucose, why does oxygen consumption other researchers in Europe and the United States remain elevated post-exercise? Several possibilities suggested that the oxygen debt could be divided into exist. First, at least part of the O2 consumed imme- two portions: the rapid portion immediately follow- diately following exercise is used to restore PC in ing exercise (i.e., approximately two to three minutes muscle and O2 stores in blood and tissues (12, 46). post-exercise) and the slow portion, which persisted Restoration of both PC and oxygen stores in mus- for greater than 30 minutes after some types of ex- cle is completed within two to three minutes of re- ercise. The rapid portion is represented by the steep covery (59). This is consistent with the classic view of decline in oxygen uptake following exercise, and the the rapid portion of the oxygen debt. Further, heart slow portion is represented by the slow decline in rate and breathing remain elevated above resting lev- O2 across time following exercise (Fig. 4.3(a) and (b)). els for several minutes following exercise; therefore, The rationale for the two divisions of the O2 debt was both of these activities require additional O2 above based on the belief that the rapid portion of the O2 resting levels. Other factors that may result in the debt represented the oxygen that was required to EPOC are an elevated body temperature and specific resynthesize stored ATP and PC and replace tissue circulating hormones. Increases in body temperature stores of O2 (~20% of the O2 debt), while the slow por- result in an increased metabolic rate (called the Q10 tion of the debt was due to the oxidative conversion effect) (19, 57, 101). Further, it has been argued that of lactate to glucose in the liver (~80% of the O2 debt). high levels of epinephrine or norepinephrine result Contradicting previous beliefs, current evidence in increased oxygen consumption after exercise (48). shows that only about 20% of the oxygen debt is used However, both of these hormones are rapidly removed to convert the lactate produced during exercise to glu- from the blood following exercise and therefore may cose (the process of glucose synthesis from noncarbo- not exist long enough to have a significant impact on hydrate sources is called gluconeogenesis) (14, 17). the EPOC. Several investigators have argued that the term oxygen Compared to moderate-intensity exercise, the debt be eliminated from the literature because the ele- EPOC is greater during high-intensity exercise be- vated oxygen consumption following exercise does not cause (53) appear to be entirely due to the “borrowing” of oxygen Heat production and body temperature are from the body’s oxygen stores (14, 15, 17, 46). In recent years, several replacement terms have been suggested. higher One such term is EPOC, which stands for “excess PC is depleted to a greater extent and more post-exercise oxygen consumption” (15, 46). O2 is required for its resynthesis Chapter Four Exercise Metabolism 73 A CLOSER LOOK 4.1 Removal of Lactate Following Exercise What happens to the lactate that is 12 formed during exercise? Classical the- No exercise ory proposed that the majority of the Light exercise Blood lactate concentration 10 ˙ 2 max) (35% VO post-exercise lactate was converted into glucose in the liver and resulted in an elevated post-exercise oxygen 8 (mmol/L) uptake (i.e., O2 debt). However, recent evidence suggests that this is not the 6 case and that lactate is mainly oxidized 4 Figure 4.4 Blood lactate re- after exercise (14, 17). That is, lactate is converted to pyruvate and used as moval following strenuous a substrate by the heart and skeletal Resting baseline exercise. Note that lactate 2 muscle. It is estimated that approxi- can be removed more rap- mately 70% of the lactate produced idly from the blood during during exercise is oxidized, while 20% Intense 10 20 30 40 recovery if the subject en- exercise gages in continuous light is converted to glucose and the remain- Recovery time (min) exercise. ing 10% is converted to amino acids. Figure 4.4 demonstrates the time course of lactate removal from the e xercise intensities would likely result Due to the increase in muscle blood following strenuous exercise. in an increased muscle production of oxidative capacity observed with Note that lactate removal is more lactate and therefore hinder removal. endurance training, some authors rapid if continuous light exercise is In one study, the intensity of the have speculated that trained subjects performed as compared to a resting active recovery was set at a percent- might have a greater capacity to re- recovery. The explanation for these age of the lactate threshold (LT), rather move lactate during recovery from findings is linked to the fact that light than a percentage of V̇O2 max. They intense exercise (7, 9). However, two exercise enhances the oxidation of lac- found that when subjects did their ac- well-designed investigations have re- tate by the working muscle (34, 47, tive recovery at intensities just below ported no differences in blood lac- 63). It is estimated that the optimum the LT (the point at which lactate ac- tate disappearance between trained intensity of recovery exercise to pro- cumulation occurs), blood lactate was and untrained subjects during rest- mote blood lactate removal is around removed faster than at lower intensi- ing recovery from a maximal exer- 30% to 40% of V̇O2 max (34). Higher ties (e.g., 40% to 60% of the LT) (92). cise bout (7, 42). Higher blood lactate levels mean more O2 is oxygen consumption. Further, see A Closer Look 4.1 required for lactate conversion to glucose in for more details on removal of lactate following gluconeogenesis exercise. Epinephrine and norepinephrine levels are much higher. IN SUMMARY All these factors may contribute to the EPOC be- The oxygen debt (also called excess post- ing greater following high-intensity exercise than exercise oxygen consumption [EPOC]) is the O2 following moderate-intensity exercise. This obser- consumption above rest following exercise. vation has been used to make claims that high in- Several factors contribute to the EPOC. First, tensity exercise is better for weight loss because some of the O2 consumed early in the recovery of the prolonged “after-burn.” However, it isn’t as period is used to resynthesize stored PC in the important for weight loss as people might hope. It muscle and replace O2 stores in both muscle has been shown that when subjects are tested at and blood. Other factors that contribute to the the same absolute work rate following an endur- “slow” portion of the EPOC include an elevated ance training program, the majority of these factors body temperature, O2 required to convert lactate are reduced in magnitude, and with it, the EPOC to glucose (gluconeogenesis), and elevated blood (110). Figure 4.5 contains a summary of factors levels of epinephrine and norepinephrine. thought to contribute to the excess post-exercise 74 Section One Physiology of Exercise Factors Contributing to Excess metabolism, with glycolysis producing most of the ATP. Post-Exercise Oxygen Consumption In general, the ATP-PC system can supply almost all the needed ATP for work for events lasting one to five Oxygen debt or excess seconds; intense exercise lasting longer than five sec- post-exercise onds begins to utilize the ATP-producing capability of oxygen glycolysis. It should be emphasized that the transition consumption from the ATP-PC system to an increased dependence upon glycolysis during exercise is not an abrupt change Resynthesis but rather a gradual shift from one pathway to another. Elevated of PC Events lasting longer than 45 seconds use a hormones in muscle combination of all three energy systems (i.e., ATP- PC, glycolysis, and aerobic systems). This point was Post-exercise emphasized in Figure 3.23 in Chap. 3. In general, in- Lactate elevation of conversion HR and tense exercise lasting approximately 60 seconds uti- to glucose breathing lizes 70%/30% (anaerobic/aerobic) energy production, whereas competitive events lasting 2 to 3 minutes Restoration of utilize anaerobic and aerobic bioenergetic path- Elevated muscle and body ways almost equally to supply the needed ATP (see blood oxygen temperature Fig. 3.23). Clearly, the contribution of aerobic energy stores production to the total energy requirement increases with the duration of the competition. Figure 4.5 A summary of factors that might contribute to excess post-exercise oxygen consumption (EPOC). See text for details. IN SUMMARY During high-intensity, short-term exercise (i.e., 2 to 20 seconds), the muscle’s ATP production METABOLIC RESPONSES TO is dominated by the ATP-PC system. EXERCISE: INFLUENCE OF Intense exercise lasting more than 20 seconds relies more on anaerobic glycolysis to produce DURATION AND INTENSITY much of the needed ATP. Finally, high-intensity events lasting longer The point was made in Chap. 3 that short-term, high- than 45 seconds use a combination of the intensity exercise lasting less than ten seconds utilizes ATP-PC system, glycolysis, and the aerobic primarily anaerobic metabolic pathways to produce system to produce the needed ATP for muscular ATP. In contrast, an event like the marathon makes contraction, with a 50%/50% (anaerobic/ primary use of aerobic ATP production to provide the aerobic) contribution needed for exercise needed ATP for work. However, events lasting longer lasting between two and three minutes. than 10 to 20 seconds and less than 10 minutes gener- ally produce the needed ATP for muscular contraction via a combination of both anaerobic and aerobic path- Prolonged Exercise ways. In fact, most sports use a combination of anaero- bic and aerobic pathways to produce the ATP needed The energy to perform long-term exercise (i.e., more for muscular contraction. The next three sections con- than ten minutes) comes primarily from aerobic me- sider which bioenergetic pathways are involved in en- tabolism. A steady-state oxygen uptake can generally ergy production in specific types of exercise. be maintained during submaximal, moderate-intensity exercise. However, two exceptions to this rule exist. First, prolonged exercise in a hot/humid environment Short-Term, Intense Exercise results in a “drift” upward of oxygen uptake; therefore, The energy to perform short-term exercise of high in- a steady state is not maintained in this type of exer- tensity comes primarily from anaerobic metabolic path- cise even though the work rate is constant (101) (see ways. Whether the ATP production is dominated by Fig. 4.6(a)). Second, continuous exercise at a high rela- the ATP-PC system or glycolysis depends primarily on tive work rate (i.e., >75% V̇O2 max) results in a slow the length of the activity (1, 4, 73, 80). For example, rise in oxygen uptake across time (57) (Fig. 4.6(b)). In the energy to run a 50-meter dash or to complete a each of these two types of exercise, the drift upward single play in a football game comes principally from in V̇O2 is due principally to the effects of increasing the ATP-PC system. In contrast, the energy to complete body temperature and to rising blood levels of the hor- the 400-meter dash (i.e., 55 seconds) comes from a com- mones epinephrine and norepinephrine (19, 46, 48, bination of the ATP-PC system, glycolysis, and aerobic 57, 78). Both of these variables tend to increase the Chapter Four Exercise Metabolism 75 (a) Incremental Exercise 2.4 Incremental exercise tests (also called graded e xercise tests) are often employed by physicians to ˙ 2 (L · min−1) 2 examine patients for possible heart disease and by exercise scientists to determine a subject’s cardiovas- cular fitness. These tests are usually conducted on VO 1.6 a treadmill or a cycle ergometer. However, an arm crank ergometer can be employed for testing indi- viduals who have lost use of their legs or athletes 1.2 10 20 30 40 50 whose sport involves arm work (e.g., swimmers). The Time (min) test generally begins with the subject performing a (b) brief warm-up, followed by an increase in the work 3.6 rate every one to three minutes until the subject can- not maintain the desired power output. This increase 3.2 in work rate can be achieved on the treadmill by ˙ 2 (L · min−1) increasing either the speed of the treadmill or the in- cline. On the cycle or arm ergometer, the increase in 2.8 power output is obtained by increasing the resistance VO against the flywheel. 2.4 Maximal Oxygen Uptake The maximal capacity to transport and utilize oxygen during exercise (maximal 2 0 5 10 15 20 25 oxygen uptake, or V̇O2 max) is considered by many Time (min) exercise scientists to be the most valid measurement of cardiovascular fitness. Figure 4.6 Comparison of oxygen uptake (V̇O2) across Figure 4.7 illustrates the change in oxygen up- time during prolonged exercise in a hot and humid en- vironment (a) and during prolonged exercise at a high take during a typical incremental exercise test on a relative work rate (>75% V̇O2 max) (b). Note that in cycle ergometer. Oxygen uptake increases systemati- both conditions there is a steady “drift” upward in V̇O2. cally with work rate until V̇O2 max is reached. When See text for details. V̇O2 max is reached, an increase in power output does not result in an increase in oxygen uptake; thus, V̇O2 metabolic rate, resulting in increased oxygen uptake max represents a “physiological ceiling” for the abil- across time. Use of a drug that blocks the receptors ity of the oxygen transport system to deliver O2 to that epinephrine and norepinephrine bind to (to block contracting muscles. In the classic presentation of this their effects) results in an elimination of the drift in test, the V̇O2 levels off or exhibits a “plateau” when V̇O2, confirming the link between the two (78). In the subject completes one stage further in the test contrast, even though an eight-week endurance train- (66, 117); Figure 4.7 shows this as a dashed line. The ing program was shown to reduce the drift in V̇O2 reason for using a dashed line is that many subjects during heavy exercise, it was not linked to a lower body temperature (21). Could lower epinephrine and 4.0 ˙ 2 max VO norepinephrine levels be the cause, as was shown for the lower EPOC following endurance training (110)? Clearly, additional research is needed to explain the 3.0 ˙ 2 ( L · min−1) causes of this drift in V̇O2 in heavy exercise. IN SUMMARY 2.0 VO The energy to perform prolonged exercise (i.e., more than 10 minutes) comes primarily from 1.0 aerobic metabolism. A steady-state oxygen uptake can generally be maintained during prolonged, moderate-intensity 50 100 150 200 250 300 exercise. However, exercise in a hot/humid Work rate (watts) environment or exercise at a high relative work rate results in an upward “drift” in oxygen con- Figure 4.7 Changes in oxygen uptake (V̇O2) during an sumption over time; therefore, a steady state is incremental exercise test. The dashed line indicates a plateau in V̇O2 at a higher work rate; however, this is not obtained in these types of exercise. not observed in many cases. 76 Section One Physiology of Exercise do not demonstrate this plateau at the end of an clude the following: (a) the maximum ability of the incremental test; they simply cannot do one more cardiorespiratory system to deliver oxygen to the con- stage beyond the one at which the highest V̇O2 was tracting muscle and (b) the muscle’s ability to take up achieved. However, there are ways to demonstrate the oxygen and produce ATP aerobically. Both genet- that the highest value reached is V̇O2 max (3, 32, 71, ics and exercise training are known to influence V̇O2 72, 76, 106) (see A Closer Look 4.2 for more on this). max; this will be discussed in Chap. 13. Chapter 15 The physiological factors that influence V̇O2 max in- describes tests to measure or estimate V̇O2 max. A CLOSER LOOK 4.2 V̇O2 max and Its Verification The concept of V̇O2 max and its de- having the subject do a single-stage test. However, the authors expressed pendence on cardiac output was de- follow-up test at a work rate higher appropriate concern that without scribed by Hill and Lupton in 1923 than the last one just completed on looking at individual data, that is, (66). Over the following decades, a maximal incremental exercise test. how much of a difference existed be- investigators had to deal with a cen- In this sense, a verification of the V̇O2 tween the first and follow-up test for tral issue in the measurement of V̇O2 max value would indicate that a “pla- each person, the average values could max: How can one be certain that a teau” had been achieved. The follow- disguise inherent within-subject vari- true V̇O2 max had actually been ob- ing studies provide some insights into ability. In effect, they are asking, what tained? The concept of a plateau in current approaches to this issue. In a is the largest difference in V̇O2 max oxygen uptake with increasing inten- study by Foster et al. (44), the tread- between the tests that you would sities of exercise (the “primary” crite- mill grade was set at 3% for female run- accept as signifying no difference in rion) was central to Hill and Lupton’s ners and at 4% for male runners, with response? This is similar to the issue description of V̇O2 max. Taylor et al. the initial speed set at 134 m · min−1 Taylor et al. (117) dealt with in es- (117) operationally defined the pla- (5 mph). The speed was increased by tablishing their criterion V̇O2 differ- teau for their test procedures (7 mph, 27 m · min−1 (1 mph) at 3-min in- ence of 2.1 ml · kg−1 · min−1 for one 2.5% grade change per stage) as a V̇O2 tervals until exhaustion. Following additional stage of their exercise test increase of