Plant Biotechnology: Historical Background & Micropropagation - PDF
Document Details
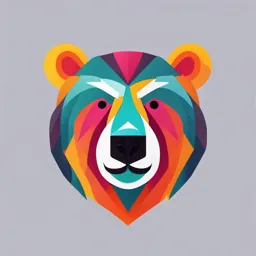
Uploaded by WarmheartedPun
Tags
Summary
This document provides a history of plant biotechnology, showing the evolution of techniques from ancient practices to modern innovations. It discusses key topics from the role of tissue culture media to the methods of micropropagation and related biological principles. You can also explore topics such as somatic embryogenesis and the commercial aspects of these technologies.
Full Transcript
Historical Background of Plant Biotechnology From Ancient Practices to Modern Innovations Role of Media in Tissue Culture Acts as a nutrient source. Supports cell growth and differentiation. Why Plant Biotechnology? Introduction What is Plant Biotechnology? coll...
Historical Background of Plant Biotechnology From Ancient Practices to Modern Innovations Role of Media in Tissue Culture Acts as a nutrient source. Supports cell growth and differentiation. Why Plant Biotechnology? Introduction What is Plant Biotechnology? collection of techniques, molecular tools that can be used to enhance the quality of the crop or better for the industrial use OR Plant biotechnology is the application of science and technology to modify plants for specific uses, including agriculture, industry, and research. Importance: Improves crop yield, disease resistance, and environmental sustainability. Ancient Biotechnology Early Agricultural Practices (10,000 BCE – 1700s) Domestication: Early humans selectively cultivated plants with desirable traits (e.g., wheat, maize, rice). Examples: o Domestication of wild wheat in Mesopotamia (~10,000 years ago). o Cultivation of maize from teosinte in Mesoamerica (~9,000 years ago). o Egyptians used yeast in wine and bread making (~4,000 years ago) o Chinese develops fermentation (~2,000 years ago) o New foods were developed accidently Classical Biotechnology 1700-1720: Thomas Fairchild, the forgotten father of the flower garden, creates Europe's first hybrid plant. Classical Biotechnology Dianthus barbatus Dianthus caryophyllus he was the first person to scientifically produce an artificial hybrid, Dianthus Caryophyllus barbatus, known as "Fairchild's Mule", a cross between a Sweet William (Dianthus barbatus) and a Carnation (Dianthus caryophyllus). Dianthus Caryophyllus barbatus Classical Biotechnology In 1838, while Schleiden and Schwann were enjoying after-dinner coffee, Schleiden talked excitedly about the universality of plant cells. All of the species that he had examined seemed to be made up of recognizable units or cells. Schwann was immediately struck by the similarity of Schleiden’s observations to his own studies of animals. After dinner they retreated to Schwann’s laboratory to look at his microscopic preparations Classical Biotechnology They published their results a year apart—Schleiden (1838), and Schwann (1839)—without any reference or acknowledgement of each other’s contribution (an unfortunate and unacceptable practice that is still a part of scientific publishing). Who will pay the bill ? Classical Biotechnology 1866: Austrian monk Gregor Mendel publishes important work on heredity Classical Biotechnology 1870-1890: Plant researchers cross-breed cotton to develop hundreds of new varieties with superior qualities. 1871-early 1900s: Luther Burbank develops the Russet Burbank Potato, and several hybrid fruits, including plums, berries, prunes and peaches. 1902: Gottlieb Haberlandt developed the concept of plant tissue culture. His original idea presented in 1902 was called totipotency Classical Biotechnology 1908: First U.S. hybrid maize produced by G.H. Shull of Carnegie Institute through self- pollination. PROPOSED HYBRIDISATION 1919: Word “biotechnology” coined by Hungarian engineer Karl Ereky. 1930: Inspired by writings of Luther Burbank, U.S. Congress passes the Plant Patent Act, enabling the products of plant breeding to be patented. Classical Biotechnology 1933: Hybrid maize becomes available commercially in the United States, causing maize yields to triple over the past 50 years. 1953: James Watson and Francis Crick describe the double helix structure of deoxyribonuleic acid (DNA), providing more insight into how DNA carries genetic information. 1960s: After decades of work, Norman Borlaug creates dwarf wheat that increases yields by 70 percent, launching the Green Revolution that helps save millions of lives. His grandfather once told him, "you're wiser to fill your head now if you want to fill your belly later on. Classical Biotechnology 1964, Guha and Maheshwari made discovery of the pollen culture technique, which is used to produce haploid plants In 1966, they developed a new high-speed culture technique for producing homozygous pure lines of haploid plants which is now in practice for crop improvement and for commercial production of horticultural and ornamental plants. Modern Biotechnology 1973: Stanley Cohen and Herbert Boyer were the first scientists to transplant genes from one living organism to another, a fundamental discovery for genetical engineering, launching the modern biotechnology era. Invented recombinant DNA (rDNA) technology by combining a frog gene with a bacterial plasmid in E-coli Modern biotechnology 1982: The first biotech plant is produced — a tobacco plant resistant to an antibiotic. 18th January 1983, one of the most important dates in the history of plant biotechnology at the Miami Winter Symposium, where Agrobacterium tumefaciens-mediated genetic transformation leading to the production of normal, fertile transgenic plants was presented. Modern biotechnology 1985: Field trials for biotech tobacco plants that are resistant to insects, viruses and bacteria are held in the United States. The first transgenic food crop to be commercialized was Flavr Savr, a delayed ripening tomato, in 1994 Modern biotechnology 1994: The first transgenic food crop to be commercialized was Flavr Savr, a delayed ripening tomato, 1995-96: Biotech soybeans and maize are approved for sale and biotech cotton is commercialized in the United States. Modern biotechnology 1999: German and Swiss scientists develop golden rice, fortified with betacarotene, which stimulates production of vitamin A that can prevent some forms of blindness. 2018: Canada and the United States approved golden rice, declaring it safe for consumption. 2019: It was approved for use as human food and animal feed or for processing in the Philippines. Modern biotechnology 2000: The first entire plant genome is sequenced, Arabadopsis thaliana A Small Plant's Genome Has Huge Impact Modern biotechnology Clustered regularly interspaced short palindromic repeats, CRISPRs Discovery of CRISPR and its function 1993 - 2005 — Francisco Mojica, University of Alicante, Spain Modern biotechnology 2005: Discovery of Cas9 and PAM — Alexander Bolotin, French National Institute for Agricultural Research (INRA) 2008: Spacer sequences are transcribed into guide RNAs— John van der Oost, University of Wageningen, Netherlands Modern biotechnology 2008: CRISPR acts on DNA targets Luciano Marraffini and Erik Sontheimer, Northwestern University, Illinois 2010: Cas9 cleaves target DNA Sylvain Moineau, University of Laval, Quebec City, Canada Modern biotechnology Moineau and colleagues demonstrated that CRISPR-Cas9 creates double-stranded breaks in target DNA at precise positions, 3 nucleotides upstream of the PAM. They also confirmed that Cas9 is the only protein required for cleavage in the CRISPR- Cas9 system. Modern biotechnology 2011: Discovery of tracrRNA for Cas9 system — Emmanuelle Charpentier, Umea University, Sweden and University of Vienna, Austria At about the same time, Doudna was mapping the cas proteins, a series of enzymes associated with CRISPR that This is the first time the snip apart DNA at specific spots. chemistry Nobel has gone to two women. Components of Plant Tissue Culture Media 1.Macro and Micronutrients: 1.Examples: Nitrogen, Phosphorus, Potassium, Calcium (macronutrients); Iron, Zinc, Manganese (micronutrients). 2.Vitamins: 1.Common ones: Thiamine (Vitamin B1), Nicotinic acid, Pyridoxine. 3.Carbon Source: 1.Usually sucrose or glucose. Components of Plant Tissue Culture Media 1.Macro and Micronutrients: 1.Examples: Nitrogen, Phosphorus, Potassium, Calcium (macronutrients); Iron, Zinc, Manganese (micronutrients). 2.Vitamins: 1.Common ones: Thiamine (Vitamin B1), Nicotinic acid, Pyridoxine. 3.Carbon Source: 1.Usually sucrose or glucose. 4.Plant Growth Regulators (PGRs): 1.Auxins, cytokinins, gibberellins, and their roles. 5.Gelling Agents: 1.Agar or alternatives for semi-solid media. 6.pH Adjustment: 1.Optimum range: 5.6–5.8. Plant cell culture media Three basic components: (1) essential elements, or mineral ions, supplied as a complex mixture of salts; (2) an organic supplement supplying vitamins and/or amino acids; and (3) a source of fixed carbon; usually supplied as the sugar sucrose. Plant cell culture media 1. Nitrogen Component of proteins, nucleic acids and some coenzymes 2. Potassium Regulates osmotic potential, principal inorganic cation 3. Calcium Cell wall synthesis, membrane function, cell signalling 4. Magnesium Enzyme cofactor, component of chlorophyll 5. Phosphorus Component of nucleic acids, energy transfer, component of intermediates in respiration and photosynthesis 6. Sulphur Component of some amino acids (methionine, cysteine) and some cofactors Plant cell culture media 7. Chlorine Required for photosynthesis 8. Iron Electron transfer as a component of cytochromes 9. Manganese Enzyme cofactor 10. Cobalt Component of some vitamins 11. Copper Enzyme cofactor, electron-transfer reactions 12. Zinc Enzyme cofactor, chlorophyll biosynthesis 13. Molybdenum Enzyme cofactor, component of nitrate reductase For practical purposes, the essential elements are further divided into the following categories: (1) macroelements (or macronutrients); (2) microelements (or micronutrients); and (3) an iron source. Macroelements Nitrogen, phosphorus, potassium, magnesium, calcium and sulphur (and carbon, which is added separately) are usually regarded as macroelements. These elements usually comprise at least 0.1% of the dry weight of plants. Concentration in medium (mg/l) NH4NO3 1650 KNO3 1900 CaCl2.2H20 440 MgS04.7H20 370 kh2po4 170 Macroelements Nitrogen must be in the reduced form to be incorporated into macromolecules. However, at high concentrations, ammonium ions can be toxic to plant cell cultures and uptake of ammonium ions from the medium causes acidification of the medium. Hence, Nitrogen is most commonly supplied as a mixture of nitrate ions (from the KNO3) and ammonium ions (from the NH4NO3). High concentrations of phosphate can lead to the precipitation of medium elements as insoluble phosphates. Microelements Manganese, iodine, copper, cobalt, boron, molybdenum, iron and zinc usually comprise the microelements. Concentration in medium (mg/l) KI 0.83 H3BO3 6.2 MnS04.4H20 22.3 ZnS04.7H20 8.6 Na2Mo04.2H20 0.25 CuS04.5H20 0.025 CoC12.6H20 0.025 Microelements Iron source Concentration in medium (mg/l) FeS04.7H20 27.8 Na2EDTA.2H20 37.3 Iron is usually added as iron sulphate and is usually used in conjunction Ethylenediaminetetraacetic acid (EDTA) to allow the slow and continuous release of iron into the medium. Uncomplexed iron can precipitate out of the medium as ferric oxide. Organic supplements Only two vitamins, thiamine (vitamin B1) and myoinositol (considered a B vitamin) are considered essential for the culture of plant cells in vitro. The most frequently used is glycine (arginine, asparagine, aspartic acid, alanine, glutamic acid, glutamine and proline are also used). Concentration in medium (mg/l) Myoinositol 100 Nicotinic acid 0.5 Pyridoxine-HCl 0.5 Thiamine-HCl 0.5 Glycine 2 Carbon source Sucrose is cheap, easily available, readily assimilated and relatively stable and is therefore the most commonly used carbon source. Other carbohydrates such as glucose, maltose, galactose and sorbitol) can also be used Concentration in medium (mg/l) Sucrose 30 000 Gelling agents For any culture types that require the plant cells or tissues to be grown on the surface of the medium, it must be solidified (more correctly termed ‘gelled’). Agar, produced from seaweed, is the most common type of gelling agent, and is ideal for routine applications. Plant growth regulators Plant growth regulators are the critical media components in determining the developmental pathway of the plant cells. The plant growth regulators used most commonly are plant hormones or their synthetic analogues. There are five main classes of plant growth regulator (1) auxins; (2) cytokinins; (3) gibberellins; (4) abscisic acid; (5) ethylene. Plant growth regulators Auxins Auxins promote both cell division and cell growth. The most important naturally occurring auxin is IAA (indole-3-acetic acid), but its use in plant cell culture media is limited because it is unstable to both heat and light. Amino acid conjugates of IAA (such as indole-acetyl-L-alanine and indole-acetyl-L-glycine), which are more stable. 2,4-Dichlorophenoxyacetic acid (2,4-D) is the most commonly used auxin and is extremely effective in most circumstances. Plant growth regulators Abbreviation/name Chemical name 2,4-D 2,4-dichlorophenoxyacetic acid 2,4,5-T 2,4,5-trichlorophenoxyacetic acid Dicamba 2-methoxy-3,6-dichlorobenzoic acid IAA Indole-3-acetic acid IBA Indole-3-butyric acid MCPA 2-methyl-4-chlorophenoxyacetic acid NAA 1-naphthylacetic acid NOA 2-naphthyloxyacetic acid Picloram 4-amino-2,5,6-trichloropicolinic acid Plant growth regulators Cytokinins promote cell division. Naturally occurring cytokinins are purine derivatives for example zeatin and 2iP (2-isopentyl adenine). They are expensive (particularly zeatin) and relatively unstable. The synthetic analogues, kinetin and BAP (benzylaminopurine), are therefore used more frequently. Plant growth regulators Abbreviation/name Chemical name BAP 6-benzylaminopurine 2iP (or IPA) N6-(2-isopentyl)adenine Kinetin 6-furfurylaminopurine Thidiazuron 1-phenyl-3-(1,2,3-thiadiazol-5-yl)urea Zeatin 4-hydroxy-3-methyl-trans-2-butenylaminopurine Plant growth regulators Gibberellins are involved in regulating cell elongation, and are agronomically important in determining plant height and fruit-set. GA3 being the most commonly used in plant tissue culture media Abscisic acid (ABA) inhibits cell division. It is most commonly used in plant tissue culture to promote distinct developmental pathways such as somatic embryogenesis Plant growth regulators The ratio of the auxin to the cytokinin determining the type of culture established or regenerated A high auxin to cytokinin ratio generally favours root formation, whereas a high cytokinin to auxin ratio favours shoot formation. An intermediate ratio favours callus production. Intermediate ratio high cytokinin to auxin ratio high auxin to cytokinin ratio Culture types An explant is a piece of tissue used to initiate a tissue culture. Generally, younger, more rapidly growing tissue (or tissue at an early stage of development) is most effective. Callus An unorganized, growing, and dividing mass of cells from explants. During callus formation there is some degree of dedifferentiation, both in morphology and metabolism. One major consequence of this dedifferentiation is that most plant cultures lose the ability to photosynthesise necessitates the addition of other components such as vitamins and carbon source. Callus Culture Callus culture is often performed in the dark as light can encourage differentiation of the callus.. During long-term culture, the culture may lose the requirement for auxin and/or cytokinin. This process, known as ‘habituation’. Callus cultures can also be used to initiate cell suspensions culture cell suspensions culture Callus cultures fall into one of two categories: compact or friable. In friable callus the cells are only loosely associated with each other and can breaks apart easily to provides the inoculum to form cell- suspension cultures. The friability can be improved by manipulating the medium components or by repeated subculturing. or by culturing it on ‘semi-solid’ medium cell suspensions culture A relatively large inoculum should be used when initiating cell suspensions so that the released cell numbers build up quickly. The inoculum should not be too large though, as toxic products released can build up to lethal levels. Cell suspensions can be maintained relatively simply as batch cultures by repeated subculturing into fresh medium to avoid ‘stationary phase’. Protoplast culture Protoplasts are plant cells with the cell wall removed. Protoplasts are most commonly isolated from either leaf mesophyll cells or cell suspensions. Removing the cell wall can be taken— mechanical or enzymatic isolation Mechanical isolation results in low yields and poor quality due to substances released from damaged cells Protoplast culture Enzymatic isolation is usually carried out in a simple salt solution with a high osmoticum, plus the cell wall degrading cellulase and pectinase enzymes Protoplasts are fragile and easily damaged, hence liquid medium is not agitated and a high osmotic potential is maintained. Protoplasts can be plated out on to solid medium and callus produced. Protoplasts are ideal targets for transformation. Single cell culture Single cell culture is a method of growing isolated single cell aseptically on a nutrient medium under controlled condition. Single cells from the intact plant tissue (leaf, stem, root cladode etc.) are isolated either mechanically or enzymatically by pectinase or macerozyme treatment The friable tissue in liquid medium is continuously agitated by a shaker to dispenses the single cells Single cell culture The five methods to culture single cell are: (1) The Paper Raft Nurse Technique (2) The Petri Dish Plating Technique (3) The Micro-chamber Technique (4) The Nurse Callus Technique and (5) The Micro-droplet Technique. Method # 1. The Paper Raft Nurse Technique: A sterile 8 mm x 8 mm squares of filter paper are placed aseptically on the upper surface of the actively growing callus tissue of the same or different species called nurse tissue. The isolated single cell is placed aseptically on the wet filter paper raft get nutrition from nurse tissue through diffusion. The single cell divides and re-divides and ultimately forms a small cell colony. Method # 2. The Petri Dish Plating Technique: A suspension of purely single cells is prepared aseptically from the stock cell suspension culture by filtering and centrifugation. The solid medium (1.6% ‘Difco’ agar added) is melted in water bath. Single cell suspension is mixed in cooled (35°C) agar liquid medium and mixture is poured in petridish and petridish placed in inverted position. The dividing cells ultimately form pin-head shaped cell colonies within 21 days of incu- bation Method # 3. The Micro-chamber Technique: A drop of liquid nutrient medium containing single cell is first isolated aseptically from stock suspension culture with the help of Pasteur pipette. The culture drop is placed on the centre of a ‘ sterile microscopic slide and ringed with sterile paraffin oil. The whole micro-chamber slide is placed in a petri-dish and is incubated to give rise to single cell clone Method # 4. The Nurse Callus Technique: This method is a modification of petridish plating method and the paper raft nurse culture method. Single cells are plated on to agar medium in a petridish. Two or three callus masses (Nurse tissue) derived from the same plant tissue are also embedded directly along with the single cells in the same medium Method # 5. The Micro-droplet Technique: Single cells are cultured in special Cuprak dishes which have two chambers—a small outer chamber and a large inner chamber. Each well of the inner chamber is filled with a micro-drop of liquid medium containing isolated single cell. The outer chamber is filled with sterile distilled water to maintain the humidity inside the dish The cell colony derived from the single cell is transferred on to fresh solid or semisolid medium Micropropagation The science and art of plant tissue culture had its roots in the vision of the Austrian botanist Gottlieb Haberlandt who visualized in 1902 the ability ‘‘to culture isolated vegetative cells from higher plants in simple nutrient solutions.’’ The term “micropropagation” refers to the large-scale multiplication of plants by a variety of tissue, and cell and organ culture methods in closed vessels with defined culture media and under controlled environmental conditions. Micropropagation Micropropagation is now an integral part of the plant propagation industry, complementing or replacing other methods of clonal vegetative propagation (cutting, grafting, division, and separation), or in some cases also propagation by seeds. The ability to regenerate whole plants from in vitro culture of pollen, protoplasts, and cell suspensions resulted in new disciplines of plant science: somatic cell genetics and metabolite production; somatic hybrids; production of haploid plants; selection of variants and mutants; and improved generation of metabolites. Micropropagation Objectives of Micropropagation 1. Large-scale clonal propagation where conventional vegetative propagation is not possible or practical 2. Initial rapid clonal propagation of new varieties (with offspring from sexual breeding and rare species selected from the wild) 3. Embryo rescue where seed and embryo germination is done in vitro to save otherwise nonviable sexual offspring Micropropagation 4. Recovery of pathogen-free propagation material 5. In vitro gene-banks for crop improvement 6. Breeding by somatic cell genetics (with haploid production from in vitro cultured pollen, somatic fusion of protoplasts or in vitro selection of somaclonal variation) 7. Initial generation of transgenic (genetically engineered) plants BIOLOGICAL PRINCIPLES OF MICROPROPAGATION Micropropagation relies on the 1. Totipotency of plant cells is a unique feature where all live explants, or even isolated cells, are able to give rise to a new plant when given the proper culture conditions. 2. Induced and enhanced cell division is done by various wounding effects and exposure to the new nutritional and hormonal components of culture medium and new environmental conditions. 3. The processes include expression of specific wound-associated growth factors and signaling molecules (e.g. systemin and jasmonic acid), changes in the tissue endogenous hormone balance, metabolic activation. BIOLOGICAL PRINCIPLES OF MICROPROPAGATION Micropropagation relies on the 4. Regeneration of events which leads to organogenesis (organ formation, i.e. shoots and/or roots) or to somatic embryogenesis (formation of seed- like embryos from somatic cells). 5. Regeneration and organization are extremely complicated and controlled biological processes such as the determination of cell polarity, cell cycle factors, hormonal and metabolic signaling, proper temporal and spatial activation of specific genes, and controlled cytoskeleton organization. Axillary bud proliferation Axillary bud proliferation in vitro is usually considered a convenient route for micropropagation. Since axillary bud proliferation typically does not include a callus stage, the probability of somaclonal variation is lower than in true organogenesis. A very large number of otherwise quiescent axillary shoot buds grow extensively when the shoot tips, or even small apical meristems, are excised and cultured in an appropriate medium, usually containing high concentrations of cytokinins Organogenesis: Formation of Shoots, Roots, and Specialized Structures Organogenesis from explants, or from callus and cell cultures, results in de novo formation of shoots and/or roots. These two events may take place simultaneously during culture, but frequently either shoots or, less frequently, roots are formed first. Organogenesis starts with a distinct organization of a group of a few meristematic cells (meristemoids). Organogenesis: Formation of Shoots, Roots, and Specialized Structures Further stages of organization, differentiation, and growth include the formation of functional vascular connections between the developing shoots and roots, finally giving rise to plantlets in vitro. New plantlets that are removed from the culture vessels, acclimatized under ex vitro conditions and subsequently, cultivated under conventional greenhouse or field conditions. In some plant species, organogenesis brings about the formation of modified shoots and roots, usually, storage organs such as bulbs and tubers. Somatic embryogenesis Somatic embryogenesis is different from organogenesis in that regeneration and organization is bipolar, that is, a progenitor cell divides unequally, forming simultaneously a shoot and a root meristem that give rise to a group of cells known as proembryonic masses. Somatic embryogenesis Usually, a two-stage culture is involved First, induction of proembryonic masses, which is frequently favored by an initial exposure of the tissue to 2,4-D or other auxin-type growth regulators. Afterwards, the proembryonic cultures are transferred to a medium with a modified composition from which the auxin has been removed or its concentration reduced. Somatic embryogenesis First, ‘‘globular’’ embryonic structures are formed. Then a ‘‘heart’’ stage in which the shoot and root meristems can be clearly distinguished at the two poles, and finaly to the ‘‘torpedo’’ stage at which elongation of the shoot, and especially the root, takes place, and vascular connections between the two are established. Technical Aspects of Micropropagation Explant quality is primary to the success of any micropropagation scheme. Choosing particular cells, tissues or organs as explants is a function of the plant species and cultivar, micropropagation goals, tissue-culture methods, and the specific condition of the source plants. Explants range from barely visible true meristems to larger meristematic clusters, shoot tips, leaf, stem, or flower segments as well as seeds, pollen, or anthers Technical Aspects of Micropropagation Asepsis (Axenic Culture) Micropropagation requires an aseptic environment and axenic cultures. This is ideally achieved with surface sterilization of contamination or disease-free explants, followed by initiation, establishment, and subculture under aseptic conditions using presterilized culture media and growth vessels. Technical Aspects of Micropropagation Clean Room Technology A clean room is defined as ‘‘a room in which the concentration of airborne particles is controlled, and which is constructed and used in a manner to minimize the introduction, generation, and retention of particles inside the room, and in which other relevant parameters, for example, temperature, humidity, and air pressure, are controlled as necessary’’. it is necessary to create workspaces with minimal levels of particulate matter, including fungal and bacterial spores. Manipulation of plant material is usually carried out in laminar flow workspaces. Technical Aspects of Micropropagation Surface Sterilization of Plant Material Plant parts containing explants, that is, shoot tips, seeds, and stems, are first cleansed, often with combinations of detergents, disinfectants, agitation, and water rinses. Disinfection treatments usually include household bleach and/or alcohol. Technical Aspects of Micropropagation Culture Media An appreciation and knowledge of the nutritional requirements of cultured cells and tissues is invaluable, not only in a decision on the type of media to use, but also in preparation and storage. The most widely used, standard medium formulation is Murashige and Skoog (MS) Additional factors, including complex, undefined materials, such as coconut milk, yeast extract, or protein hydrolysates, may be beneficial for certain plants or growth stages. Technical Aspects of Micropropagation Essential Equipment Essential equipment for media preparation include an autoclave, pH meter, analytical and semi-analytical scales and measuring instruments and containers; heating, stirring, and pouring equipment; refrigeration; a water distillation/deionization system; storage facilities; and a washing up area for tools and equipment. Technical Aspects of Micropropagation Essential Equipment The microenvironment, inside the culture vessel, may be influenced not only by the generation and absorption of gases by the plantlet and the culture medium, but also by the gas exchange between the room and the vessel. Most containers should be small, usually holding from 1 to 100 plantlets Technical Aspects of Micropropagation Three sets of environmental conditions must be monitored (i) The current season and environment, including temperature and humidity, in the non-aseptic, ambient areas of the laboratory; (ii) The controlled environment of the growing room, including sanitation, temperature, humidity, air flow and circulation, light quality and intensity, and day length; (iii) The microenvironment of the growth vessel including asepsis, temperature, humidity, gas exchange, light intensity and quality, nutrition, and potential sources of stress. Technical Aspects of Micropropagation Temperature In vitro growth conditions for most plants require ambient temperatures of 22–27◦C. Room temperature is somewhat lower than illuminated shelf temperature with the gap depending on the level of air circulation, amount of thermal energy (heat) produced by the light sources above the shelves, and the amount of insulation between a light source and the shelf above. Technical Aspects of Micropropagation Relative Humidity Micro-humidity in the plant growth vessel, at 88%–94% or less. Growth room ambient humidity is usually 50%–80%. Room RH lower than 40% can result in growth medium desiccation, increased salt concentration, and drying out of plant material. Room RH higher than 85% may increase microbial contamination and vitrification. Technical Aspects of Micropropagation Light Traditionally, Fluorescent lamps have been the primary light source used in micropropagation. Photon density is between 20 and 200 μmol/m2/s , with a standard photoperiod of 12– 16 h. The use of light emitting diodes (LED) have emerged in micropropagation. It is possible to fine tune and easily alter light spectra and intensity using combinations of LEDs. For example, cool white, warm white, red and/or blue light light emitting diodes (LED) LEDs may be used to effectively illuminate in vitro plantlets on a single shelf. Stages and Major Operations of Micropropagation Five critical and ordered operational stages are involved, (0) selection and preparation of the explant source; (I) Establishment of viable explants in culture; (II) rapid regeneration of numerous propagules; (III) establishment of complete rooted plantlets (IV) acclimatization and establishment ex vitro Stage 0: Explant Source and Mother Plants. The success of micropropagation is largely dependent on the quality of the source plant. Source plants should provide the following characteristics: 1. It should be true-to-type representative of the desired species and cultivar. 2. It is free from disease and endogenous contamination 3. It is viable and vigorous, that is, potentially able to respond to culture conditions that induce intensive cell division and regeneration. Stage I: Explant Establishment in Culture The initial explant may range in size from 0.1 mm (e.g. meristems used for establishing virus-free plants) to about 1 cm (e.g. bulb scales and stems). During this stage, lasting from 1 week to 1–3 months, or even longer, the explant is established in culture, resulting in tissue activation and multiplication With continual visual monitoring, this stage serves as the baseline for screening for microbial contamination and fitness Stage II: Rapid Multiplication Primary explants that have successfully passed through Stage I are transferred aseptically to Stage II for generation of numerous clonal propagules. The types of regeneration and proliferation are largely dependent on growth regulator combinations. A high proportion of cytokinins usually stimulates continued multiplication of axillary or adventitious shoots, and a higher proportion of specific auxins is required for callus proliferation with the generation of somatic embryogenesis. The duration of this stage is potentially unlimited, but usually lasts from several months to 1 to 2 years. Stage III: Plantlet Establishment, Elongation, and Rooting Stage III is designed to arrest rapid multiplication and to induce the establishment of a fully developed plantlet, i.e., shoot elongation, root formation and when required, formation of storage organs that serve as independent propagation units (bulbs, corms, and tubers) This stage also provide the conditions for stimulation of photosynthesis, stomatal function, and other physiological changes that are required to establish autotrophic growth in the ex vitro acclimatization stage Stage III: Plantlet Establishment, Elongation, and Rooting This is achieved by culture media and environmental modifications such as reduction of cytokinin concentrations or their total elimination, the addition of or increase in auxins and/or reduced sugar levels, and often, increased light intensity. Recalcitrance, or general lack of success in rooting in vitro plants has been because of incomplete understanding of the processes, or the inability to apply existing knowledge Root formation is split in precise stages: induction, cellular activation, orientation, organization, and rooting expression, with the emergence of the first root being the most direct expression of the rooting capacity. Stage IV: Acclimatization in vitro culture leads to formation of plantlets with morphology, anatomy, and physiology different from naturally grown plants, including reduced leaf wax deposits, nonfunctional stomata, aberrant photosynthetic activity, reduced root hair development, and so on. After transfer from in vitro to ex vitro culture stage IV, acclimatization is needed to correct abnormalities and to ensure proper growth and survival of the plants. Initially, plantlets are placed under low light and temperatures and humidity closely resembling in vitro conditions; an incubator for ex vitro plants Stage IV: Acclimatization Over the following weeks, light intensity is gradually raised and ambient temperature and humidity are regulated to the greenhouse environment. Most laboratories transplant into a uniform medium that adequately supports the plant, has a suitable pH, is well buffered, is reproducible, and is sufficiently porous to allow adequate drainage and aeration In vitro plants that are unable to survive this stage must be considered unsuccessful, thereby losing the entire investment in the micropropagation process ADVANTAGES OF MICROPROPAGATION Production of a Very Large Number of Clonal Propagules within a Relatively Short Time Span e.g. thousands and millions of in vitro plants can be produced from relatively few selected source plants Production of Disease-Free Plant Material with the Possibility of Eliminating Viral, Bacterial, and Fungal Contamination. Production of a Large Stock of True-to-Type Clonal Propagation Material i.e. is the ability to control and guarantee a consistently high degree of likeness between source plant characteristics and the final plant product. ADVANTAGES OF MICROPROPAGATION The ability to safely ship large quantities of plant material quickly, efficiently, and relatively inexpensively. Tens of thousands of in vitro or several thousand acclimatized plants can be packed into a cubic meter of air shipping space. The possibility of bringing new technologies or newly bred plants and selections to market, rapidly and in large quantities. Breeding and selection of commercial plant products have been lengthy, often lifetime processes. Micropropagation and the tracking and testing of new genotypes or field selections may be achieved in months or a few years. DISADVANTAGES OF MICROPROPAGATION In 1988, Pierik listed ‘‘Handicaps for Large-Scale Commercial Application of Micropropagation’’. They included the following in vitro issues: 1. frequent mutations;Tissue culture conditions can induce genetic changes in the plants, leading to variability which may be undesirable if true-to-type propagation is the goal. 2. lack of basic knowledge of organogenesis or embryogenesis; Insufficient understanding of the developmental processes (organogenesis and embryogenesis) in some species hinders the development of effective micropropagation protocols 3. exceptional difficulty with woody species; Micropropagation of woody plants is often more challenging compared to herbaceous plants due to factors like complex tissue differentiation and secondary metabolites 4. internal infections; Cultures can be contaminated with bacteria or fungi that reside within the plant tissue, making them difficult to detect and eliminate. DISADVANTAGES OF MICROPROPAGATION 5. vitrification, and toxic exudates; Cultures can experience abnormal glassy states (vitrification) or release toxic substances, harming growth. 6. increased ethylene and CO2 levels; Unfavorable gas buildup in culture vessels can negatively impact development. 7. neglect of the role of physical growth factors (light, temperature, humidity, and the gas phase);failure to optimize light, temperature, humidity, and gas composition can lead to poor results 8. losses on transfer from in vitro to acclimatization; Moving plants from sterile lab conditions to the real world (acclimatization) often results in significant losses. 9. labor costs and lack of realistic mechanization; Micropropagation is often labor-intensive and lacks automation, increasing expenses 10. many newly developed techniques are not economically viable; commercial production is often insufficiently controlled.’’ Commercial Aspects of Micropropagation Establishing an average size commercial micropropagation facility, and the accompanying agricultural, research, marketing, and management systems necessary to support it, may cost more than US $1 million. In the more developed world, labor remains the major economic obstacle, and may represent up to 85% of costs. In developing countries, electricity is often the greatest limiting economic factor. The agricultural community is slow to accept change, and demands significant justification for the cost, time, and effort involved. Commercial Aspects of Micropropagation The quality issues that are common in traditional manufacturing have also arisen in commercial plant technology. Economic survival will be awarded only to those micropropagation companies and laboratories that are able and willing to comply. A single flaw in the system seldom results in economic failure; it is usually an accumulation of some or all of the above disadvantages that determine the ultimate success or failure of a project or a company. Commercial Aspects of Micropropagation Plant tissue culture is carried out in most countries of the world. Commercial foci are found in the United States, Europe, India, and in the Asian Pacific Rim (Japan, Taiwan, Thailand, and Australia/New Zealand). China has significant research in and production of micropropagated plant material, but it appears that most plant tissue culture is subsidized or state-run commercial production. Commercial Aspects of Micropropagation Although, most tissue-cultured plant material remains in China or its region of production, 10%–20% of in vitro or acclimatized plantlets are exported. Much of the European production is shipped in vitro to centers of cheaper labor and warmer climate for acclimatization and planting out, and a large quantity of the finished plants is subsequently shipped back to Europe for distribution and sale. Major Crops in Commercial Micropropagation The annual volume of plants micropropagated from tissue culture is estimated at hundreds of millions, representing tens of thousands of varieties Tissue culture production of ornamental plants in general and orchids in particular, forms the basis for an entire horticultural industry Orchids always appreciated and in demand, were once very rare in the world flower market. Orchids represent the first floricultural crop successfully mass propagated through tissue culture. Major Crops in Commercial Micropropagation Thailand is the world’s largest micropropagated orchid producer with production in 2005 of 44.6 million tons of flowers and potted plants. Taiwan, India, and China are all increasing production and technology and investing heavily in the production and export industry. Major Crops in Commercial Micropropagation Banana They are the main fruit in international trade and in terms of volume they are the first in exported fruit. By the 1990s, tens of millions of micropropagated banana plants had been provided to commercial plantations in America and Africa. Initially, most of these came from Europe and Israel. Major banana producing countries such as India or Brazil are hardly involved in international trade. Major Crops in Commercial Micropropagation Banana The outbreak of Panama disease or Fusarium wilt, caused by the fungus Fusarium oxysporum f. sp. cubense race 4, and attacking the major commercial varieties of dessert bananas, has highlighted the importance of providing large quantities of disease-free banana plantlets to be grown. Major Crops in Commercial Micropropagation Sugarcane Micropropagated sugarcane plants have been used in Brazil and Indonesia for almost three decades Rising demand for both sugar and ethanol are creating opportunity for micropropagation of sugarcane. The meristem culture is the only method to remove the SCMV (Sugarcane mosaic virus) as the meristematic tissue remains free from virus disease. Major Crops in Commercial Micropropagation Potato Potato serves as an effective model for a variety of micropropagation methods nd technologies. Potatoes are cultivated as a valued crop in over 100 countries and about a third of all potatoes are harvested in China and India with China the largest producer worldwide. There is a huge gap between the requirement and supply of certified seed potatoes in India Major Crops in Commercial Micropropagation Potato The Central Potato Research Institute (CPRI) of the Indian Council of Agricultural Research (ICAR) produces about 2,400 tons of breeder’s seed (basic seed) every year and supplies 80% of it to the states and other agencies for its multiplication On the assumption of a 100% seed replacement rate (SRR), it meets only 10% of the total seed requirement, leaving a deficit of about 4.9 million tons Haploid Plants What is a haploid plant? Haploid plants originate from gametes (or gamete-like cells) that do not go through fertilization, but can still generate a viable individual. Haploids contain only the chromosome set found after meiosis in male (sperm cells) or female (egg cells) gametes. Depending on whether the single set of chromosomes comes from the maternal or paternal side, the plant is referred to as maternal haploid and paternal haploid, respectively. Haploid Plants What is a doubled haploid (DH) plant? In a DH plant, the chromosome set of a haploid plant has been doubled spontaneously or artificially. Chromosome doubling is necessary since haploid plants are generally frail, have reduced organ size and are not fertile. The most commonly used chemical agent to render haploid plantlets diploid is colchicine, which blocks cell division without blocking chromosome Haploid Plants What is a doubled haploid (DH) plant? This treatment acts like a ‘copy–paste’ of the haploid genome into a diploid genome. DH plants all loci are homozygous. Chromosome doubling creates ‘pure’ homozygotes or fully inbred lines The primary advantage of DH plants is to possess a phenotypic stability due to the fact that all alleles are in a homozygous state. Haploid Plants What are the different methods to produce haploid plants? 1. in vitro methods are based on the culture of haploid cells and their differentiation into haploid embryos and ultimately haploid plants. Both male (microspores or pollen) and female haploid cells (megaspores or ovules) are used, depending on the responsiveness of the cells in a given species. 2. in situ methods make use of particular pollination Haploid Plants Figure 1. Overview of doubled haploid technology. First different methods are used to create haploid plants. Then chromosome doubling on haploid plantlets results in perfect homozygous plants named doubled haploid (DH) plants. Haploid Plants In flowering plants, sexual reproduction is characterized by a unique biological process named double fertilization, which consists of two separate fusion events between male and female gametes. This double fertilization leads to a diploid embryo and triploid endosperm that represent the two major seed components. Haploid Plants In the case of fertilization with pollen from a maize ‘inducer’ line, double fertilization is impaired, resulting in seeds containing a haploid embryo with only the maternal genome (gynogenesis) What are the molecular players behind in situ haploid induction in maize? The inducing capacity of inducer lines has been recently tracked to a 4 bp insertion at the end of the coding sequence of a gene named NOT LIKE DAD (NLD) / MATRILINEAL (MTL) / ZmPHOSPHOLIPASE A1 (ZmPLA1). NLD/MTL/ZmPLA1 is specifically expressed in male gametes. It encodes a patatin-like phospholipase A localized at the plasma membrane of the male germ unit. Androgenesis First in vitro culture of anthers of Datura (Guha and Maheshwari 1964,1966) the male gametophyte (microspore or immature pollen) produces haploid plants. The basic principle is to stop the development of pollen into a gamete (sex cell) and force it to develop into a haploid plant or sporophyte. Androgenesis Androgenesis is just the reverse of gynogenesis and the mitochondrial DNA is still maternally derived. It is induced by UV irradiation of the egg to inactivate chromosomal DNA followed by fertilization with normal sperm. This results in a haploid zygote containing a single chromosome set. The normal diploid state is restored by applying pressure or temperature shock at the first cleavage. Androgenesis Anther culture (i) Select the flower buds from an elite plant and determine the stage of microspores by by staining them with fluorescent dye DAPI. (ii) Inoculate the anthers, bearing early-to-late uninucleate stage of microspores, in a nutrient medium and maintain the cultures in defined conditions. (iii) As the anthers proliferate, they produce embryos/callus which finally produce haploid plants and then diploidize them by colchicine to produce homozygous diploids. Androgenesis Microspore (Pollen) culture (i) Extract the microspores by pressing and squeezing the buds with a glass rod and filter the pollen suspension to remove anther tissue debris. (ii) Culture these microspores on a solid or liquid medium. As the microspores undergo multiple divisions, they produce multicellular and multinuclear structure. (iii) The callus/ embryos formed finally produce a haploid plants and then diploid plants by colchicine treatment Doubled haploidy A doubled haploid (DH) is a genotype formed when haploid cells undergo chromosome doubling. If the original plant was diploid, the haploid cells are monoploid, and the term doubled monoploid may be used for the doubled haploids haploid cells = MONOPLOID DOUBLE MONOPLOID = DOUBLE HAPLOID Haploid organisms derived from tetraploids or hexaploids are sometimes called dihaploids Doubled haploidy Conventional inbreeding procedures take six generations to achieve approximately complete homozygosity, whereas doubled haploidy achieves it in one generation. Doubled haploids can be produced in vivo or in vitro. Haploid embryos are produced in vivo by parthenogenesis, pseudogamy, or chromosome elimination after wide crossing. The haploid embryo is rescued, cultured, and chromosome-doubling produces doubled haploids. IN VIVO for haploid embryos Doubled haploidy The in vitro methods include gynogenesis (ovary and flower culture) and androgenesis (anther and microspore culture). Androgenesis is the preferred method. IN VITRO for haploid embryos Another method of producing the haploids is wide crossing. In barley, haploids can be produced by wide crossing with the related species Hordeum bulbosum; fertilization is affected, but during the early stages of seed development the H. bulbosum chromosomes are eliminated leaving a haploid embryo. Speed: why is DH useful? Traditional breeding to achieve homozygosity (AA or aa) can take many generations of crossing and selection. Doubled haploidy drastically shortens this process, allowing breeders to develop new varieties much faster. Doubled haploidy Efficiency: It allows breeders to quickly fix desired traits (make them homozygous) in a population. This is essential for creating inbred lines used in hybrid seed production. Genetic Uniformity: Doubled haploid lines are completely homozygous, ensuring that all plants within the line are genetically identical. This is important for research and commercial purposes. In DH method only two types of genotypes occur for a pair of alleles, A and a, with the frequency of ½ AA and ½ aa, while in diploid method three genotypes occur with the frequency of ¼ AA, ½ Aa, ¼ aa. Thus, if AA is desirable genotype, the probability of obtaining this genotype is higher in haploid method than in diploid method. " This is because doubled haploidy allows breeders to quickly and efficiently produce plants that are homozygous for desired traits, saving time and resources compared to traditional breeding methods Applications of DHs plant breeding As the quantitative trait loci (QTL) effects are small and highly influenced by environmental factors, accurate phenotyping with replicated trials is needed. This is possible with doubled haploidy organisms because of their true breeding nature and because they can conveniently be produced in large numbers. Applications of DHs plant breeding In backcross conversion, genes are introgressed from a donor cultivar or related species into a recipient elite line through repeated backcrossing. A problem in this procedure is being able to identify the lines carrying the trait of interest at each generation. The problem is particularly acute if the trait of interest is recessive, as it will be present only in a heterozygous condition after each backcross. Applications of DHs plant breeding In bulked segregant analysis, a population is screened for a trait of interest and the genotypes at the two extreme ends form two bulks. Then the two bulks are tested for the presence or absence of molecular markers. Since the bulks are supposed to contrast in the alleles that contribute positive and negative effects, any marker polymorphism between the two bulks indicates the linkage between the marker and trait of interest. DH population has particular advantage in that they are true breeding and can be tested repeatedly. Embryo Rescue Interspecific hybridization involves crossing two different species within the same genus, aiming to combine desirable traits from each parent species. However, this process often encounters a significant hurdle – the high rate of embryo abortion. The phenomenon of embryo abortion in interspecific hybridization arises due to genetic and physiological differences between the parent species. These differences can result in incompatible interactions during fertilization and early embryo development, hindering the establishment of a robust and viable plant. Embryo Rescue Embryo rescue (ER) is a set of techniques commonly used to rescue immature/mature-lethal embryos, and hybrid embryos generated from interspecific and intergeneric crosses unable to survive in vivo or during traditional plant breeding practices. The procedure involves excising immature or lethal embryos and culturing them in vitro on a specific nutrient culture medium. The developmental differences between dicots and monocots need to be considered when attempting to recover immature embryos. Embryo Rescue Embryo rescue (ER) is a set of techniques commonly used to rescue immature/mature-lethal embryos, and hybrid embryos generated from interspecific and intergeneric crosses unable to survive in vivo or during traditional plant breeding practices. The procedure involves excising immature or lethal embryos and culturing them in vitro on a specific nutrient culture medium. The developmental differences between dicots and monocots need to be considered when attempting to recover immature embryos. The Origins of Embryo Rescue: A Brief Historical Overview Charles Bonnet (1720–1793) documented ER for the first time in the 18th century. He achieved remarkable success by excising mature embryos of Phaseolus vulgaris and Fagopyrum and transplanting them into the soil where they could grow. In 1904, E. Hanning successfully introduced mature embryos into the in vitro tissue culture of some Brassicaceae species. The culture method was used by Brown (1906) to assess the efficacy of various organic nitrogenous compounds in promoting the growth of isolated barley (Hordeum vulgare) embryos nourished in a mineral saline medium supplemented with sucrose. The Origins of Embryo Rescue: A Brief Historical Overview In 1924, Kurt Dieterich was a precursor in testing the possibility of embryo culture both in mature and immature explants The first application of the in vitro culture of zygotic immature embryos from interspecific cross dates to 1925, when Friedrich Laibach used this technique to prevent embryonic abortion in Lilium perenne × L. austriacum cross. Pre-Zygotic Techniques to Overcome Them in vitro fertilization, the reproductive organs (stigma and anthers) are isolated, followed by controlled fusion of the male and female gametes. In addition, it is necessary to set up a culture medium that allows the zygote to develop to full maturity, followed by seed germination. The graft-on-style method is useful when pollen germinates on the stigma but an incompatibility reaction occurs between pollen and style. In this case, the pollination of the entire gynoecium in vitro would not yield fruitful results, as all pollen tubes would be halted at the early part of the style, even under in vitro conditions. Pre-Zygotic Techniques to Overcome Them In the grafting technique is employed, wherein an incompatible style is replaced with a compatible one. This allows the pollen tube to follow its normal growth with the compatible style grafted into the ovary. Alternatively, in species with this type of genetic incompatibility, one proceeds by either shortening the style and micrografting the stigma at the base of the style or by directly depositing pollen on the ovule. Post-Zygotic Techniques Failure to form endosperm commonly accompanies interspecific and intergeneric crosses, and this phenomenon is often present in embryo-lethal mutants. in vitro culture of immature embryos has emerged as the most widely employed technique. Equally important are the following aspects: the excision procedure, the preservation of embryo integrity, the protocol of sterilization methods, the culture medium composition, and the environmental conditions encompassing light intensity, quality, and temperature. Intrinsic factors, such as embryo size and developmental stage, also play an important role