Plasma Protein Systems.docx
Document Details
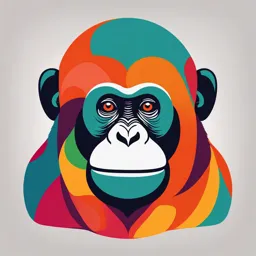
Uploaded by TransparentLemur
Brant Community Healthcare System
Full Transcript
Plasma Protein Systems ________________________________________ There are three interrelated plasma protein systems that normally exist in an inactive state. They include the complement system, the clotting system or coagulation cascade and the kinin system, each having its own end goal. These plasm...
Plasma Protein Systems ________________________________________ There are three interrelated plasma protein systems that normally exist in an inactive state. They include the complement system, the clotting system or coagulation cascade and the kinin system, each having its own end goal. These plasma derived mediators of inflammation are synthesized in the liver and include coagulation factors and complement proteins. Many of these protein components are enzymes that circulate in an inactive or ‘proenzyme’ state. Activation of a proenzyme by infection or injury creates a cascade type effect. Components of these plasma protein systems are usually short-live and are rapidly deactivated. Let’s look at each system separately. Complement: Components of the complement system include 20 different proteins, labelled C1 through C9, and make up about 10% of the total circulating proteins in the body. They are capable of direct destruction of pathogens or can activate and work with other components of the inflammatory response to affect the same results. As proenzymes are activated, a cascade forms that plays an important role in immunity and inflammation. Complement fragments contribute to the inflammatory response by causing vasodilation, increasing vascular permeability and enhancing activity of phagocytes. C3 and C5 activation is the most important piece of this cascade: activation of C3 and C5 results in formation of opsonins, chemotactic factors and anaphylatoxins. Recall that opsonins coat bacteria and tag them for destruction. The most potent component is C3b. Chemotactic factors draw other important inflammatory mediators to the site of injury as needed. Anaphylatoxins cause rapid degranulation of mast cells, increasing the inflammatory response. The most potent one is C3a. C5a has both chemotactic and anaphylatoxic properties. Other important components are C2b which causes vasodilation and increased permeability by smooth muscle relaxation and C4a which also acts as an anaphylatoxin inducing mast cell degranulation with release of histamine. The final endpoint of the complement cascade is formation of the membrane attack complex (MAC) which is composed of C5 through C9. Its job is to create holes in the membranes of pathogens, allowing entry of water, causing the cells to explode. As you can see from this diagram, there are three possible avenues through which the complement cascade can be activated: The Classical pathway is activated by antibodies and requires at least two Ag-Ab complexes to initiate the cascade. Thus, the immune system can take advantage of this option for destruction of bacteria. The Lectin Pathway is activated by bacterial carbohydrates and is similar to the classical pathway, but no antibodies are required. The Alternative Pathway is activated by gram negative bacterial and fungal cell wall polysaccharides (termed endotoxins); no antibodies are required; it begins with activation of C3b and merges with the classical pathway at C5. In summary, the complement cascade is activated in three ways and has 4 major effects: Opsonization Mast cell degranulation through anaphylatoxic activity Leukocyte chemotaxis Cell lysis Clotting System: The clotting system is activated by substances released during tissue destruction and infection. As you can see, there are two pathways (intrinsic and extrinsic) that converge at the place where factor X becomes Xa. Factor xa and thrombin both act to provide the link between the coagulation system and inflammation. Inflammation is enhanced by many of the by-products or fragments that are produced during the clotting cascade. For example, during fibrin production, fibrinogen releases fibrinopeptides that are chemotactic for neutrophils, cause increase vascular permeability and enhance the effect of bradykinin from the kinin cascade. Similarly, plasmin works with the complement cascade to activate C3a and C5a causing the release of histamine, enhancing the inflammatory response. And factor XIIa is also called ‘prekallekrein activator’, an enzyme that activates prekallekrein in the kinin cascade. So, you can clearly see the interdependent relationship between the three plasma protein systems. Stimulation of one cascade leads to stimulation of some of the components of the others, designed to enhance the overall inflammatory response. The primary goal of the clotting system then, is to produce a fibrous clot. The fibrinous network that forms serves to prevent the spread of infection by ‘trapping’ the offending agent and retaining it at the site of inflammatory activity, to prevent bleeding and to provide the framework for eventual healing and repair. Kinin System: The kinin system is the third plasma protein system involved in the inflammatory response and interacts closely with the clotting system. Both the clotting system and the kinin system are initiated by activated factor XII (factor XIIa). In this cascade, vasoactive peptides are generated from plasma proteins called ‘kininogens’ by the action of proteases called kallekreins. Kallekreins can be found in tissue and body fluids including saliva, tears, sweat, urine, feces, etc. The end result is production of bradykinin which causes vasodilation, increases vascular permeability, causes smooth muscle contraction, enhances leukocyte chemotaxis and stimulates pain receptors. Effects of bradykinin are very similar to histamine, although the effects are much shorter in duration as kinins are eventually degraded by kininases, to maintain homeostasis. Bradykinin appears to be of greater importance during the latter phases of inflammation. Emigration of Leukocytes ________________________________________ In this slide, you can click on each term to view the definition. Initially, as leukocytes are ‘recruited’ to the site of injury they move along the endothelial wall and are facilitated to linger there, by the slowing of blood flow. They then line up and move ‘adhere’ to the endothelium with the help of those adhesion molecules we talked about earlier. Some of the adhesion molecules are ‘selectins’, integrins’ and members of the Ig superfamily (ICAM-1, ICAM-2 and VCAM-1) and are found on both the leukocyte and endothelial cell walls and are complementary, meaning they fit together like a lock and key. Then, through a process, called ‘chemotaxis’, leukocytes are encouraged to move into the tissue where the injury has occurred. Migration: Leukocytes move along the endothelial wall. Adhesion: Leukocytes stick to the endothelium with the help of adhesion molecules. Transmigration: Leukocytes cross the blood vessel wall into the tissues. Chemotaxis: A response involving cell orientation or cell movement that is either toward (positive chemotaxis) or away from (negative chemotaxis) a chemical stimulus. Permeability Changes ________________________________________ Examples of chemical mediators that bind to endothelial cells to cause retraction include histamine, bradykinin, leukotrienes, etc. Take a moment now to review the roles of these mediators. When mediators bind to receptors on the endothelial cells, the cells begin to retract, leaving wide gaps between them. As a result, proteins and molecules that were once too big to squeeze between the endothelial cells, can now leave the vessel quite freely, taking water along with them. Loss of water into the extravascular space leads to a decrease in osmotic pressure within the blood vessel and edema of the tissues. The individual will also experience discomfort and quite possibly, impaired function. Also, with a loss of fluid from the intravascular space, comes increased blood viscosity. The blood becomes thick and sluggish leading to a slower flow. Now, the leukocytes are much more able to stick to the endothelium and prepare to leave the area to travel to the site of injury. Cellular Phase of Inflammation Now let's discuss the cellular phase of inflammation. Several simultaneous events occur during the vascular and cellular phases of inflammation. As previously mentioned, the endothelial lining undergoes changes which results in wide gaps between cells, allowing larger molecules and fluid to leak out into the tissues. How this occurs is an interesting process, involving a series of simultaneous steps. Let’s first look at the process of leukocyte emigration. During normal conditions, cells float within the blood vessel continuously and the endothelium secretes nitric oxide, causing vasodilation which limits the sticking of cells to itself (again, the concept of homeostasis). During inflammation, as biochemical mediators are released from mast cells, activation of plasma proteins are released from dying cells and cause production of adhesion molecules on the surface of many cells involved in inflammation. These adhesion molecules cause certain cells (leukocytes and platelets) to become ‘sticky’ and they adhere to the endothelial wall. Overview of Inflammation Video ________________________________________ Inflammation is the protective reaction of vascularized tissue to local injury. Acute inflammation is the early almost immediate reaction of tissue to the injury and it is the first phase of wound healing. Acute inflammation can be triggered by cell or tissue damage or by the presence of dead cells or noxious agents such as bacteria. Acute inflammation typically occurs before the immune response becomes established and aims primarily at removing the injury causing agent and limiting the extent of tissue damage. Acute inflammation occurs in two overlapping stages: vascular and cellular. In the vascular stage arterioles and venules near the site of injury constrict briefly then dilate. Dilation promotes congestion while an accompanying increase in capillary permeability leads to the movement of fluid into the affected tissue, resulting in the five classic signs of inflammation: redness, swelling, heat, pain, and loss of function. As fluid leaves the capillaries, the blood remaining in circulation becomes more viscous, it flows more slowly and clotting occurs. The cellular stage of acute inflammation is initiated by the movement of phagocytic white blood cells or leukocytes into the area of the injury. The leukocytes begin to adhere to the vessel wall and then in a process called emigration, squeeze through the wall and move into the inflamed tissue. The leukocytes wander through the tissue guided by chemical signals in a process called chemotaxis. The cellular stage culminates in the leukocyte engulfing and degrading the bacteria and cellular debris in a process called phagocytosis. Products of phagocytosis, along with plasma and blood cells, form exudates which accumulate causing swelling and pain. Exudates are composed of serous fluid, red blood cells, fibrinogen or tissue debris, and white blood cell breakdown products. Concurrent with the events of the vascular and cellular stages chemical mediators released bio-active agents that act to mediate the inflammatory response. Mediators are derived from the cells or from plasma. One of the first mediators of an inflammatory response is the cell-derived mediator histamine, found in high concentrations in the mast cells of connective tissues adjacent to blood vessels, as well as in blood basophils and platelets. Histamine is released in response to a variety of stimuli and causes dilation and increased permeability of capillaries. Serotonin, another cell-derived mediators, performs similar actions. The three major plasma-derived mediators are present in the plasma in precursor forms that must be activated usually by a series of proteolytic enzymes. The kinins increase capillary permeability and stimulate pain receptors. The clotting system traps exudates, microorganisms, and foreign bodies. The compliment cascade causes vasodilation, promotes leukocyte chemotaxis, and augments phagocytosis. Acute inflammation comprises a variety of complex protective responses to injury, that together, lay the ground work for the next stages of the body’s recovery – immune response and tissue repair. Local Manifestations of Inflammation ________________________________________ Now that we have discussed acute inflammation in great detail, let’s see if you can identify the pathophysiological processes that cause each of these clinical manifestations of inflammation. Then, click on each item on the list to check your answer. Heat: Caused by vasodilation and increased movement of blood to site of injury Redness: Caused by vasodilation and increased movement of blood to site of injury Edema: Caused by vasodilation, increased movement of blood to site of injury, increased capillary permeability, leakage of proteins and cells out of the endothelial wall taking water with it; results in increased fluid in the tissues Pain: Increased fluid in tissues causes pain; as well prostaglandins act directly on nerve endings to cause pain Pus: As neutrophils die off, their contents become exudate (pus) Clot: Initiation of the clotting cascade results in formation of a thrombus; acts to sequester invaders in the area of injury Loss of Function: May be due to edema and pain Phagocytosis ________________________________________ Here is a diagram illustrating all of the steps involved in the activity of a phagocyte. In addition, you can see the formation of a phagosome when a microorganism is engulfed by the phagocyte. When phagocytes die, the lysosomal contents are released and these can be toxic to surrounding tissue, causing increased permeability, chemotaxis of monocytes, connective tissue breakdown and activation of the plasma protein systems. In order to avoid toxicity, alpha-1 antitrypsin (a plasma protein from the liver) is released and inhibits the destructive effects. Feel free to have a look at the video on the next slide which shows all of the steps of phagocytosis. neutrophil chemotaxis. Chemotaxis is a dynamic process of directed cell migration. When leukocytes leave the capillary, they move in response to a chemical gradient caused by chemokines, bacterial and cellular debris, and protein fragments derived from the complement system. Both immune and non-immune cells ensure that leukocytes move in the appropriate direction. Chemotactic factors, also called chemoattractants, cause directional movement of cells toward the site of injury. Examples of chemotactic factors include neutrophil chemotactic factor and eosinophil chemotactic factor, and these are both contained in mast cells. Leukocyte Activation and Phagocytosis ________________________________________ Some offending microbes are ‘tagged’ or ‘opsonized’ by a complement factor known as C3b and by various antibodies of the immune system. We’ll look at complement factors shortly... Opsonization enhances recognition and binding activity of the phagocytes, acting like a glue between the phagocyte and the target. Major phagocytes including monocytes, neutrophils and tissue macrophages are activated by injured tissue which triggers the leukocyte response for phagocytosis and cell killing. Neutrophils are then ‘activated’ to form pseudopods that will encircle the offending organism or debris. Then, the phagocyte will fuse with an intracellular lysosome to form a phagolysosome which will release its enzymes and oxygen radicals to attack and destroy the offending microbe. Phagocytosis Process Review ________________________________________ Here we have a list of all the steps involved in phagocytosis. Clicking on each term will allow you to view individual definitions. The process of phagocytosis begins with margination or the slowing and adherence of leukocytes, mainly neutrophils, to the endothelium. They move along the periphery of the blood vessels and accumulate in clusters. Their goal now is to find the target and phagocytose or destroy it by capture and digestion. The plasma membrane of the phagocyte extends into finger-like projections through the gaps between individual endothelial cells and leaves the blood vessel by digesting the basement membrane and squeezing between the cells. Now the phagocyte migrates or moves towards the target which has been opsonised by compliment C3b or antibodies that have tagged or marked the target cell to make it more recognizable to the phagocyte and to allow for enhanced adherence with the phagocyte. The phagocyte upon reaching the target uses the finger-like projection to surround and engulf the target. This engulfment creates a new vacuole containing the target called the phagosome. This phagosome fuses with a lysosome to form a phagolysosome. Finally, the enzymes contained within the phagolysosome works on destruction and digestion of the target. Once phaogcytes have destroyed the foreign material, they themselves die off and become exudate or pus. Phagocyte products exit via the lymphatics. Margination: Leukocytes slow migration, adhere tightly to the endothelium, and move along the periphery of the blood vessels where they accumulate. Pseudopod formation: A process in which the plasma membrane of the phagocyte extends in finger-like projections. Diapedesis: Movement of leukocytes through the widened junction between endothelial cells into the tissue. Migration: Phagocytes move toward the target. Opsonization: A process whereby adherence is enhanced between the phagocyte and the target cell. Engulfment: A process in which the phagocyte pseudopods surround and encircle the target, forming an intracellular phagocytic vacuole, or ‘phagosome’. Fusion with lysosome: A process whereby the phagosome merges with a lysosome to form a ‘phagolysosome’. Destruction: The target is destroyed; the ultimate goal of phagocytosis. Once phagocytes have destroyed the foreign material, they themselves die off and become exudate (pus). Phagocytic products exit via the lymphatics Cell Derived Mediators of Inflammation Inflammatory mediators are responsible for the wide variety of clinical manifestations seen with the inflammatory response. In the normal state, cell derived mediators are held within intracellular granules to be secreted at a time when the body is in need of protection from invaders or injury. We are also capable of synthesizing additional mediators based upon need. The major sources of these mediators include platelets, neutrophils, monocytes and macrophages and mast cells, endothelial cells, smooth muscle, fibroblasts and most epithelial cells. Several types of mediators exist, and they can act on one or several target cells and have very different effects on different types of cells. Most of these mediators are short-lived (think homeostasis) as they are inactivated by enzymes, scavenged or degraded. Let’s look at the properties and functions of each of these mediators in turn. As you click you click on each individual mediator from the list on this slide a text box will appear with highlights of that particular mediator’s function. Histamine: Histamine is present in preformed cells and is one of the first mediators to be released during acute inflammation. Histamine is found in many places, including well perfused connective tissue, where it is most prevalent, circulating platelets and basophils, and within mast cell granules where it is released in response to trauma and immune reactions involving IgE. Histamine binds to H1 receptors on endothelial cells, causing vasodilation which helps to increase blood flow to the microcirculation. It also contributes to increased vascular permeability by causing retraction of endothelial cells in capillaries and stimulates increased adherence of leukocytes to the endothelium. Histamine also has some negative consequences as it causes smooth muscle constriction of the bronchioles, making breathing difficult during an acute inflammatory reaction. Thankfully, antihistamine (H1 receptor antagonist) medications are capable of binding to the H1 receptor to compete with histamine to antagonize the many effects of the acute inflammatory response. Serotonin: Serotonin is very similar to histamine in that it is released mainly by mast cells, basophils and platelets and causes smooth muscle contraction, small blood vessel dilation and increased vascular permeability. Lysosomal enzymes: Are small membrane-enclosed sacs that contain very powerful enzymes. These lysozomes are capable of fusing with phagocytes for the purpose of destroying foreign invaders. Arachidonic metabolites: Are fatty acids found in phospholipids of the cell membrane. They are released from mast cells and initiate complex reactions that lead to the production of other inflammatory mediators (prostaglandins and leukotrienes) Prostaglandins: Are synthesized from arachidonic acid metabolites and serve to induce inflammation and enhance the effects of histamine and other inflammatory mediators. They promote vasodilation and bronchoconstriction and increase neutrophil chemotaxis, cause pain through direct action on nerves and fever. The use of ASA and NSAIDs counteract this effect by inhibiting prostaglandin synthesis. Thromboxane A2: Thromboxane A2 is produced primarily by platelets at the site of injury and promotes platelet aggregation, bronchoconstriction and vasoconstriction. Leukotrienes: Have a similar function to histamine and are synthesized while histamine is busy at work. They increase vascular permeability, induce smooth muscle contraction and constrict pulmonary airways, thus playing a major role in mediation of asthma and anaphylaxis. In addition, they affect the adhesion properties of endothelial cells and the extravasation and chemotaxis of neutrophils, eosinophils and monocytes. Leukotrienes, because of their ‘late start’, help to prolong the inflammatory response. Platelet Activating Factor: PAF is generated from lipids stored in the cell membrane and induce platelet aggregation. It also serves to activate neutrophils and potentially acts as a chemoattractant for eosinophils. Cytokines and chemokines: Are proteins that play a role in both acute and chronic inflammation and immunity. They are either pro-inflammatory or anti-inflammatory. Again, remember the concept of homeostasis. They are produced by many cell types, including activated macrophages and lymphocytes, endothelium, epithelium, and connective tissue and modulate the inflammatory response by many other cells by travelling and binding to and affecting the function of those target cells. There are literally hundreds of examples of cytokines and chemokines. A few examples of cytokines and their roles are shown on the diagram that you can enlarge by clicking on the magnifying glass. For more details on the many specific cytokines and chemokines, please refer to the attachment for this module entitled ‘Cytokines & Chemokines’ Nitric Oxide: NO is produced by the endothelial cells and causes smooth muscle relaxation and antagonizes platelet adhesion, aggregation and degranulation. It also plays a role in regulating recruitment of leukocytes. Blocking of NO production promotes leukocyte rolling and adhesion to capillary venules; delivery of NO reduces leukocyte recruitment; therefore, production of NO reduces the cellular phase of inflammation. Impaired production of NO is implicated in the inflammatory changes associated with atherosclerosis (more on this later) and also has some antimicrobial properties. Oxygen-free radicals: Are often released by leukocytes after exposure to microbes, cytokines and immune complexes or during the phagocytic process. Release of low levels increases expression of cytokines and endothelial adhesion molecules, enhancing the inflammatory process. At high levels, they can cause significant damage to the endothelium leading to increased permeability. Human Defense Mechanisms ________________________________________ Inflammation can be specific (and is also called the immune response) or non-specific (also termed the inflammatory response). Inflammation can be innate (meaning natural or present at birth) or acquired (evolving over time after birth). We are all born with the same innate abilities to protect ourselves, but each one of us will each vary in our ability to acquire immunity based on our individual experiences with exposure to pathogens and foreign antigens over our lifetime. We will all develop our own unique immune systems, tailored just for us. So, we have three types of defense mechanisms: Natural barriers (our first line of defense) include the epithelial layer of the skin and mucous membranes lining the gastrointestinal, genitourinary and respiratory tracts. These are called natural ‘physical’ barriers. These physical barriers can include mechanical and chemical types. Mechanical means of ridding the body of pathogens include sloughing, sneezing, coughing, vomiting, urinating and the cilial action of the respiratory tract. As well, low skin temperatures discourage the growth of bacteria. Chemical barriers include mucous, perspiration, saliva, tears, and earwax that trap and kill microorganisms. Some of these barriers contain enzymes, fatty and lactic acids and antimicrobial proteins that destroy bacteria. Similarly, our own normal bacterial flora are capable of producing chemicals to keep pathogens at bay. Inflammation (our second line of defense) is the primary focus of this module and will be discussed in more detail shortly. Immunity is considered our third line of defense and is an acquired, specific and adaptive ability. Over time, our immune system develops specific antibodies designed to target specific antigens and this defense mechanism has memory. This means that upon first exposure to an antigen, our immune system makes antibodies targeted toward that antigen. Then, upon subsequent exposure to that same antigen, those antibodies that ‘match’ the antigen will be called to action to fight off new infection. In your readings, you will also come across terms like ‘humoral’ and ‘cellular’ in reference to inflammation and immunity. These terms simply tell us where the inflammatory response originates. Humoral implies that the response comes from the blood or plasma components. Humoral response in inflammation involves complement factors while in immunity, it involves the formation and action of antibodies. The cellular response refers to a cell-derived process. In inflammation, the involved cells are neutrophils and macrophages and in the immune response, we are talking about lymphocytes. Goals of Inflammation ________________________________________ The goals of inflammation include: •Movement of all the necessary blood and cellular components to the site of injury or insult •Delivery of nutrients and blood cells to eradicate the offender •Dilution, confinement and elimination of the offending agents •Stimulation and facilitation of components of the immune system •Promotion of healing with generation of new tissue How are these goals accomplished? There are three major events that occur pretty much simultaneously and include: The first is an increased metabolic rate. When called upon to fight injury or infection, cells step up their usual daily routines and increase production of the necessary items for battle. As a result, we increase our heat production, our oxygen and glucose consumption and our production of wastes. Secondly, we have dilation of blood vessels to help speed up delivery of the inflammatory components to the site of injury. And finally, we have an increased capillary permeability which allows for movement of white cells, (specifically neutrophils) proteins and nutrients out of the blood vessels and into the tissue where they can go to work. Cells Involved in Inflammation ________________________________________ Before we examine the processes involved in inflammation, it would first be helpful to review the functions and roles of the cells involved in these processes. Normally, cells float within our blood vessels continuously and harmoniously. In response to inflammation though, each cell type springs into action to perform a very specific function. There are numerous biochemical mediators released from mast cells, plasma proteins and dying cells which trigger the production of ‘adhesion molecules’ on the surface of many cells. Adhesion molecules cause these cells to stick to, or ‘adhere’ to the endothelium. Let’s look at each of the cell types involved in the inflammatory response, beginning with the endothelium. Endothelial Cells: Endothelial cells line the walls of blood vessels and normally maintain very close contact with each other. The space between them is very tight, limiting the movement of cells and particles across the vessel wall. In addition to this ‘traffic control’ function, endothelial cells also: Produce antiplatelet and antithrombotic agents to prevent formation of clots Produce both vasoconstrictors and vasodilators to regulate flow Regulate leukocyte extravasation through the use of adhesion molecules Regulate immune cell proliferation through secretion of colony-stimulating factors Participate in the repair process through angiogenesis and formation of an extracellular matrix Platelets: These cells are also referred to as thrombocytes and they circulate passively until activated by products of tissue degradation like collagen, thrombin, and platelet activating factor. Their primary role is one of hemostasis or the stemming of blood flow. Once activated, they produce potent inflammatory mediators which result in increased vascular permeability, chemotaxis, adhesive and proteolytic properties of the endothelium Neutrophils: These cells represent one type of granulocyte (so named because enzyme-containing lysosomal granules are found within their cytoplasm) and are considered the chief phagocytic leukocytes. We’ll talk more about phagocytosis a little later. Early in the inflammatory response (about 90 minutes to 6-12 hours post injury), these cells are attracted to the site of injury by chemotactic factors. On their surface are found a number of different ‘receptors’ each designed to recognize and interact with certain substances such as bacterial glycoproteins, microbes, cytokines, chemokines. Because of their lysosomal enzymes, they are called upon to destroy invaders and remove subsequent debris. Once they themselves die off, they become exudate, or pus. In the presence of inflammation, neutrophils are released from bone marrow and the neutrophil count will rise. They are relatively short-lived because they are incapable of division, and when they die off, they release macrophage chemotactic factor to attract macrophages to the site of injury. In the presence of severe inflammation, as demand for neutrophils increases, the bone marrow can’t quite keep up and releases immature neutrophils called ‘bands’. When the ‘band’ count is elevated on a CBC, we then know that the bone marrow is overworked and trying valiantly to keep up with an on-going inflammatory process. Monocytes/Macrophages: Like the neutrophils, monocytes are also leukocytes derived from bone marrow, but contain larger and fewer lysosomes than their counterparts. They too, express receptors that interact with a variety of substances. Typically, monocytes exit the circulation in response to inflammation and take up residence in various tissues as the more mature macrophage. Monocytes then, are considered to be an immature form of macrophage. Macrophages are named according to their tissue location- some examples include Kupffer cells in the liver, alveolar macrophages in the lung, microglia in the brain to name a few. Macrophages arrive at the site of inflammation a little later than the neutrophils (about 24-48 hours post injury). Eventually, they replace the neutrophils as they die off. Macrophages are often associated with chronic inflammation as they are somewhat sluggish. Monocytes/macrophages differ somewhat from neutrophils in other ways too. They produce very potent vasoactive mediators (prostaglandins, leukotrienes, platelet activating factor, inflammatory cytokines and growth factor) and they engulf more material than neutrophils. Their lifespan is 3-4x longer than neutrophils and they also interact with the immune system. Macrophages are responsive to lymphokines from T cells which enhance their efficacy and work with the immune system by processing and presenting antigens to the lymphocytes and by stimulating growth and differentiation of granulocytes and monocytes in the bone marrow and substances that promote wound healing. Eosinophils: Eosinophils are granulocytes with many lysosomes. They contain biochemical mediators of inflammation and are especially prominent in the allergic response and hypersensitivity disorders. As well, they are particularly good at tackling parasitic infections. Like their counterparts, eosinophils circulate in the blood until they are needed to respond to insult or injury. Then, they migrate to the tissues where they modulate release of inflammatory mediators and degrade vasoactive molecules, controlling the vascular effects of histamine and serotonin. Basophils: Basophils are very similar in function to eosinophils. They too, produce lipid mediators and cytokines to induce the inflammatory response. They too, are important in the allergic and hypersensitivity reactions. They also interact with the immune system in that they bind to IgE through receptors on their cell surface. This action triggers the release of histamine and vasoactive agents. Mast Cells: In just a few moments, we will be discussing the role and function of mast cells in great detail. Suffice it to say, they are considered the most important activators of the inflammatory response, by performing two functions: degranulation and synthesis of mediators.