Powder Characterization: Methods and Techniques (PDF)
Document Details
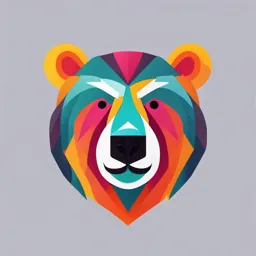
Uploaded by EffectiveTriumph8618
Johannes Kepler Universität Linz
Tags
Summary
This document provides an overview of powder characterization techniques, focusing on methods for measuring particle size. It discusses direct dimensional measurement, transport measurement, and rapid physical response measurement. The document would likely be useful for undergraduate students or researchers in material science or related fields.
Full Transcript
"A powder shall consist of discrete particles of any material with a maximum dimension of less than 1000 μm (= 1 mm)" An irregularly shaped particle has no unique dimension, and its size should be expressed in terms of the equivalent spherical diameter. A diagram of a project Description automati...
"A powder shall consist of discrete particles of any material with a maximum dimension of less than 1000 μm (= 1 mm)" An irregularly shaped particle has no unique dimension, and its size should be expressed in terms of the equivalent spherical diameter. A diagram of a project Description automatically generated Measurement methods for particle size Direct dimensional measurement ------------------------------ Sieving 10--25 000 μm Applicable for free-flowing dry or slurries wet powders Shaking the powdered sample through a series of wires of mesh or aperture arranged in decreasing aperture size Static microscopy direct observation (visual and/or electronic) of irregularly shaped particles Dynamic microscopy Moving particles-dynamic image analysis A static pictures of moving particles for a short duration of time period of illumination is determined by the magnification of the image desired from the image capture instrumentation and the resolution required moving particles observed in 3 systems - sheath flow- a sheath of liquid would control particle position directs the dispersed particle precisely to the focal point of the image capture instrument =\> a still image is then captured by short illumination periods of the flowing particles - electrical sensing zone ESZ- the image-capture equipment is focused on the orifice in the ESZ tube =\> as one particle passes through the ESZ orifice, an electrical pulse is generated which triggers a signal for the illumination of that particle, by a strobe flash light, at the precise instant the particle passes the - focal point of the image capture instrument the free-falling method-particles falling from a vibrating feeder are illuminated, and a still image is captured at the focal point. From image analyzers, we can deduct the area equivalent diameter, the ratio between martin's diameter and ferret's diameter, or from the projected area of each particle in pixels, the perimeter of the particle (p), the longest dimension, the shortest dimension, and the maximum and minimum Feret diameter. Transport measurement --------------------- Gravity- particle-size range of 10--1000 μm centrifugal and ultracentrifugation- 0.05--25 μm, smaller in sizes but reduces the time involved in the sedimentation of particles.  h- measure the terminal velocity or height of fall t- given time ρs- density of particles ρL- density of dispersed liquid μ- the liquid viscosity it is used to determine the particle size. Rapid physical response measurement ----------------------------------- 0.1--1000 μm electrical sensing zone, light scattering (optical and laser), light diffraction, photon correlation spectroscopy (dynamic light scattering), light blockage, ultrasonics. For example, in an electrical sensing zone, - 0.4 - 1200 µm The basic function is a change in electrical resistance occurring when a particle in an ionic solution passes through an aperture between two electrodes (the technology revolutionized medical research and diagnostics by eliminating the labour-intensive and often inaccurate method of manually counting blood cells). The conductivity changes when a particle passes through this orifice \~ to the volume of both a spherical and an irregularly shaped particle \- the magnitude of signal generated is evaluated from a calibrated instrument to size the particles to count the number of particle(s) passing through the orifice is also counted =\> size, number and volume, distribution. A diagram of a mercury manometer Description automatically generated In diffraction and light-scattering instruments, the intensity of light scattered by the particles, usually in suspension, is recorded as a function of the scattered angle  Resonator - optical cavity Gain medium - active medium absorbs "pump" energy (100% and 99% reflectivity mirror) creating population inversion a population inversion requires a minimum of 3 levels! with only two levels, the probabilities for adsorption and spontaneous emission are exactly the same Einstein coefficients A and B directly describe the spontaneous emission and stimulated emission probabilities, respectively A mathematical equation with numbers and symbols Description automatically generated Hellium is pumped up to excited state by electrical discharge, followed by energy transfer with collision to neon into metastable state. Then the stimulated emission is released. The sontaneous emission deplete the lower level to maintain the population inversion of neon.  Laser radiation has to be - Coherent -all laser photons have the same phase - Monochromatic- all emttied photons oscillate with same frequency. - Collimated- due opital resonator, the laser beam is parallel with a small dispersion. equilibrium radiation energy density per unit volume and per unit frequency - electron transitions - visible and ultraviolet - molecular vibrational transitions - infrared - molecular rotational transitions -- microwave photon correlation spectroscopy (dynamic light scattering - DLS) \- particle size for light scattering and light diffraction methods - generally, in the range: 0.05 to 3500 μm \- DLS - measurement of the time-dependent fluctuations of the intensity of light scattered by particles or molecules in a dispersed system illuminated by a coherent (laser) light source \- time-dependent scattered light fluctuations arise from the Brownian motion, and possibly other forces, of the dispersed particles, colloids or macromolecules \- scattered waves can interfere constructively or destructively depending on the distance traveled to the detector \- the decay time of the fluctuations - related to the diffusion constants =\> to the size of the particles \- small particles moving rapidly cause faster decaying fluctuations than larger particles moving slowly \- a coherent monochromatic laser light beam illuminates a sample of dispersed particles in a liquid \- scattered light intensity is measured by a photodetector placed at an angle (usually at 90°) to the light source A diagram of a scientific experiment Description automatically generated Surface area and porosity ------------------------- Before measuring surface area and porosity, CONSIDER Physisorption processes (adsorption) are due to weak electrostatic or dipole interactions between gas molecules and surface atoms (van der Waals and London forces) Chemisorption processes are due to formation of chemical bonds between gas molecules and surface atoms which involves sharing of the electrons in new molecular orbitals. Also consider incorporation of gas molecules with substrate resulting in substrate sputtering. Langmuir isothermal model describes the surface interactions, - the surface of the substrate (adsorbent) is uniform, thus all adsorption sites are equivalent - adsorbed molecules does not interact with each other - all adsorptions occur through the same mechanism - at the maximum adsorption rate, only one monolayer is formed  A white rectangular sign with black text Description automatically generated  Multilayer absorption is described by BET(Brunauer-Emmett-Teller) isotherm: A black and white math equation Description automatically generated with medium confidence - V - total volume of adsorbed gas - VM - gas volume in the 1st monolayer - (monolayer saturation) - xB = P / Psat , gas pressure and saturation vapor pressure at given T - cB = K1 / Km , equilibrium constants for 1st layer and multilayer adsorption  A black text with a white background Description automatically generated surface area - important to the characterization of irregular particles by particle size and shape \- the ease of agglomeration and compaction, together with many other powder technological processes, can vary widely with materials which have chemically identical structures but which may possess a differing physical nature \- whenever a solid is in contact with a fluid, the interface between the two phases is dependent upon the surface area available to interact with the surrounding fluid, be it gaseous or liquid \- the internal surface areas of size-enlarged particles or powders (\>1.0 m2/g) are generally determined from the monolayer or multilayer adsorption region of a low-temperature nitrogen adsorption isotherm using the Brunauer, Emmett and Teller (BET) equation =\> can also be used to evaluate the size and shape of pores within particles or the voids between particles (not \> 500 nm) permeametry - achieved by the passage of gases slightly above atmospheric pressure through a packed bed of powder, evaluates the external area of particles porosity - the material matrix within an agglomerated or compacted mass of powder may have pores or voids which are generally in the size range 1.0 nm to 0.1 mm \- these pore/void spaces can be sized by the physical adsorption of various vapours or gases at low (nitrogen, krypton and carbon dioxide) and ambient (water, butane or organic vapours).  Mechanical testing of elements Density Measurement ------------------- - **Pycnometer**: Measures density using fluid displacement. measures the density of solid and fluid. - A white background with black text Description automatically generated - **Areometer/Densimeter**: Measures relative density using graduated cylinder to probe the acidity and anti-freezing agents, and to measure the sugar content in grape and wine. - **Westphal's (Mohr's)Balance**: Uses hydrostatic weighing with an unequal-arm balance to measure the density of lquids and solids. Hardness Testing ---------------- 1 N = 1 kg m / s² normal gravitational acceleration g = 9,80665 m / s² 1 kp = 9.80665 N ( 1 kg x 9,80665 m / s² ) 1 N = 0.102 kp Mohr hardness scale  **(a) Brinell Hardness (HBW)** - Uses a **steel/tungsten carbide ball** pressed into the material. - Suitable for **coarse materials**, but not thin samples. Especially Tungsten carbide with 1, 2, 2.5, 5, 10 mm diameter. - A math formula with text Description automatically generated with medium confidence  Advantages Disadvantages **(b) Vickers Hardness (HV)** - Uses a **diamond pyramid** with 136^o^ for precise measurement. - Works for **very small/thin samples**, but requires careful preparation. - Advantages - Disadvantages **(c) Knoop Hardness (HK)** - Uses a **diamond rhombus** with angles of 172,5°, 130°for **microhardness** testing. - Works for coatings and thin materials. - Advantages - appropriate for using in harsh working places - Disadvantages - very long times required for the sample preparation and exact measurements **(d) Rockwell Hardness (HRC)** - Uses a **diamond cone with 120^o^ or steel ball** with applied force. - Fast, simple, but affected by surface imperfections. - the testing is easily conducted - possibility of failure through displacement during the test load - the quality of the diamond indenter has a great influence on the test Strength and Elasticity ----------------------- **Tensile Testing**: Measures force required to stretch a material. 3 types of deformation and fractures- double-notched tension, three-point bend, and compact tension. **Stress-Strain Curve**: Shows **elasticity (reversible deformation) vs. plasticity (permanent deformation)**. A close-up of a text Description automatically generated In a [ductile] specimen (metal or polymer), the breaking strength is defined as the stress imposed for an uniform change in the cross sectional area (Einschnürung). In a [brittle] material, the breaking strength is simply the stress at the breaking point.  A close-up of a white background Description automatically generated  A table with numbers and text Description automatically generated  At the beginning of the experiment, the stress changes linearly with the strain. In this range, any deformation is considered elastic because the sample returns to its original shape after the removal of the stress. At higher loads, the stress-strain curve is not linear. It describes a plastic deformation, that is, a permanent deformation of the material that does not regress after relieving the material. The stress at which a permanent deformation sets in is called the elastic limit (or proportional limit). However, a 0.2% deviation from this is usually considered to be the onset of plastic deformation.  Example: Breakaway of dislocations from the solute carbon atoms (for low carbon steels). If the physical appearance of the tensile specimen is considered, localized distortions, called Lüders fronts (local regions of yielded material), will traverse the length of the specimen during yield-point elongation, and continuous plastic flow under an increasing load will not commence until the entire gage section has yielded. The extent of the yield-point elongation will depend on the density of mobile dislocations (i.e., those that are not "locked") and the ease with which these dislocations can move once initiated. **Ductility**: Ability to stretch without breaking. For ductile metals, the tensile strength should be regarded as a measure of the maximum load that a metal can withstand under the very restrictive conditions of uniaxial loading.Typical tension stress-strain curve for ductile metal indicating yielding criteria. Point A, elastic limit; point A′, proportional limits; point B, yield strength or offset (0 to C) yield strength; 0, intersection of the stress-strain curve with the strain axis: Proportional limit (A′) - the highest stress at which stress is directly proportional to strain. It is obtained by observing the deviation from the straight-line portion of the stress-strain curve. The yield strength (B) - the stress required to produce a small specified amount of plastic deformation. The usual definition of this property is the offset yield strength determined by the stress corresponding to the intersection of the stress-strain curve offset by a specified strain. Offset yield strength determination requires a specimen that has been loaded to its 0.2% offset yield strength and unloaded so that it is 0.2% longer than before the test. **Fracture Toughness**: Resistance to crack propagation.  The stress-strain curves for high and low-toughness materials. The high-carbon spring steel has a higher yield strength and tensile strength than the medium-carbon structural steel. The structural steel is more ductile and has a greater total elongation. The total area under the stress-strain curve is greater for the structural steel; therefore, it is a tougher material. This illustrates that toughness is a parameter thatcomprises both strength and ductility. Comparison of stress-strain curves for high- and low-toughness steels. Cross hatched regions in this curve represent the modulus of resilience (UR ) of the two materials. The UR is determined by measuring the area under the stress-strain curve up to the elastic limit of the material. Point A represents the elastic limit of the spring steel; point B represents that of the structural steel. Material-Specific Tests ----------------------- **Metals**: Measured using tensile tests, yield strength, and elongation. **Polymers**: Show different stress-strain behaviors (e.g., plastics, fiber, and elastomers). A diagram of a diagram Description automatically generated  A graph of stress-strain curves Description automatically generated **Ceramics**: Tested via **flexural (bending) strength** or **uniaxial compression**. The most common is flexure testing, in either the so-called three-point or four-point configuration. The latter is usually further specified by a description of the distance from the outer support points and the inner points, such as or four-point loading. The high compressive strength of ceramics is a consequence of the resistance of the material to plastic flow and the insensitivity of defects to compressive stress. Compression strength is extremely difficult to accurately measure, because slight misalignments can create bending stresses, and end loading effects can cause parasitic tensile stresses that cause fracture. Mismatches of the elastic properties of the platens and test specimen can cause tensile stresses or frictional constraints. Buckling can occur if specimens are too long. Because the stresses being applied to a compression specimen is extremely high, the alignment errors may be greater than they would be for an equivalent tensile test specimen. Nanomechanical Testing ---------------------- - **Nanoindentation**: Uses atomic force microscopy (AFM) to measure small-scale hardness. Thermal analysis Radiation and elementary particles ### 1. Fundamental Forces and Nuclear Scale There are **four fundamental forces** that govern nuclear interactions: - **Gravitational** (weakest, acts on mass). - **Electromagnetic** (acts on charged particles). - **Strong nuclear** (binds protons and neutrons). - **Weak nuclear** (responsible for radioactive decay). ### 2. Radioactivity - Discovered by **Henri Becquerel (1896)**. - Results from **unstable atomic nuclei** emitting radiation to become stable. - **E. Rutherford** classified radiation into **alpha (α), beta (β), and gamma (γ) rays**. - **Other radioactive decays - electron capture, positron, internal conversion** #### Radioactive Half-Life - Time required for half of a radioactive sample to decay. - **Unaffected by external conditions** (temperature, pressure, chemical state). - It can only change via **high-energy nuclear interactions** with other particles. - A close up of black text Description automatically generated ### 3. Types of Radiation #### (a) Alpha (α) Radiation - **2 protons + 2 neutrons** (helium nucleus). Nuclear binding energy is -28.3MeV - **Low penetration** (stopped by paper or skin). - **High ionization (destructive) power** (dangerous if inhaled or ingested). - **Example decay:** #### (b) Beta (β) Radiation - **Fast-moving electrons (β⁻) or positrons (β⁺).** - **Higher penetration** than alpha, but stopped by plastic or aluminum. - **Beta-minus (β⁻) decay:** the emission of a electron and an electron antineutrino which shares the momentum and energy of the decay - **Beta-plus (β⁺) decay (positron emission):** -  #### (c) Gamma (γ) Radiation - **High-energy photons (electromagnetic waves).** - extremely high frequency (1020 Hz), therefore high energy photons (Me - **Highly penetrating** (requires lead shielding). - **No change in atomic number or mass.** - Used in **medical imaging, gamma spectroscopy, and metrology such as converting a star to a black hole.** \- emitters (medical): ^67^Ga, ^111^In, ^123^I, ^123m^Xe, ^123^Cs, ^201^Pb - \- others (spectroscopy): ^60^Co, ^137^Cs, ^241^Am ### 4. Other Nuclear Processes #### (a) Electron Capture - An atom **captures an inner electron(K shell)**, emitting a neutrino. p + e^-^−→n+ν - Competes with **positron emission** and produces **X-rays**. - In the middle range of the periodic table, those isotopes which are lighter than the most stable isotopes tend to decay by electron capture, and those heavier decay by negative beta decay. - Highly relevant for Mossbauer effect studies. - Nucleus is excited to its ground state by gamma emission. #### (b) Double Beta Decay - An extremely rare process where **two neutrons decay into two protons**, emitting **two electrons and two neutrinos**. - typical half-lives are larger than 10^20^ years - **Neutrinoless double beta decay** (if proven) would suggest that **neutrinos are their own antiparticles**. Cryogenic Underground Observatory for Rare Events (CUORE) #### (c) Internal Conversion - **An excited nucleus transfers energy to an orbital electron**, ejecting it without gamma emission. #### (d) Electron-Positron Annihilation - **A positron (e⁺) collides with an electron (e⁻), producing two gamma photons in opposite directions.** e^-^ + e^+^ −→ γ+γ E=511keV Conservation laws must be obeyed: \- electric charge before and after is 0 \- angular momentum \- linear momentum - basis for positron emission tomography (PET) and positron annihilation spectroscopy (PAS) - also used for measuring the Fermi surface and band structure in metals #### (e) Pair Production - **The opposite of annihilation:** a high-energy photon (gamma rays or x-rays) **creates an electron and a positron, resulting in a pair production.** γ→e++e− - Requires photon energy **\>1.022 MeV**. #### (f) Neutron Emission - Unstable nuclei **eject neutrons** without changing the atomic number, resulting in the same isotope. - Important in **nuclear fission and reactor control, which delays the emission of neutrons and slows down the nuclear reaction for better control.** - **Neuton radiation is** used for scattering and diffraction measurements - crystallography, condensed matter physics, etc - cold (0 - 0.025 eV), thermal (0. 025 eV), slow (1-10 eV), fast (1-50 MeV) - cold and thermal neutrons are used for diffraction, innelastic scattering, tomography - \- cancer treatment: fast neutron therapy (50-70 MeV) - slow neutrons capture therapy (1-10 eV ### 5. Radiation Detection Methods **Geiger Counter** -- Detects ionizing radiation by counting events.\ **Proportional Counter** -- Measures radiation energy.\ **Scintillation Detector** -- Converts radiation into light (luminescence).\ **Semiconductor Detector (Si, Ge, SiLi)** -- Converts radiation into electrical signals.\ **Film Badge Dosimeter** -- Personal exposure monitoring (photographic film). ### 6. Radiation Applications ✅ **Medical Imaging** -- PET scans, gamma-ray imaging.\ ✅ **Material Testing** -- X-ray and gamma-ray for detecting fractures.\ ✅ **Nuclear Power** -- Fission reactions for energy production.\ ✅ **Smoke Detectors** -- Use **alpha decay (Americium-241)** to ionize air.