Physiology Hormones (Adrenal, Thyroid) PDF
Document Details
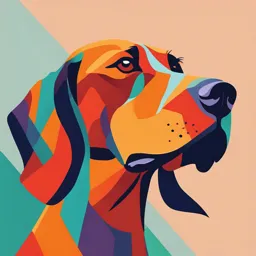
Uploaded by A1StudyFlex
null
Tags
Summary
This document provides a comprehensive overview of coordination functions of the body by chemical messengers, focusing on endocrine and neuroendocrine hormones. It details the structure, function, and synthesis of various hormones, including examples.
Full Transcript
CHAPTER 75 COORDINATION OF BODY FUNCTIONS BY CHEMICAL MESSENGERS The multiple activities of the cells, tissues, and organs of the body are coordinated by the interplay of several types of chemical messenger systems: 1. Neurotransmitters are released by axon terminals of neurons into the synaptic jun...
CHAPTER 75 COORDINATION OF BODY FUNCTIONS BY CHEMICAL MESSENGERS The multiple activities of the cells, tissues, and organs of the body are coordinated by the interplay of several types of chemical messenger systems: 1. Neurotransmitters are released by axon terminals of neurons into the synaptic junctions and act locally to control nerve cell functions. 2. Endocrine hormones are released by glands or specialized cells into the circulating blood and influence the function of target cells at another location in the body. 3. Neuroendocrine hormones are secreted by neurons into the circulating blood and influence the function of target cells at another location in the body. 4. Paracrines are secreted by cells into the extracellular fluid and affect neighboring target cells of a different type. 5. Autocrines are secreted by cells into the extracellular fluid and affect the function of the same cells that produced them. 6. Cytokines are peptides secreted by cells into the extracellular fluid and can function as autocrines, paracrines, or endocrine hormones. Examples of cytokines include the interleukins and other lymphokines that are secreted by helper cells and act on other cells of the immune system (see Chapter 35). Cytokine hormones (e.g., leptin) produced by adipocytes are sometimes called adipokines. In the next few chapters, we discuss mainly the endocrine and neuroendocrine hormone systems, keeping in mind that many of the body’s chemical messenger systems interact with one another to maintain homeostasis. For example, the adrenal medullae and the pituitary gland secrete their hormones primarily in response to neural stimuli. The neuroendocrine cells, located in the hypothalamus, have axons that terminate in the posterior pituitary gland and median eminence and secrete several neurohormones, including antidiuretic hormone, oxytocin, and hypophysiotropic hormones, which control the secretion of anterior pituitary hormones. The endocrine hormones are carried by the circulatory system to cells throughout the body, including the nervous system in some cases, where they bind with receptors and initiate many cell reactions. Some endocrine hormones affect many different types of cells of the body; for example, growth hormone from the anterior pituitary gland causes growth in most parts of the body, and thyroxine from the thyroid gland increases the rate of many chemical reactions in almost all the body’s cells. Other hormones affect mainly specific target tissues because these tissues have abundant receptors for the hormone. For example, adrenocorticotropic hormone from the anterior pituitary gland specifically stimulates the adrenal cortex, causing it to secrete adrenocortical hormones, and the ovarian hormones have their main effects on the female sex organs and the secondary sexual characteristics of the female body. Figure 75-1 shows the anatomical loci of the major endocrine glands and endocrine tissues of the body, except for the placenta, which is an additional source of the sex hormones. Table 75-1 provides an overview of the different hormone systems and their major actions. The multiple hormone systems play a key role in regulating almost all body functions, including metabolism, growth and development, water and electrolyte balance, reproduction, and behavior. For example, without growth hormone, a person would have very short stature. Without thyroxine and triiodothyronine from the thyroid gland, almost all the chemical reactions of the body would become sluggish and the person would become sluggish as well. Without insulin from the pancreas, the body’s cells could use little of the food carbohydrates for energy. And without the sex hormones, sexual development and sexual functions would be absent.␣ CHEMICAL STRUCTURE AND SYNTHESIS OF HORMONES Three general classes of hormones exist: 1. Proteins and polypeptides, including hormones secreted by the anterior and posterior pituitary gland, the pancreas (insulin and glucagon), the parathyroid 915 UNIT XIV Introduction to Endocrinology UNIT XIV Endocrinology and Reproduction Hypothalamus Pineal gland Parathyroid glands (behind thyroid gland) Pituitary gland Thyroid gland Thymus gland Stomach Adrenal glands Pancreas Kidney Adipose tissue Small intestine Ovaries (female) Steroid Hormones Are Usually Synthesized From Cholesterol and Are Not Stored. Steroid hormones have Testes (male) Figure 75-1. Anatomical loci of the principal endocrine glands and tissues of the body. gland (parathyroid hormone), and many others (see Table 75-1). 2. Steroids secreted by the adrenal cortex (cortisol and aldosterone), the ovaries (estrogen and progesterone), the testes (testosterone), and the placenta (estrogen and progesterone). 3. Derivatives of the amino acid tyrosine, secreted by the thyroid (thyroxine and triiodothyronine) and the adrenal medullae (epinephrine and norepinephrine). There are no known polysaccharides or nucleic acid hormones. Polypeptide and Protein Hormones Are Stored in Secretory Vesicles Until Needed. Most of the hormones in the body are polypeptides and proteins. These hormones range in size from small peptides with as few as 916 three amino acids (e.g., thyrotropin-releasing hormone) to proteins with almost 200 amino acids (e.g., growth hormone and prolactin). In general, polypeptides with 100 or more amino acids are called proteins, and those with fewer than 100 amino acids are referred to as peptides. Protein and peptide hormones are synthesized on the rough end of the endoplasmic reticulum of the different endocrine cells, in the same fashion as most other proteins (Figure 75-2). They are usually synthesized first as larger proteins that are not biologically active (preprohormones) and are cleaved to form smaller prohormones in the endoplasmic reticulum. These prohormones are then transferred to the Golgi apparatus for packaging into secretory vesicles. In this process, enzymes in the vesicles cleave the prohormones to produce smaller, biologically active hormones and inactive fragments. The vesicles are stored within the cytoplasm, and many are bound to the cell membrane until their secretion is needed. Secretion of the hormones (as well as the inactive fragments) occurs when the secretory vesicles fuse with the cell membrane and the granular contents are extruded into the interstitial fluid or directly into the blood stream by exocytosis. In many cases, the stimulus for exocytosis is increased cytosolic calcium concentration caused by depolarization of the plasma membrane. In other cases, stimulation of an endocrine cell surface receptor causes increased cyclic adenosine monophosphate (cAMP) and subsequently activation of protein kinases that initiate secretion of the hormone. The peptide hormones are water soluble, allowing them to enter the circulatory system easily, where they are carried to their target tissues.␣ a chemical structure that is similar to cholesterol, and in most cases are synthesized from cholesterol. They are lipid soluble and consist of three cyclohexyl rings and one cyclopentyl ring combined into a single structure (Figure 75-3). Although there is usually very little hormone storage in steroid-producing endocrine cells, large stores of cholesterol esters in cytoplasm vacuoles can be rapidly mobilized for steroid synthesis after a stimulus. Much of the cholesterol in steroid-producing cells comes from the plasma, but there is also de novo synthesis of cholesterol in steroid-producing cells. Because steroids are highly lipid soluble, once they are synthesized, they can simply diffuse across the cell membrane and enter the interstitial fluid and then the blood.␣ Amine Hormones Are Derived From Tyrosine. The two groups of hormones derived from tyrosine, the thyroid and the adrenal medullary hormones, are formed by the actions of enzymes in the cytoplasmic compartments of glandular cells. The thyroid hormones are synthesized and stored in the thyroid gland and incorporated into macromolecules of the protein thyroglobulin, which is stored in large follicles within the thyroid gland. Hormone secretion occurs when the amines are split from Chapter 75 Introduction to Endocrinology Table 75-1 Endocrine Glands, Hormones, and Their Functions and Structure Hormones Major Functions Chemical Structure Hypothalamus (Chapter 76) Thyrotropin-releasing hormone Corticotropin-releasing hormone Growth hormone–releasing hormone Growth hormone inhibitory hormone (somatostatin) Gonadotropin-releasing hormone Dopamine or prolactininhibiting factor Stimulates secretion of thyroid-stimulating hormone and prolactin Causes release of adrenocorticotropic hormone Causes release of growth hormone Peptide Inhibits release of growth hormone Peptide Causes release of luteinizing hormone and follicle-stimulating hormone Inhibits release of prolactin Peptide Growth hormone Stimulates protein synthesis and overall growth of most cells and tissues Stimulates synthesis and secretion of thyroid hormones (thyroxine and triiodothyronine) Stimulates synthesis and secretion of adrenocortical hormones (cortisol, androgens, and aldosterone) Promotes development of the female breasts and secretion of milk Causes growth of follicles in the ovaries and sperm maturation in Sertoli cells of testes Stimulates testosterone synthesis in Leydig cells of testes; stimulates ovulation, formation of corpus luteum, and estrogen and progesterone synthesis in ovaries Peptide Increases water reabsorption by the kidneys and causes vasoconstriction and increased blood pressure Stimulates milk ejection from breasts and uterine contractions Peptide Thyroxine (T4) and triiodothyronine (T3) Calcitonin Increases the rates of chemical reactions in most cells, thus increasing body metabolic rate Promotes deposition of calcium in the bones and decreases extracellular fluid calcium ion concentration Amine Cortisol Has multiple metabolic functions for controlling metabolism of proteins, carbohydrates, and fats; also has anti-inflammatory effects Increases renal sodium reabsorption, potassium secretion, and hydrogen ion secretion Steroid Anterior pituitary (Chapter 76) Thyroid-stimulating hormone Adrenocorticotropic hormone Prolactin Follicle-stimulating hormone Luteinizing hormone Posterior pituitary (Chapter 76) Antidiuretic hormone (also called vasopressin) Oxytocin Thyroid (Chapter 77) Adrenal cortex (Chapter 78) Aldosterone Peptide Peptide Amine Peptide Peptide Peptide Peptide Peptide Peptide Peptide Steroid Adrenal medulla (Chapter 61) Norepinephrine, epinephrine Same effects as sympathetic stimulation Amine Pancreas (Chapter 79) Insulin (beta cells) Promotes glucose entry in many cells, and in this way controls carbohydrate metabolism Peptide Glucagon (α cells) Increases synthesis and release of glucose from the liver into the body fluids Peptide Parathyroid (Chapter 80) Parathyroid hormone Controls serum calcium ion concentration by increasing calcium absorption by the gut and kidneys and releasing calcium from bones Peptide Testes (Chapter 81) Testosterone Promotes development of male reproductive system and male secondary sexual characteristics Steroid Ovaries (Chapter 82) Estrogens Promotes growth and development of female reproductive system, female breasts, and female secondary sexual characteristics Stimulates secretion of “uterine milk” by the uterine endometrial glands and promotes development of secretory apparatus of breasts Steroid Progesterone UNIT XIV Gland/Tissue Steroid Continued 917 UNIT XIV Endocrinology and Reproduction Table 75-1 Endocrine Glands, Hormones, and Their Functions and Structure—cont’d Gland/Tissue Hormones Major Functions Chemical Structure Placenta (Chapter 83) Human chorionic gonadotropin Human somatomammotropin Estrogens Progesterone Promotes growth of corpus luteum and secretion of estrogens and progesterone by corpus luteum Probably helps promote development of some fetal tissues, as well as the mother’s breasts See actions of estrogens from ovaries. See actions of progesterone from ovaries. Peptide Renin Catalyzes conversion of angiotensinogen to angiotensin I (acts as an enzyme) Increases intestinal absorption of calcium and bone mineralization Increases erythrocyte production Peptide Kidney (Chapter 26) 1,25-Dihydroxycholecalciferol Erythropoietin Peptide Steroid Steroid Steroid Peptide Heart (Chapter 22) Atrial natriuretic peptide Increases sodium excretion by kidneys, reduces blood pressure Peptide Stomach (Chapter 65) Gastrin Stimulates hydrogen chloride secretion by parietal cells Peptide Small intestine (Chapter 65) Secretin Stimulates pancreatic acinar cells to release bicarbonate and water Stimulates gallbladder contraction and release of pancreatic enzymes Peptide Inhibits appetite, stimulates thermogenesis Peptide Cholecystokinin Adipocytes (Chapter 72) Nucleus Leptin Peptide CH2OH DNA C HO Transcription Synthesis O mRNA O O HO OH O Cortisol OH CH2OH HC C O Aldosterone OH Translation Endoplasmic reticulum O Testosterone HO Estradiol Figure 75-3. Chemical structures of several steroid hormones. Packaging Golgi apparatus Storage Secretory vesicles ↑ Ca2+ ↑ cAMP Secretion Extracellular fluid Stimulus Figure 75-2. Synthesis and secretion of peptide hormones. The stimulus for hormone secretion often involves changes in intracellular calcium or changes in cyclic adenosine monophosphate (cAMP) in the cell. 918 thyroglobulin, and the free hormones are then released into the blood stream. After entering the blood, most of the thyroid hormones combine with plasma proteins, especially thyroxine-binding globulin, which slowly releases the hormones to the target tissues. Epinephrine and norepinephrine are formed in the adrenal medulla, which normally secretes about four times more epinephrine than norepinephrine. Catecholamines are taken up into preformed vesicles and stored until secreted. Similar to the protein hormones stored in secretory granules, catecholamines are also released from adrenal medullary cells by exocytosis. Once the catecholamines enter the circulation, they can exist in the plasma in free form or in conjugation with other substances.␣ Chapter 75 Introduction to Endocrinology HORMONE SECRETION, TRANSPORT, AND CLEARANCE FROM THE BLOOD mones, such as norepinephrine and epinephrine, are secreted within seconds after the gland is stimulated and may develop full action within another few seconds to minutes; the actions of other hormones, such as thyroxine or growth hormone, may require months for full effect. Thus, each of the different hormones has its own characteristic onset and duration of action—each tailored to perform its specific control function.␣ Concentrations of Hormones in the Circulating Blood and Hormonal Secretion Rates. The concentra- tions of hormones required to control most metabolic and endocrine functions are incredibly small. Their concentrations in the blood range from as little as 1 picogram (which is one millionth of one millionth of a gram) in each milliliter of blood up to at most a few micrograms (a few millionths of a gram) per milliliter of blood. Similarly, the rates of secretion of the various hormones are extremely small, usually measured in micrograms or milligrams per day. We shall see later in this chapter that highly specialized mechanisms are available in the target tissues that allow even these minute quantities of hormones to exert powerful control over the physiological systems.␣ FEEDBACK CONTROL OF HORMONE SECRETION Negative Feedback Prevents Overactivity of Hormone Systems. Although the plasma concentrations of many hormones fluctuate in response to various stimuli that occur throughout the day, all hormones studied thus far appear to be closely controlled. In most cases, this control is exerted through negative feedback mechanisms (described in Chapter 1) that ensure a proper level of hormone activity at the target tissue. After a stimulus causes release of the hormone, conditions or products resulting from the action of the hormone tend to suppress its further release. In other words, the hormone (or one of its products) has a negative feedback effect to prevent oversecretion of the hormone or overactivity at the target tissue. Feedback regulation of hormones can occur at all levels, including gene transcription and translation steps involved in the synthesis of hormones and steps involved in processing hormones or releasing stored hormones. The controlled variable is sometimes the degree of activity of the target tissue rather than the synthesis or secretory rates of the hormone. Therefore, only when the target tissue activity rises to an appropriate level will Surges of Hormones Can Occur With Positive Feedback. In a few cases, positive feedback occurs when the biological action of the hormone causes additional secretion of the hormone. One example of positive feedback is the surge of luteinizing hormone (LH) that occurs as a result of the stimulatory effect of estrogen on the anterior pituitary before ovulation. The secreted LH then acts on the ovaries to stimulate additional secretion of estrogen, which in turn causes more secretion of LH. Eventually, LH reaches an appropriate concentration and typical negative feedback control of hormone secretion is then exerted.␣ Cyclical Variations Occur in Hormone Release. Su- perimposed on the negative and positive feedback control of hormone secretion are periodic variations in hormone release that are influenced by seasonal changes, various stages of development and aging, the diurnal (daily) cycle, and sleep. For example, the secretion of growth hormone is markedly increased during the early period of sleep but is reduced during the later stages of sleep. In many cases, these cyclical variations in hormone secretion are due to changes in activity of neural pathways involved in controlling hormone release. Oscillations of endocrine signaling are driven, in part by circadian clocks. As discussed in Chapter 59 (see Figure 59- 8), the suprachiasmatic nucleus (SCN) of the hypothalamus serves as a “master clock” that controls rhythmic patterns of biological clocks in many parts of the body, including neuroendocrine cells and endocrine glands. There is also evidence for local clock control in peripheral endocrine tissues, such as the adrenal gland and pancreas, which have cyclical changes in their sensitivity to various signals. Cyclical changes in tissue responsiveness and fluctuations in hormone concentrations provide important mechanisms for the body to anticipate and adapt to the dramatic changes in stresses and demands that normally occur over the course of a day— ranging from restful sleep to eating meals and high levels of mental and physical activity. Rhythmic changes in female sex hormones that occur with an average 28-day cycle are also essential for reproduction, as discussed in Chapter 82.␣ TRANSPORT OF HORMONES IN THE BLOOD Water-soluble hormones (peptides and catecholamines) are dissolved in the plasma and transported from their sites of synthesis to target tissues, where they diffuse out 919 UNIT XIV Hormone Secretion After a Stimulus and Duration of Action of Different Hormones. Some hor- feedback signals to the endocrine gland become powerful enough to slow further synthesis and secretion of the hormone.␣ UNIT XIV Endocrinology and Reproduction of the capillaries, into the interstitial fluid, and ultimately to target cells. Steroid and thyroid hormones, in contrast, circulate in the blood while being mainly bound to plasma proteins. Usually less than 10% of steroid or thyroid hormones in the plasma exist free in solution. For example, more than 99% of the thyroxine in the blood is bound to plasma proteins. However, protein-bound hormones cannot easily diffuse across the capillaries and gain access to their target cells and are therefore biologically inactive until they dissociate from plasma proteins. The relatively large amounts of hormones bound to proteins serve as reservoirs, replenishing the concentration of free hormones when they are bound to target receptors or lost from the circulation. Binding of hormones to plasma proteins greatly slows their clearance from the plasma. “Clearance” of Hormones From the Blood. Two fac- tors can increase or decrease the concentration of a hormone in the blood: (1) the rate of hormone secretion into the blood; (2) the rate of removal of the hormone from the blood, which is called the metabolic clearance rate and is usually expressed in terms of the number of milliliters of plasma cleared of the hormone per minute. To calculate this clearance rate, one measures (1) the rate of disappearance of the hormone from the plasma (e.g., nanograms per minute) and (2) the plasma concentration of the hormone (e.g., nanograms per milliliter of plasma). Then, the metabolic clearance rate is calculated with use of the following formula: Metabolic clearance rate = Rate of disappearance of hormone from the plasma/Concentration of hormone The usual procedure for making this measurement is as follows. A purified solution of the hormone to be measured is tagged with a radioactive substance. Then the radioactive hormone is infused at a constant rate into the blood stream until the radioactive concentration in the plasma becomes steady. At this time, the disappearance rate of the radioactive hormone from the plasma equals the rate at which it is infused, which gives one the rate of disappearance. At the same time, the plasma concentration of the radioactive hormone is measured using a standard radioactive counting procedure. Then, using the formula just cited, the metabolic clearance rate is calculated. Hormones are “cleared” from the plasma in several ways, including (1) metabolic destruction by the tissues, (2) binding with the tissues, (3) excretion by the liver into the bile, and (4) excretion by the kidneys into the urine. For certain hormones, a decreased metabolic clearance rate may cause an excessively high concentration of the hormone in the circulating body fluids. For example, excessive steroid hormones accumulate when the liver is 920 diseased because these hormones are conjugated mainly in the liver and then “cleared” into the bile. Hormones are sometimes degraded at their target cells by enzymatic processes that cause endocytosis of the cell membrane hormone-receptor complex; the hormone is then metabolized in the cell, and the receptors are usually recycled back to the cell membrane. Most of the peptide hormones and catecholamines are water soluble and circulate freely in the blood. They are usually degraded by enzymes in the blood and tissues and rapidly excreted by the kidneys and liver, thus remaining in the blood for only a short time. For example, the halflife of angiotensin II circulating in the blood is less than a minute. Hormones that are bound to plasma proteins are cleared from the blood at much slower rates and may remain in the circulation for several hours or even days. The half-life of adrenal steroids in the circulation, for example, ranges between 20 and 100 minutes, whereas the half-life of the protein-bound thyroid hormones may be as long as 1 to 6 days.␣ MECHANISMS OF ACTION OF HORMONES HORMONE RECEPTORS AND THEIR ACTIVATION The first step of a hormone’s action is to bind to specific receptors at the target cell. Cells that lack receptors for the hormones do not respond. Receptors for some hormones are located on the target cell membrane, whereas other hormone receptors are located in the cytoplasm or the nucleus. When the hormone combines with its receptor, this action usually initiates a cascade of reactions in the cell, with each stage becoming more powerfully activated so that even small concentrations of the hormone can have a large effect. Hormone receptors are large proteins, and each cell that is to be stimulated usually has some 2000 to 100,000 receptors. Also, each receptor is usually highly specific for a single hormone, which determines the type of hormone that will act on a particular tissue. The target tissues that are affected by a hormone are those that contain its specific receptors. The locations for the different types of hormone receptors are generally the following: 1. In or on the surface of the cell membrane. The membrane receptors are specific mostly for the protein, peptide, and catecholamine hormones. 2. In the cell cytoplasm. The primary receptors for the different steroid hormones are found mainly in the cytoplasm. 3. In the cell nucleus. The receptors for the thyroid hormones are found in the nucleus and are believed to be located in direct association with one or more of the chromosomes. Chapter 75 Introduction to Endocrinology The Number and Sensitivity of Hormone Receptors Are Regulated. The number of receptors in a target cell INTRACELLULAR SIGNALING AFTER HORMONE RECEPTOR ACTIVATION Almost without exception, a hormone affects its target tissues by first forming a hormone-receptor complex. Formation of this complex alters the function of the receptor, and the activated receptor initiates the hormonal effects. To explain this process, let us give a few examples of the different types of interactions. rotransmitter substances, such as acetylcholine and norepinephrine, combine with receptors in the postsynaptic membrane. This combination almost always causes a change in the structure of the receptor, usually opening or closing a channel for one or more ions. Some of these ion channel–linked receptors open (or close) channels for sodium ions, others for potassium ions, others for calcium ions, and so forth. The altered movement of these ions through the channels causes the subsequent effects on the postsynaptic cells. Although a few hormones may exert some of their actions through activation of ion channel receptors, most hormones that open or close ions channels do this indirectly by coupling with G protein–linked or enzyme-linked receptors, as discussed next.␣ G Protein–Linked Hormone Receptors. Many hor- mones activate receptors that indirectly regulate the activity of target proteins (e.g., enzymes or ion channels) by coupling with groups of cell membrane proteins called heterotrimeric guanosine triphosphate (GTP)-binding proteins (G proteins) (Figure 75-4). All of the known G protein–coupled receptors (∼1000) have seven transmembrane segments that loop in and out of the cell membrane. Some parts of the receptor that protrude into the cell cytoplasm (especially the cytoplasmic tail of the receptor) are coupled to G proteins that include three (i.e., trimeric) parts—the α, β, and γ subunits. When the ligand (hormone) binds to the extracellular part of the receptor, a conformational change occurs in the receptor that activates the G proteins and induces intracellular signals that either (1) open or close cell membrane ion channels, (2) change the activity of an enzyme in the cytoplasm of the cell, or (3) activate gene transcription. The trimeric G proteins are named for their ability to bind guanosine nucleotides. In their inactive state, the α, β, and γ subunits of G proteins form a complex that binds guanosine diphosphate (GDP) on the α subunit. Hormone Receptor Extracellular fluid γ β γ α γ GDP G protein (inactive) β β α G protein (active) GTP Cytoplasm α GTP-activated target protein (enzyme) Figure 75-4. Mechanism of activation of a G protein–coupled receptor. When the hormone activates the receptor, the inactive α, β, and γ G protein complex associates with the receptor and is activated, with an exchange of guanosine triphosphate (GTP) for guanosine diphosphate (GDP). This process causes the α subunit (to which the GTP is bound) to dissociate from the β and γ subunits of the G protein and to interact with membrane-bound target proteins (enzymes) that initiate intracellular signals. 921 UNIT XIV usually does not remain constant from day to day or even from minute to minute. Receptor proteins are often inactivated or destroyed during the course of their function, and at other times they are reactivated or new ones are manufactured by the cell. For example, increased hormone concentration and increased binding with its target cell receptors sometimes cause the number of active receptors to decrease. This down-regulation of the receptors can occur as a result of (1) inactivation of some of the receptor molecules; (2) inactivation of some of the intracellular protein signaling molecules; (3) temporary sequestration of the receptor to the inside of the cell, away from the site of action of hormones that interact with cell membrane receptors; (4) destruction of the receptors by lysosomes after they are internalized; or (5) decreased production of the receptors. In each case, receptor downregulation decreases the target tissue’s responsiveness to the hormone. Some hormones cause up-regulation of receptors and intracellular signaling proteins; that is, the stimulating hormone induces greater than normal formation of receptor or intracellular signaling molecules by the target cell or greater availability of the receptor for interaction with the hormone. When up-regulation occurs, the target tissue becomes progressively more sensitive to the stimulating effects of the hormone.␣ Ion Channel–Linked Receptors. Virtually all the neu- UNIT XIV Endocrinology and Reproduction Enzyme-Linked Hormone Receptors. Some receptors, Fibroblast growth factor Growth hormone Hepatocyte growth factor Insulin Insulin-like growth factor-1 Leptin Prolactin Vascular endothelial growth factor 922 Leptin receptor P Activation of enzymes JAK2 Y Y STAT3 P Y Y P Y Y JAK2 P P STAT3 P P Physiological effects P STAT3 Translation mRNA P Table 75-2 Hormones That Use Receptor Tyrosine Kinase Signaling Leptin P when activated, function directly as enzymes or are closely associated with enzymes that they activate. These enzymelinked receptors are proteins that pass through the membrane only once, in contrast to the seven-transmembrane G protein–coupled receptors. Enzyme-linked receptors have their hormone-binding site on the outside of the cell membrane and their catalytic or enzyme-binding site on the inside. When the hormone binds to the extracellular part of the receptor, an enzyme immediately inside the cell membrane is activated (or occasionally inactivated). Although many enzyme-linked receptors have intrinsic enzyme activity, others rely on enzymes that are closely associated with the receptor to produce changes in cell function. Table 75-2 lists a few of the many peptide growth factors, cytokines, and hormones that use the enzyme-linked receptor tyrosine kinases for cell signaling. One example of an enzyme-linked receptor is the leptin receptor (Figure 75-5). Leptin is a hormone secreted by fat cells and has many physiological effects, but it is especially important in regulating appetite and energy balance, as discussed in Chapter 72. The leptin receptor is a member of a large family of cytokine receptors that do not themselves contain enzymatic activity but signal through associated enzymes. In the case of the leptin receptor, one of the signaling pathways occurs through a tyrosine kinase of the janus kinase (JAK) family, JAK2. The leptin receptor exists as a dimer (i.e., in two parts), and binding of leptin to the extracellular part of the receptor alters its conformation, enabling phosphorylation and activation of the intracellular associated JAK2 molecules. The activated JAK2 molecules then phosphorylate other tyrosine residues within the leptin receptor–JAK2 complex to mediate intracellular signaling. The intracellular signals include phosphorylation of signal transducer and activator of transcription (STAT) proteins, which activates transcription by leptin target genes to initiate protein synthesis. Phosphorylation of JAK2 also leads to activation of other intracellular enzyme pathways such as mitogen-activated protein kinases (MAPK) and phosphatidylinositol 3-kinase (PI3K). Some of the effects of leptin occur rapidly as a result of activation of these intracellular enzymes, whereas other actions occur more slowly and require synthesis of new proteins. Another example, one widely used in hormonal control of cell function, is for the hormone to bind with a ST AT 3 ST AT 3 When the receptor is activated, it undergoes a conformational change that causes the GDP-bound trimeric G protein to associate with the cytoplasmic part of the receptor and to exchange GDP for GTP. Displacement of GDP by GTP causes the α subunit to dissociate from the trimeric complex and to associate with other intracellular signaling proteins; these proteins, in turn, alter the activity of ion channels or intracellular enzymes such as adenylyl cyclase or phospholipase C, which alter cell function. The signaling event is terminated when the hormone is removed and the α subunit inactivates itself by converting its bound GTP to GDP; then the α subunit once again combines with the β and γ subunits to form an inactive, membrane-bound trimeric G protein. Additional details of G protein signaling are discussed in Chapter 46 and shown in Figure 46-7. Some hormones are coupled to inhibitory G proteins (denoted as Gi proteins), whereas others are coupled to stimulatory G proteins (denoted as Gs proteins). Thus, depending on the coupling of a hormone receptor to an inhibitory or stimulatory G protein, a hormone can either increase or decrease the activity of intracellular enzymes. This complex system of cell membrane G proteins provides a vast array of potential cell responses to different hormones in the various target tissues of the body.␣ STAT3 Target gene Figure 75-5. An enzyme-linked receptor—the leptin receptor. The receptor exists as a homodimer (two identical parts), and leptin binds to the extracellular part of the receptor, causing phosphorylation (P) and activation of the intracellular associated janus kinase 2 (JAK2). This mechanism causes phosphorylation of signal transducer and activator of transcription (STAT) proteins, which then activates the transcription of target genes and the synthesis of proteins. JAK2 phosphorylation also activates several other enzyme systems that mediate some of the more rapid effects of leptin. Y, Specific tyrosine phosphorylation sites. Chapter 75 Introduction to Endocrinology Intracellular Hormone Receptors and Activation of Genes. Several hormones, including adrenal and gonadal steroid hormones, thyroid hormones, retinoid hormones, and vitamin D, bind with protein receptors inside the cell rather than in the cell membrane. Because these hormones are lipid soluble, they readily cross the cell membrane and interact with receptors in the cytoplasm or nucleus. The activated hormone-receptor complex then binds with a specific regulatory (promoter) sequence of the DNA called the hormone response element, and in this manner either activates or represses transcription of specific genes and formation of messenger RNA (mRNA; Figure 75-6). Therefore, minutes, hours, or even days after the hormone has entered the cell, newly formed proteins appear in the cell and become the controllers of new or altered cellular functions. Many different tissues have identical intracellular hormone receptors, but the genes that the receptors regulate are different in the various tissues. An intracellular receptor can activate a gene response only if the appropriate combination of gene regulatory proteins is present, and many of these regulatory proteins are tissue specific. Thus, the responses of different tissues to a hormone are determined not only by the specificity of the receptors but also by the expression of genes that the receptor regulates.␣ SECOND MESSENGER MECHANISMS FOR MEDIATING INTRACELLULAR HORMONAL FUNCTIONS We noted earlier that one way that hormones exert intracellular actions is to stimulate formation of the second messenger cAMP inside the cell membrane. The cAMP then causes subsequent intracellular effects of the hormone. Thus, the only direct effect that the hormone has on the cell is to activate a single type of membrane receptor. The second messenger does the rest. cAMP is not the only second messenger used by the different hormones. Two other especially important ones are (1) calcium ions and associated calmodulin and (2) products of membrane phospholipid breakdown. In some cases, a hormone may stimulate more than one second messenger system in the same target tissue. Adenylyl Cyclase–cAMP Second Messenger System Table 75-3 shows a few of the many hormones that use the adenylyl cyclase–cAMP mechanism to stimulate their target tissues, and Figure 75-7 shows the adenylyl cyclase–cAMP second messenger system. Binding of the Lipophilic hormone Extracellular fluid Diffusion Target cell Nucleus Proteins DNA Nuclear receptor Ribosome Cytoplasmic receptor Hormone receptor complex mRNA Hormone response element mRNA Nuclear envelope Nuclear pore Figure 75-6. Mechanisms of interaction of lipophilic hormones, such as steroids, with intracellular receptors in target cells. After the hormone binds to the receptor in the cytoplasm or in the nucleus, the hormone-receptor complex binds to the hormone response element (promoter) on the DNA. This action either activates or inhibits gene transcription, formation of messenger RNA (mRNA), and protein synthesis. 923 UNIT XIV special transmembrane receptor, which then becomes the activated enzyme adenylyl cyclase at the end that protrudes to the interior of the cell. This cyclase catalyzes the formation of cAMP, which has a multitude of effects inside the cell to control cell activity, as discussed later. cAMP is called a second messenger because it is not the hormone itself that directly institutes the intracellular changes; instead, the cAMP serves as a second messenger to cause these effects. For a few peptide hormones, such as atrial natriuretic peptide, cyclic guanosine monophosphate, which is only slightly different from cAMP, serves in a similar manner as a second messenger.␣ UNIT XIV Endocrinology and Reproduction Table 75-3 Hormones That Use the Adenylyl Cyclase– cAMP Second Messenger System Angiotensin II (vascular smooth muscle) Catecholamines (α receptors) Gonadotropin-releasing hormone (GnRH) Growth hormone–releasing hormone (GHRH) Parathyroid hormone (PTH) Oxytocin Thyrotropin-releasing hormone (TRH) Vasopressin (V1 receptor, vascular smooth muscle) Adrenocorticotropic hormone (ACTH) Angiotensin II (epithelial cells) Calcitonin Catecholamines (beta receptors) Corticotropin-releasing hormone (CRH) Follicle-stimulating hormone (FSH) Glucagon Growth hormone–releasing hormone (GHRH) Human chorionic gonadotropin (hCG) Luteinizing hormone (LH) Parathyroid hormone (PTH) Secretin Somatostatin Thyroid-stimulating hormone (TSH) Vasopressin (V2 receptor, epithelial cells) cAMP, Cyclic adenosine monophosphate. Extracellular fluid Hormone γ Cytoplasm β α GTP cAMP Active cAMPdependent protein kinase Protein – PO4 + ADP Adenylyl cyclase ATP Inactive cAMPdependent protein kinase Protein + ATP Cell’s response Figure 75-7. The cyclic adenosine monophosphate (cAMP) mechanism by which many hormones exert their control of cell function. ADP, Adenosine diphosphate; ATP, adenosine triphosphate; GTP, guanosine triphosphate. hormones with the receptor allows coupling of the receptor to a G protein. If the G protein stimulates the adenylyl cyclase–cAMP system, it is called a Gs protein, denoting a stimulatory G protein. Stimulation of adenylyl cyclase, a membrane-bound enzyme, by the Gs protein then catalyzes the conversion of a small amount of cytoplasmic adenosine triphosphate into cAMP inside the cell. This then activates cAMP-dependent protein kinase, which phosphorylates specific cell proteins, triggering biochemical reactions that ultimately lead to the cell’s response to the hormone. 924 Table 75-4 Hormones That Use the Phospholipase C Second Messenger System Once cAMP is formed inside the cell, it usually activates a cascade of enzymes. That is, first one enzyme is activated, which activates a second enzyme, which activates a third, and so forth. The importance of this mechanism is that only a few molecules of activated adenylyl cyclase immediately inside the cell membrane can cause many more molecules of the next enzyme to be activated, which can cause still more molecules of the third enzyme to be activated, and so forth. In this way, even the slightest amount of hormone acting on the cell surface can initiate a powerful cascading activating force for the entire cell. If binding of the hormone to its receptors is coupled to an inhibitory G protein (denoted Gi protein), adenylyl cyclase will be inhibited, reducing formation of cAMP and ultimately leading to an inhibitory action in the cell. Thus, depending on the coupling of the hormone receptor to an inhibitory or a stimulatory G protein, a hormone can either increase or decrease the concentration of cAMP and phosphorylation of key proteins inside the cell. The specific action that occurs in response to increases or decreases of cAMP in each type of target cell depends on the nature of the intracellular machinery; some cells have one set of enzymes, and other cells have other enzymes. Therefore, different functions are elicited in different target cells, such as initiating synthesis of specific intracellular chemicals, causing muscle contraction or relaxation, initiating secretion by the cells, and altering cell permeability. Thus, a thyroid cell stimulated by cAMP forms the metabolic hormones thyroxine and triiodothyronine, whereas the same cAMP in an adrenocortical cell causes secretion of the adrenocortical steroid hormones. In some epithelial cells of the distal and collecting tubules of the kidney, cAMP increases their permeability to water.␣ Cell Membrane Phospholipid Second Messenger System Some hormones activate transmembrane receptors that activate the enzyme phospholipase C attached to the inside projections of the receptors (Table 75-4). This enzyme catalyzes the breakdown of some phospholipids in the cell membrane, especially phosphatidylinositol biphosphate (PIP2), into two different second messenger products: inositol triphosphate (IP3) and diacylglycerol (DAG). The IP3 mobilizes calcium ions from mitochondria and the endoplasmic reticulum, and the calcium ions Chapter 75 Introduction to Endocrinology Extracellular fluid Receptor G protein Cell membrane Phospholipase C DAG + IP3 PIP2 HORMONES THAT ACT MAINLY ON THE GENETIC MACHINERY OF THE CELL Cytoplasm Active protein kinase C Protein – PO4 Steroid Hormones Increase Protein Synthesis Inactive protein kinase C Ca2+ Protein Endoplasmic reticulum Cell’s response Cell’s response Figure 75-8. The cell membrane phospholipid second messenger system by which some hormones exert their control of cell function. DAG, Diacylglycerol; IP3, inositol triphosphate; PIP2, phosphatidylinositol biphosphate. then have their own second messenger effects, such as smooth muscle contraction and changes in cell secretion. DAG, the other lipid second messenger, activates the enzyme protein kinase C, which then phosphorylates a large number of proteins, leading to the cell’s response (Figure 75-8). In addition to these effects, the lipid portion of DAG is arachidonic acid, which is the precursor for the prostaglandins and other local hormones that cause multiple effects in tissues throughout the body.␣ Calcium-Calmodulin Second Messenger System Another second messenger system operates in response to entry of calcium into the cells. Calcium entry may be initiated by (1) changes in membrane potential that open calcium channels or (2) a hormone interacting with membrane receptors that open calcium channels. Upon entering a cell, calcium ions bind with the protein calmodulin. This protein has four calcium sites, and when three or four of these sites have become bound with calcium, the calmodulin changes its shape and initiates multiple effects inside the cell, including activation or inhibition of protein kinases. Activation of calmodulindependent protein kinases causes, via phosphorylation, activation or inhibition of proteins involved in the cell’s response to the hormone. For example, one specific function of calmodulin is to activate myosin light chain kinase, Another means by which hormones act, especially steroid hormones, is to cause protein synthesis in target cells. These proteins then function as enzymes, transport proteins, or structural proteins, which in turn provide other functions of the cells. The sequence of events in steroid function is essentially the following (see Figure 75-6): 1. The steroid hormone diffuses across the cell membrane and enters the cytoplasm of the cell, where it binds with a specific receptor protein. 2. The combined receptor protein–hormone then diffuses into or is transported into the nucleus. 3. The combination binds at specific points on the DNA strands in the chromosomes, which activates the transcription process of specific genes to form mRNA. 4. The mRNA diffuses into the cytoplasm, where it promotes the translation process at the ribosomes to form new proteins. To give an example, aldosterone, one of the hormones secreted by the adrenal cortex, enters the cytoplasm of renal tubular cells, which contain a specific receptor protein often called the mineralocorticoid receptor. Therefore, in these cells, the sequence of events cited earlier ensues. After about 45 minutes, proteins begin to appear in the renal tubular cells and promote sodium reabsorption from the tubules and potassium secretion into the tubules. Thus, the full action of the steroid hormone is characteristically delayed for at least 45 minutes—up to several hours or even days. This action is in marked contrast to the rapid actions of some of the peptide and amino acid–derived hormones, such as vasopressin and norepinephrine.␣ Thyroid Hormones Increase Gene Transcription in the Cell Nucleus The thyroid hormones thyroxine and triiodothyronine cause increased transcription by specific genes in the nucleus. To 925 UNIT XIV which acts directly on the myosin of smooth muscle to cause smooth muscle contraction (see Figure 8-3). The normal calcium ion concentration in most cells of the body is 10−8 to 10−7 mol/L, which is not enough to activate the calmodulin system. However, when the calcium ion concentration rises to 10−6 to 10−5 mol/L, enough binding occurs to cause all the intracellular actions of calmodulin. This is almost exactly the same amount of calcium ion change in skeletal muscle that is required to activate troponin C, which causes skeletal muscle contraction, as explained in Chapter 7. It is interesting that troponin C is similar to calmodulin in both function and protein structure.␣ Peptide hormone UNIT XIV Endocrinology and Reproduction Measurement of Hormone Concentrations in the Blood 100 90 Percent of antibody bound with radioactive aldosterone accomplish this increased transcription, these hormones first bind directly with receptor proteins in the nucleus, as discussed in more detail in Chapter 77 (see Figure 77-5); these receptors are activated transcription factors located within the chromosomal complex, and they control the function of the gene promoters, as explained in Chapter 3. The following two features of thyroid hormone function in the nucleus are important: 1. They activate the genetic mechanisms for synthesizing many types of intracellular proteins—probably 100 or more. Many of these intracellular proteins are enzymes that promote enhanced intracellular metabolic activity in virtually all cells of the body. 2. Once bound to the intranuclear receptors, the thyroid hormones can continue to express their control functions for days or even weeks.␣ 80 70 60 50 40 30 20 10 0 2 4 8 16 32 64 128 Aldosterone concentration in test sample (ng/dl) Figure 75-9. Standard curve for radioimmunoassay of aldosterone. (Courtesy Dr. Manis Smith.) Most hormones are present in the blood in extremely minute quantities; some concentrations are as low as one billionth of a milligram (1 picogram) per milliliter. Therefore, it was difficult to measure these concentrations by the usual chemical means. However, an extremely sensitive method, called radioimmunoassay, was developed by Rosalyn Yalow and Solomon Berson in 1959 and revolutionized the measurement of hormones, their precursors, and their metabolic end products. More recently, additional methods, such as enzyme-linked immunosorbent assays, have been developed for accurate, high-throughput measurements of hormones. Fourth, to make the assay highly quantitative, the radioimmunoassay procedure is also performed for “standard” solutions of untagged hormone at several concentration levels. Then a “standard curve” is plotted, as shown in Figure 75-9. By comparing the radioactive counts recorded from the “unknown” assay procedures with the standard curve, one can determine within an error of 10% to 15%, the concentration of the hormone in the “unknown” assayed fluid. As little as billionths or even trillionths of a gram of hormone can often be assayed in this way.␣ Radioimmunoassay Enzyme-Linked Immunosorbent Assay The method of performing radioimmunoassay is as follows. First, an antibody that is highly specific for the hormone to be measured is produced. Second, a small quantity of this antibody is (1) mixed with a quantity of fluid from the animal containing the hormone to be measured and (2) mixed simultaneously with an appropriate amount of purified standard hormone that has been tagged with a radioactive isotope. However, one specific condition must be met. There must be too little antibody to bind completely both the radioactively tagged hormone and the hormone in the fluid to be assayed. Therefore, the natural hormone in the assay fluid and the radioactive standard hormone compete for binding sites of the antibody. In the process of competing, the quantity of each of the two hormones, the natural and the radioactive, that binds is proportional to its concentration in the assay fluid. Third, after binding has reached equilibrium, the antibodyhormone complex is separated from the remainder of the solution, and the quantity of radioactive hormone bound in this complex is measured by radioactive counting techniques. If a large amount of radioactive hormone has bound with the antibody, it is clear that there was only a small amount of natural hormone to compete with the radioactive hormone, and therefore the concentration of the natural hormone in the assayed fluid was small. Conversely, if only a small amount of radioactive hormone has bound, it is clear that there was a large amount of natural hormone to compete for the binding sites. Enzyme-linked immunosorbent assays (ELISAs) can be used to measure almost any protein, including hormones. This test combines the specificity of antibodies with the sensitivity of simple enzyme assays. Figure 75-10 shows the basic elements of this method, which is often performed on plastic plates that each have 96 small wells. Each well is coated with an antibody (AB1) that is specific for the hormone being assayed. Samples or standards are added to each of the wells, followed by a second antibody (AB2) that is also specific for the hormone but binds to a different site of the hormone molecule. A third antibody (AB3) that is added recognizes AB2 and is coupled to an enzyme that converts a suitable substrate to a product that can be easily detected by colorimetric or fluorescent optical methods. Because each molecule of enzyme catalyzes the formation of many thousands of product molecules, even small amounts of hormone molecules can be detected. In contrast to competitive radioimmunoassay methods, ELISA methods use excess antibodies so that all hormone molecules are captured in antibody-hormone complexes. Therefore, the amount of hormone present in the sample or in the standard is proportional to the amount of product formed. The ELISA method has become widely used in clinical and research laboratories because (1) it does not use radioactive isotopes, (2) much of the assay can be automated using 96-well plates, and (3) it has proved to be a costeffective and accurate method for assessing hormone levels. 926 Chapter 75 Introduction to Endocrinology S S S P S P P P P E AB3 AB2 H AB1 Figure 75-10. Basic principles of the enzyme-linked immunosorbent assay for measuring the concentration of a hormone (H). AB1 and AB2 are antibodies that recognize the hormone at different binding sites, and AB3 is an antibody that recognizes AB2. E is an enzyme linked to AB3 that catalyzes the formation of a colored fluorescent product (P) from a substrate (S). The amount of the product is measured using optical methods and is proportional to the amount of hormone in the well if there are excess antibodies in the well. Bibliography Aguiar-Oliveira MH, Bartke A: Growth hormone deficiency: health and longevity. Endocr Rev 40:575, 2019. Baker ME: Steroid receptors and vertebrate evolution. Mol Cell Endocrinol Oct 1;496:110526. doi: 10.1016/j.mce.2019.110526 Bianco AC, Dumitrescu A, Gereben B, et al: Paradigms of dynamic control of thyroid hormone signaling. Endocr Rev 40:1000, 2019. 927 UNIT XIV S P S Deussing JM, Chen A: The corticotropin-releasing factor family: physiology of the stress response. Physiol Rev 98:2225, 2018. Forrester SJ, Booz GW, Sigmund CD, et al: Angiotensin II signal transduction: an update on mechanisms of physiology and pathophysiology. Physiol Rev 98:1627, 2018. Gamble KL, Berry R, Frank SJ, Young ME: Circadian clock control of endocrine factors. Nat Rev Endocrinol 10:466, 2014. Gomez-Sanchez E, Gomez-Sanchez CE: The multifaceted mineralocorticoid receptor. Compr Physiol 4:965, 2014. Haeusler RA, McGraw TE, Accili D: Biochemical and cellular properties of insulin receptor signalling. Nat Rev Mol Cell Biol 19:31, 2018. Harno E, Gali Ramamoorthy T, Coll AP, White A: POMC: the physiological power of hormone processing. Physiol Rev 98:2381, 2018. Hewitt SC, Korach KS: Estrogen receptors: new directions in the new millennium. Endocr Rev 39:664, 2018. Hunter I, Hay CW, Esswein B, Watt K, McEwan IJ: Tissue control of androgen action: the ups and downs of androgen receptor expression. Mol Cell Endocrinol 465:27, 2018. Jurek B, Neumann ID: The oxytocin receptor: from intracellular signaling to behavior. Physiol Rev 98:1805, 2018. Najjar SM, Perdomo G: Hepatic insulin clearance: mechanism and physiology. Physiology (Bethesda) 34:198, 2019. Oster H, Challet E, Ott V, et al: The functional and clinical significance of the 24-hour rhythm of circulating glucocorticoids. Endocr Rev 38:3, 2017. Pan WW, Myers MG Jr: Leptin and the maintenance of elevated body weight. Nat Rev Neurosci 19:95, 2018. Petersen MC, Shulman GI: Mechanisms of insulin action and insulin resistance. Physiol Rev 98:2133, 2018. Sarfstein R, Werner H: Minireview: nuclear insulin and insulin-like growth factor-1 receptors: a novel paradigm in signal transduction. Endocrinology 154:1672, 2013. Stenvers DJ, Scheer FAJL, Schrauwen P, et al: Circadian clocks and insulin resistance. Nat Rev Endocrinol 15:75, 2019. Yang Q, Vijayakumar A, Kahn BB: Metabolites as regulators of insulin sensitivity and metabolism. Nat Rev Mol Cell Biol 19:654, 2018. CHAPTER 76 PITUITARY GLAND AND ITS RELATION TO THE HYPOTHALAMUS ANTERIOR AND POSTERIOR LOBES OF THE PITUITARY GLAND The pituitary gland (Figure 76-1), also called the hypophysis, is a small gland—about 1 centimeter in diameter and 0.5 to 1 gram in weight—that lies in the sella turcica, a bony cavity at the base of the brain—and is connected to the hypothalamus by the pituitary (or hypophysial) stalk. Physiologically, the pituitary gland is divisible into two distinct portions: the anterior pituitary, also known as the adenohypophysis, and the posterior pituitary, also known as the neurohypophysis. Between these portions is a small, relatively avascular zone called the pars intermedia, which is much less developed in humans but is larger and much more functional in some animals. Embryologically, the two portions of the pituitary originate from different sources—the anterior pituitary from Rathke’s pouch, which is an embryonic invagination of the pharyngeal epithelium, and the posterior pituitary from a neural tissue outgrowth from the hypothalamus. The origin of the anterior pituitary from the pharyngeal epithelium explains the epithelioid nature of its cells, and the origin of the posterior pituitary from neural tissue explains the presence of large numbers of glial-type cells in this gland. Six major peptide hormones plus several other hormones of lesser known importance are secreted by the anterior pituitary, and two important peptide hormones are secreted by the posterior pituitary. The hormones of the anterior pituitary play major roles in the control of metabolic functions throughout the body, as shown in Figure 76-2. Growth hormone promotes growth of the entire body by affecting protein formation, cell multiplication, and cell differentiation. Adrenocorticotropic hormone (corticotropin) controls the secretion of some of the adrenocortical hormones, which affect metabolism of glucose, proteins, and fats. Thyroid-stimulating hormone (thyrotropin) controls the secretion rate of thyroxine and triiodothyronine UNIT XIV Pituitary Hormones and Their Control by the Hypothalamus by the thyroid gland, and these hormones control the rates of most intracellular chemical reactions in the body. Prolactin promotes mammary gland development and milk production. folliclestimulating hormone and luteinizing hormone, control growth of the ovaries and testes, as well as their hormonal and reproductive activities. The two hormones secreted by the posterior pituitary play other roles. Antidiuretic hormone (also called vasopressin) controls the rate of water excretion into the urine, thus helping to control water concentration in the body fluids. Oxytocin helps express milk from the glands of the breast to the nipples during suckling and helps in delivery of the baby at the end of gestation. The Anterior Pituitary Gland Contains Several Different Cell Types That Synthesize and Secrete Hormones. Usually, there is one cell type for each ma- jor hormone formed in the anterior pituitary gland. With Hypothalamus Optic chiasm Mammillary body Hypophysial stalk Anterior pituitary Posterior pituitary Pars intermedia Figure 76-1. Pituitary gland. 929 These proofs may contain color figures. Those figures may print black and white in the final printed book if a color print product has not been planned. The color figures will appear in color in all electronic versions of this book. UNIT XIV Endocrinology and Reproduction Gamma ( γ ) cell Growth hormone Thyrotropin Thyroid gland Anterior pituitary gland Alpha (α) cell Epsilon ( ε ) Delta (δ ) acidophil cell basophil cell Increases blood glucose level ACH Corticotropin Follicle stimulating Sinusoid Promotes secretion of insulin Adrenal cortex Pancreas Luteinizing Ovary Beta (β) cell Prolactin Mammary gland Figure 76-2. Metabolic functions of the anterior pituitary hormones. ACH, Adrenal corticosteroid hormones. special stains attached to high-affinity antibodies that bind with the distinctive hormones, at least five cell types can be differentiated (Figure 76-3). Table 76-1 provides a summary of these cell types, the hormones they produce, and their physiological actions. These five cell types are as follows: 1. Somatotropes—human growth hormone (hGH) 2. Corticotropes—adrenocorticotropic hormone 3. Thyrotropes 4. Gonadotropes—gonadotropic hormones, which include both luteinizing hormone (LH) and folliclestimulating hormone (FSH) 5. Lactotropes—prolactin (PRL) About 30% to 40% of the anterior pituitary cells are somatotropes that secrete growth hormone (GH), and of the other cell types accounts for only 3% to 5% of the total; nevertheless, they secrete powerful hormones for controlling thyroid function, sexual functions, and milk secretion by the breasts. Somatotropes stain strongly with acid dyes and are therefore called acidophils. Thus, pituitary tumors that secrete large quantities of hGH are called acidophilic tumors.␣ Posterior Pituitary Hormones Are Synthesized by Cell Bodies in the Hypothalamus. The bodies of the cells that secrete the posterior pituitary hormones are not located in the pituitary gland but are large neurons, called magnocellular neurons, located in the supraoptic and paraventricular nu- Figure 76-3. Cellular structure of the anterior pituitary gland. (Modified from Guyton AC: Physiology of the Human Body, 6th ed. Philadelphia: Saunders College Publishing, 1984.) clei of the hypothalamus. The hormones are then transported in the axoplasm of the neuron’s nerve fibers passing from the hypothalamus to the posterior pituitary gland. This mechanism is discussed later in the chapter.␣ HYPOTHALAMUS CONTROLS PITUITARY SECRETION Almost all pituitary secretion is controlled by hormonal or nervous signals from the hypothalamus. Indeed, when the pituitary gland is removed from its normal position beneath the hypothalamus and transplanted to some other part of the body, its secretion rates of the different hormones (except for prolactin) fall to very low levels. Secretion from the posterior pituitary is controlled by nerve signals that originate in the hypothalamus and terminate in the posterior pituitary. In contrast, secretion by the anterior pituitary is controlled by hormones called hypothalamic releasing and hypothalamic inhibitory hormones (or factors) secreted within the hypothalamus and then conducted, as shown in Figure 76-4, to the anterior pituitary through minute blood vessels called hypothalamic-hypophysial portal vessels. In the anterior pituitary, these releasing and inhibitory hormones act on the glandular cells to control their secretion. This system of control is discussed in the next section of this chapter. The hypothalamus receives signals from many sources in the nervous system. Thus, when a person is exposed to pain, a portion of the pain signal is transmitted into the hypothalamus. Likewise, when a person experiences some powerful depressing or exciting thought, a portion of the signal is transmitted into the hypothalamus. Olfactory stimuli denoting pleasant or unpleasant smells transmit strong signals directly and through the amygdaloid nuclei 930 These proofs may contain color figures. Those figures may print black and white in the final printed book if a color print product has not been planned. The color figures will appear in color in all electronic versions of this book. Chapter 76 Pituitary Hormones and Their Control by the Hypothalamus Table 76-1 Cells and Hormones of the Anterior Pituitary Gland and Their Physiological Functions Hormone Chemistry Physiological Action Somatotropes Growth hormone (GH) (somatotropin) Single chain of 191 amino acids Stimulates body growth; stimulates secretion of insulin-like growth factor-1; stimulates lipolysis; inhibits actions of insulin on carbohydrate and lipid metabolism Corticotropes Adrenocorticotropic hormone (ACTH) (corticotropin) Single chain of 39 amino acids Stimulates production of glucocorticoids and androgens by the adrenal cortex; maintains size of zona fasciculata and zona reticularis of cortex Thyrotropes Thyroid-stimulating hormone (TSH) (thyrotropin) Glycoprotein of two subunits, α (89 amino acids) and β (112 amino acids) Stimulates production of thyroid hormones by thyroid follicular cells; maintains size of follicular cells Gonadotropes Follicle-stimulating hormone (FSH) Glycoprotein of two subunits, α (89 amino acids) and β (112 amino acids) Glycoprotein of two subunits, α (89 amino acids) and β (115 amino acids) Stimulates development of ovarian follicles; regulates spermatogenesis in the testis Single chain of 198 amino acids Stimulates milk secretion and production Luteinizing (LH) hormone Lactotropesmammotropes Prolactin (PRL) Hypothalamus Optic chiasm Mammillary body Median eminence Artery Anterior pituitary Primary capillary plexus Hypothalamichypophysial portal vessels Posterior pituitary Vein Sinuses Figure 76-4. Hypothalamic-hypophysial portal system. into the hypothalamus. Even the concentrations of nutrients, electrolytes, water, and various hormones in the blood excite or inhibit various portions of the hypothalamus. Thus, the hypothalamus is a center for integrating information concerning the internal well-being of the body, and much of this information is used to control secretions of the many globally important pituitary hormones. HYPOTHALAMIC-HYPOPHYSIAL PORTAL BLOOD VESSELS OF THE ANTERIOR PITUITARY GLAND The anterior pituitary is a highly vascular gland with extensive capillary sinuses among the glandular cells. UNIT XIV Cell Causes ovulation and formation of the corpus luteum in the ovary; stimulates production of estrogen and progesterone by the ovary; stimulates testosterone production by the testis Almost all the blood that enters these sinuses passes first through another capillary bed in the lower hypothalamus. The blood then flows through small hypothalamic-hypophysial portal blood vessels into the anterior pituitary sinuses. Figure 76-4 shows the lowermost portion of the hypothalamus, called the median eminence, which connects inferiorly with the pituitary stalk. The median eminence is the functional link between the hypothalamus and the anterior pituitary gland. Small arteries penetrate into the median eminence and then additional small vessels return to its surface, coalescing to form the hypothalamichypophysial portal blood vessels. These vessels pass downward along the pituitary stalk to supply blood to the anterior pituitary sinuses. Hypothalamic Releasing and Inhibitory Hormones Are Secreted Into the Median Eminence. Special neu- rons in the hypothalamus synthesize and secrete the hypothalamic releasing and inhibitory hormones that control secretion of the anterior pituitary hormones. These neurons originate in various parts of the hypothalamus and send their nerve fibers to the median eminence and tuber cinereum, an extension of hypothalamic tissue into the pituitary stalk. The endings of these fibers are different from most endings in the central nervous system in that their function is not to transmit signals from one neuron to another but rather to secrete the hypothalamic releasing and inhibitory hormones into the tissue fluids. These hormones are immediately absorbed into the hypothalamic-hypophysial portal system and carried directly to the sinuses of the anterior pituitary gland.␣ 931 These proofs may contain color figures. Those figures may print black and white in the final printed book if a color print product has not been planned. The color figures will appear in color in all electronic versions of this book. UNIT XIV Endocrinology and Reproduction Table 76-2 Hypothalamic Releasing and Inhibitory Hormones That Control Secretion of the Anterior Pituitary Gland Hormone Structure Primary Action on Anterior Pituitary Thyrotropin-releasing hormone (TRH) Peptide of 3 amino acids Stimulates secretion of TSH by thyrotropes Gonadotropin-releasing hormone (GnRH) Single chain of 10 amino acids Stimulates secretion of FSH and LH by gonadotropes Corticotropin-releasing hormone (CRH) Single chain of 41 amino acids Stimulates secretion of ACTH by corticotropes Growth hormone–releasing hormone (GHRH) Single chain of 44 amino acids Stimulates secretion of growth hormone by somatotropes Growth hormone inhibitory hormone (somatostatin) Single chain of 14 amino acids Inhibits secretion of growth hormone by somatotropes Prolactin-inhibiting hormone (PIH) Dopamine (a catecholamine) Inhibits synthesis and secretion of prolactin by lactotropes ACTH, Adrenocorticotropic hormone; FSH, follicle-stimulating hormone; LH, luteinizing hormone; TSH, thyroid-stimulating hormone. Hypothalamic Releasing and Inhibitory Hormones Control Anterior Pituitary Secretion. The function of the releasing and inhibitory hormones is to control secretion of the anterior pituitary hormones. For most of the anterior pituitary hormones, it is the releasing hormones that are important, but for prolactin, a hypothalamic inhibitory hormone probably exerts more control. The major hypothalamic releasing and inhibitory hormones, which are summarized in Table 76-2, are the following: 1. Thyrotropin-releasing hormone 2. Corticotropin-releasing hormone (CRH), which 3. Growth hormone–releasing hormone (GHRH), which causes release of GH, and growth hormone inhibitory hormone (GHIH), also called somatostatin, which inhibits release of GH 4. Gonadotropin-releasing hormone (GnRH), which causes release of the two gonadotropic hormones, LH and FSH 5. Prolactin inhibitory hormone (PIH), also known as dopamine, which causes inhibition of prolactin secretion Additional hypothalamic hormones include one that stimulates prolactin secretion and perhaps others that inhibit release of the anterior pituitary hormones. Each of the more important hypothalamic hormones is discussed in detail as the specific hormonal systems controlled by them are presented in this and subsequent chapters.␣ Specific Areas in the Hypothalamus Control Secretion of Specific Hypothalamic Releasing and Inhibitory Hormones. All or most of the hypothalamic hormones are secreted at nerve endings in the median eminence before being transported to the anterior pituitary gland. Electrical stimulation of this region excites these nerve endings and, therefore, causes release of essentially all the hypothalamic hormones. However, the neuronal cell bodies that give rise to these median eminence nerve endings are located in other discrete areas of the hypothalamus or in closely related areas of the basal brain.␣ PHYSIOLOGICAL FUNCTIONS OF GROWTH HORMONE All the major anterior pituitary hormones, except for GH, exert their principal effects mainly by stimulating target glands, including thyroid gland, adrenal cortex, ovaries, testicles, and mammary glands. The functions of each of these pituitary hormones are so intimately concerned with the functions of the respective target glands that, except for GH, their functions are discussed in subsequent chapters along with the target glands. GH, however, exerts its effects directly on all or almost all tissues of the body. GROWTH HORMONE PROMOTES GROWTH OF MANY BODY TISSUES GH, also called somatotropic hormone or somatotropin, is a small protein molecule that contains 191 amino acids in a single chain and has a molecular weight of 22,005. It causes growth of almost all tissues of the body that are capable of growing. It promotes increased sizes of the cells and increased mitosis, with development of greater numbers of cells and specific differentiation of certain types of cells such as bone growth cells and early muscle cells. Figure 76-5 shows typical weight charts of two growing littermate rats; one received daily injections of GH, and the other did not receive GH. This figure shows marked enhancement of growth in the rat given GH, in the early days of life and even after the two rats reached adulthood. In the early stages of development, all organs of the treated rat increased proportionately in size; after adulthood was reached, most of the bones stopped lengthening, but many of the soft tissues continued to grow. Once the epiphyses of the long bones have united with the shafts, further lengthening of bone cannot occur, even though many other tissues of the body can continue to grow throughout life.␣ 932 These proofs may contain color figures. Those figures may print black and white in the final printed book if a color print product has not been planned. The color figures will appear in color in all electronic versions of this book. Chapter 76 Pituitary Hormones and Their Control by the Hypothalamus Hypothalamus Injected daily with growth hormone 400 300 UNIT XIV Body weight (grams) 500 Control 200 GHRH neurons (+) SST neurons (–) 100 0 0 100 200 300 400 Days 500 600 Figure 76-5. Comparison of weight gain of a rat injected daily with growth hormone with that of a normal littermate. Growth hormone GROWTH HORMONE HAS SEVERAL METABOLIC EFFECTS Aside from its general effect in causing growth, GH has multiple specific metabolic effects (Figure 76-6), including the following: (1) increased rate of protein synthesis in most cells of the body; (2) increased mobilization of fatty acids from adipose tissue, increased free fatty acids in the blood, and increased use of fatty acids for energy; and (3) decreased rate of glucose utilization throughout the body. Thus, in effect, GH enhances body protein, decreases fat stores, and conserves carbohydrates. Growth Hormone Promotes Protein Deposition in Tissues Although the precise mechanisms by which growth hormone increases protein deposition are not fully understood, a series of different effects are known, all of which could lead to enhanced protein deposition. Enhancement of Amino Acid Transport Through the Cell Membranes. GH directly enhances transport of most amino acids through cell membranes to the interior of the cells. This increases amino acid concentrations in the cells and is presumed to be at least partly responsible for the increased protein synthesis. This control of amino acid transport is similar to the effect of insulin in controlling glucose transport through the membrane, as discussed in Chapters 68 and 79.␣ Enhancement of RNA Translation to Cause Protein Synthesis by the Ribosomes. Even when the amino acid concentrations are not increased in the cells, GH still increases RNA translation, causing protein to be synthesized in greater amounts by the ribosomes in the cytoplasm.␣ Increased Nuclear Transcription of DNA to Form RNA. Over more prolonged periods (24–48 hours), GH also stimulates transcription of DNA in the nucleus, causing formation of increased quantities of RNA. This promotes more Adipose tissue Glucose uptake Lipolysis Liver IGF-1 Bone Muscle Glucose uptake Amino acid uptake Protein synthesis Osteogenic and chondrocytic cells Amino acid uptake Protein synthesis DNA/RNA synthesis Collagen Cell size and number Figure 76-6. Effects of growth hormone and insulin-like growth factor -1 (IGF-1) on growth and metabolism. Growth hormone secretion is stimulated by growth hormone–releasing hormone (GHRH) and inhibited by somatostatin (SST), as well as negative feedback effects of growth hormone and IGF-1 on the anterior pituitary gland and the hypothalamic neurons. Other factors that control growth hormone secretion are described in Table 76-3. Table 76-3 Factors That Stimulate or Inhibit Secretion of Growth Hormone Stimulate Growth Hormone Secretion Inhibit Growth Hormone Secretion Decreased blood glucose level Decreased blood free fatty acid levels Increased blood amino acid levels (arginine) Starvation or fasting, protein deficiency Trauma, stress, excitement Exercise Testosterone, estrogen Deep sleep (stages 2 and 4) Growth hormone–releasing hormone Ghrelin Increased blood glucose level Increased blood free fatty acid levels Aging Obesity Growth hormone inhibitory hormone (somatostatin) Growth hormone (exogenous) Insulin-like growth factors (somatomedins) 933 These proofs may contain color figures. Those figures may print black and white in the final printed book if a color print product has not been planned. The color figures will appear in color in all electronic versions of this book. UNIT XIV Endocrinology and Reproduction protein synthesis and growth if sufficient energy, amino acids, vitamins, and other requisites for growth are available. In the long run, this may be the most important function of GH.␣ Decreased Catabolism of Protein and Amino Acids. In addition to the increase in protein synthesis, GH decreases breakdown of cell protein. A probable reason for this decrease is that GH also mobilizes large quantities of free fatty acids from the adipose tissue, and these are used to supply most of the energy for the body’s cells, thus acting as a potent “protein sparer.”␣ Summary. GH enhances almost all facets of amino acid uptake and protein synthesis by cells, while at the same time reducing the breakdown of proteins.␣ Growth Hormone Enhances Fat Utilization for Energy GH has a specific effect to cause release of fatty acids from adipose tissue and, therefore, increases the concentration of fatty acids in body fluids. Also, in tissues throughout the body, GH enhances conversion of fatty acids to acetyl coenzyme A (acetyl-CoA) and its subsequent utilization for energy. Therefore, under the influence of GH, fat is used for energy in preference to use of carbohydrates and proteins. The ability of GH to promote fat utilization, together with its protein anabolic effect, causes an increase in lean body mass. However, mobilization of fat by GH requires several hours to occur, whereas enhancement of protein synthesis can begin in minutes under the influence of GH. “Ketogenic” Effect of Excessive Growth Hormone. Under the influence of excessive amounts of GH, fat mobilization from adipose tissue sometimes becomes so great that large quantities of acetoacetic acid are formed by the liver and released into the body fluids, thus causing ketosis. This excessive mobilization of fat from the adipose tissue also frequently causes a fatty liver.␣ Growth Hormone Decreases Carbohydrate Utilization GH has multiple effects that influence carbohydrate metabolism, including (1) decreased glucose uptake in tissues such as skeletal muscle and fat, (2) increased glucose production by the liver, and (3) increased insulin secretion. Each of these changes results from GH-induced “insulin resistance,” which attenuates insulin’s actions to stimulate uptake and utilization of glucose in skeletal muscle and adipose tissue and to inhibit gluconeogenesis (glucose production) by the liver; this leads to increased blood glucose concentration and a compensatory increase in insulin secretion. For these reasons, GH’s effects are called diabetogenic, and excess secretion of GH can produce metabolic disturbances similar to those found in patients with type 2 (non–insulin-dependent) diabetes, who are also resistant to the metabolic effects of insulin. However, patients with acromegaly who have excess GH secretion are usually lean with little visceral fat whereas patients with type 2 diabetes are frequently overweight with excessive visceral fat which drives insulin resistance. We do not know the precise mechanism by which GH causes insulin resistance and decreased glucose utilization by the cells. However, GH-induced increases in lipolysis and blood concentrations of fatty acids likely contribute to impairment of insulin’s actions on tissue glucose utilization. Experimental studies indicate that raising blood levels of fatty acids above normal rapidly decreases the sensitivity of the liver and skeletal muscle to insulin’s effects on carbohydrate metabolism.␣ Necessity of Insulin and Carbohydrate for the Growth-Promoting Action of Growth Hormone GH fails to cause growth in animals that lack a pancreas; it also fails to cause growth if carbohydrates are excluded from the diet. Thus, adequate insulin activity and adequate availability of carbohydrates are necessary for GH to be effective. Part of this requirement for carbohydrates and insulin is to provide the energy needed for the metabolism of growth, but there seem to be other effects as well. Especially important is the ability of insulin to enhance transport of some amino acids into cells, in the same way that it stimulates glucose transport.␣ GROWTH HORMONE STIMULATES CARTILAGE AND BONE GROWTH Although GH stimulates increased deposition of protein and increased growth in almost all tissues of the body, its most obvious effect is to increase growth of the skeletal frame. This results from multiple effects of growth hormone on bone, including (1) increased deposition of protein by the chondrocytic and osteogenic cells that cause bone growth, (2) increased rate of reproduction of these cells, and (3) a specific effect of converting chondrocytes into osteogenic cells, thus causing deposition of new bone. There are two principal mechanisms of bone growth. First, in response to GH stimulation, the long bones grow in length at the epiphyseal cartilages, where the epiphyses at the ends of the bone are separated from the shaft. This growth first causes deposition of new cartilage, followed by its conversion into new bone, thus elongating the shaft and pushing the epiphyses farther and farther apart. At the same time, the epiphyseal cartilage is progressively used up so, by late adolescence, no additional epiphyseal cartilage remains to provide for further long bone growth. At this time, bony fusion occurs between the shaft and the epiphysis at each end, so no further lengthening of the long bone can occur. Second, osteoblasts in the bone periosteum and in some bone cavities deposit new bone on the surfaces of older bone. Simultaneously, osteoclasts in the bone (discussed in detail in Chapter 80) remove old bone. When the rate of 934 These proofs may contain color figures. Those figures may print black and white in the final printed book if a color print product has not been planned. The color figures will appear in color in all electronic versions of this book. Chapter 76 Pituitary Hormones and Their Control by the Hypothalamus GROWTH HORMONE EXERTS MUCH OF ITS EFFECT THROUGH INSULIN-LIKE GROWTH FACTORS (SOMATOMEDINS) GH causes the liver (and, to a much less extent, other tissues) to form several small proteins called insulinlike growth factors (IGFs, also called somatomedins) that mediate some of the growth and metabolic effects of GH (Figure 76-6). At least four IGFs have been isolated, but by far the most important of these is IGF-1 (somatomedin C). The molecular weight of IGF-1 is about 7500, and its concentration in the plasma closely follows the rate of GH secretion. Children with deficiency of IGF fail to grow normally even though they may have normal or elevated secretion of GH. The pygmy peoples of Africa, for example, have very small stature due to a congenital inability to synthesize significant amounts of IGF-1. Although their plasma concentration of GH is either normal or high, they have diminished amounts of IGF-1 in the plasma, which apparently accounts for the small stature of these people. Some other dwarfs (e.g., Laron syndrome) have a similar problem, generally caused by a mutation of the GH receptor and therefore failure of GH to stimulate formation of IGF-1. It has been postulated that most of the growth effects of GH result from IGF-1 and other IGFs, rather than from direct effects of GH on the bones and other peripheral tissues. Even so, experiments have demonstrated that injection of GH directly into the epiphyseal cartilages of bones of living animals causes growth of these cartilage areas, and the amount of GH required for this growth to occur is minute. One possible explanation for this finding is that GH can cause formation of enough IGF-1 in the local tissue to cause local growth. However, GH also has IGF-independent effects that stimulate growth in some tissues such as chondrocytes of cartilage. Short Duration of Action of Growth Hormone but Prolonged Action of IGF-1. GH attaches only weakly to the plasma proteins in blood. Therefore, it is released from the blood into the tissues rapidly, having a half-time in blood of less than 20 minutes. By contrast, IGF-1 attaches strongly to a carrier protein in the blood that, like IGF-1, is produced in response to GH. As a result, IGF-1 is released only slowly from the blood to the tissues, with a half-time of about 20 hours. This slow release greatly prolongs the growth-promoting effects of the bursts of GH secretion shown in Figure 76-7.␣ Sleep Growth hormone (ng/ml plasma) 30 20 Strenuous exercise UNIT XIV deposition is greater than that of resorption, the thickness of the bone increases. Growth hormone strongly stimulates osteoblasts. Therefore, the bones can continue to become thicker throughout life under the influence of GH; this is especially true for the membranous bones. For example, the jaw bones can be stimulated to grow even after adolescence, causing forward protrusion of the chin and lower teeth. Likewise, the bones of the skull can grow in thickness and give rise to bony protrusions over the eyes.␣ 10 0 8 am 12 4 pm 8 pm 12 4 am 8 am Noon Midnight Figure 76-7. Typical variations in growth hormone secretion throughout the day, demonstrating the especially powerful effect of strenuous exercise and also the high rate of growth hormone secretion that occurs during the first few hours of deep sleep. REGULATION OF GROWTH HORMONE SECRETION After adolescence, GH secretion decreases slowly with aging, finally falling to about 25% of the adolescent level in very old age. GH is secreted in a pulsatile pattern, increasing and decreasing. The precise mechanisms that control secretion of GH are not fully understood, but several factors related to a person’s state of nutrition or stress are known to stimulate secretion: (1) starvation, especially with severe protein deficiency; (2) hypoglycemia or low concentration of fatty acids in the blood; (3) exercise; (4) excitement; (5) trauma; (6) ghrelin, a hormone secreted by the stomach before meals; and (7) some amino acids, including arginine. GH also characteristically increases during the first 2 hours of deep sleep, as shown in Figure 76-7. Table 76-3 summarizes some of the factors that are known to influence growth hormone secretion. The normal concentration of GH in the plasma of an adult is between 1.6 and 3 ng/ml; in a child or adolescent, it is about 6 ng/ml. These values may increase to as high as 50 ng/ml after depletion of the body stores of proteins or carbohydrates during prolonged starvation. Under acute conditions, hypoglycemia is a far more potent stimulator of GH secretion than is an acute decrease in protein intake. Conversely, in chronic conditions, GH secretion seems to correlate more with the degree of cellular protein depletion than with the degree of glucose insufficiency. For example, the extremely high levels of GH that occur during starvation are closely related to the amount of protein depletion. Figure 76-8 demonstrates the effect of protein deficiency on plasma GH and then the effect of adding protein to the diet. The first column shows very high levels of GH in children with extreme protein deficiency during the protein malnutrition condition called kwashiorkor; the second column shows the levels in the same children after 3 days of treatment with more than adequate quantities of carbohydrates in their diets, demonstrating that the carbohydrates did not lower the plasma GH concentration. 935 These proofs may contain color figures. Those figures may print black and white in the final printed book if a color print product has not been planned. The color figures will appear in color in all electronic versions of this book. UNIT XIV Endocrinology and Reproduction Plasma growth hormone (ng/ml) 40 30 20 10 0 Protein Carbohydrate Protein deficiency treatment treatment (kwashiorkor) (3 days) (3 days) Protein treatment (25 days) Figure 76-8. Effect of extreme protein deficiency on the plasma concentration of growth hormone in the disease kwashiorkor. Also shown is the failure of carbohydrate treatment but the effectiveness of protein treatment in lowering growth hormone concentration. (Data from Pimstone BL, Barbezat G, Hansen JD, et al: Studies on growth hormone secretion in protein-calorie malnutrition. Am J Clin Nutr 21:482, 1968.) The third and fourth columns show the levels after treatment with protein supplements for 3 and 25 days, respectively, with a concomitant decrease in the hormone. These results demonstrate that under severe conditions of protein malnutrition, adequate calories alone are not sufficient to correct the excess production of GH. The protein deficiency must also be corrected before the GH concentration will return to normal. Hypothalamic Growth Hormone– Releasing Hormone Stimulates, and Somatostatin Inhibits Growth Hormone Secretion From the preceding description of the many factors that can affect GH secretion, one can readily understand the perplexity of physiologists as they attempted to unravel the mysteries of regulation of GH secretion. It is known that GH secretion is controlled by two factors secreted in the hypothalamus and then transported to the anterior pituitary gland through the hypothalamic-hypophysial portal vessels. They are growth hormone–releasing hormone (GHRH) and growth hormone inhibitory hormone (also called somatostatin). Both of these are polypeptides; GHRH is composed of 44 amino acids, and somatostatin is composed of 14 amino acids. Neurons in the arcuate and ventromedial nuclei of the hypothalamus secrete GHRH; this is the same area of the hypothalamus that is sensitive to blood glucose concentration, causing satiety in hyperglycemic states and hunger in hypoglycemic states. Secretion of somatostatin is controlled by the nearby periventricular neurons of the hypothalamus. Therefore, it is reasonable to expect that some of the same signals that modify a person’s behavioral feeding instincts also alter the rate of GH secretion. 936 In a similar manner, hypothalamic signals depicting emotions, stress, and trauma can all affect hypothalamic control of GH secretion. In fact, experiments have shown that catecholamines, dopamine, and serotonin, each of which is released by a different neuronal system in the hypothalamus, all increase the rate of GH secretion. Most of the control of GH secretion is probably mediated through GHRH rather than through the inhibitory hormone somatostatin. GHRH stimulates GH secretion by attaching to specific cell membrane receptors on the outer surfaces of the GH cells in the pituitary gland. The receptors activate the adenylyl cyclase system inside the cell membrane, increasing the intracellular level of cyclic adenosine monophosphate (cAMP). This increase has both short-term and long-term effects. The short-term effect is to increase calcium ion transport into the cell; within minutes, this increase causes fusion of the GH secretory vesicles with the cell membrane and release of the hormone into the blood. The long-term effect is to increase transcription in the nucleus by the genes to stimulate synthesis of new GH. When GH is administered directly into the blood of an animal over several hours, the rate of endogenous GH secretion decreases. This decrease demonstrates that GH secretion is subject to typical negative feedback control, as is true for essentially all hormones. The nature of this feedback mechanism and whether it is mediated mainly through inhibition of GHRH or enhancement of somatostatin, which inhibits growth hormone secretion, are uncertain. In summary, our knowledge of the regulation of GH secretion is not sufficient to describe a composite picture. Yet, because of the extreme secretion of GH during starvation and its important long-term effect to promote protein synthesis and tissue growth, we can propose the following: the major long-term controller of GH secretion is the longterm state of nutrition of the tissues, especially their level of protein nutrition. That is, nutritional deficiency or excess tissue need for cellular proteins—for example, after a severe bout of exercise when the muscle’s nutritional status has been taxed—in some way increases the rate of GH secretion. GH, in turn, promotes synthesis of new proteins while at the same time conserving the proteins already present in the cells.␣ Abnormalities of Growth Hormone Secretion Panhypopituitarism—Decreased Secretion of All Anterior Pituitary Hormones. Decreased secretion of all anterior pituitary hormones in patients with panhypopituitarism may be congenital (present from birth), or it may occur suddenly or slowly at any time during life, most often resulting from a pituitary tumor that destroys the pituitary gland.␣ Panhypopituitarism in the Adult. Panhypopituitarism first occurring in adulthood generally results from one opharyngiomas or chromophobe tumors, may compress the pituitary gland until the functioning anterior pituitary cells are totally or almost totally destroyed. The third cause is thrombosis of the pituitary blood vessels. This abnormality occasionally occurs when a new mother experiences circulatory shock after the birth of her baby. These proofs may contain color figures. Those figures may print black and white in the final printed book if a color print product has not been planned. The color figures will appear in color in all electronic versions of this book. Chapter 76 Pituitary Hormones and Their Control by the Hypothalamus Panhypopituitarism During Childhood and Dwarfism. Most cases of dwarfism result from generalized deficiency of anterior pituitary secretion (panhypopituitarism) during childhood. In general, all the physical parts of the body develop in appropriate proportion to one another, but the rate of development is greatly decreased. A child who has reached the age of 10 years may have the bodily development of a child aged 4 to 5 years, and the same person at age 20 years may have the bodily development of a child aged 7 to 10 years. A person with panhypopituitary dwarfism does not pass through puberty and never secretes sufficient quantities of gonadotropic hormones to develop adult sexual functions. In one-third of such dwarfs, however, only GH is deficient; these persons do mature sexually and occasionally reproduce. In Laron dwarfs and African pygmies, the rate of GH secretion is normal or high, but responsiveness to GH is impaired due to mutations of the GH receptor or a hereditary inability to form IGF-1, a key step for the promotion of growth by GH. Treatment With Human Growth Hormone. Growth hormones from different species of animals are sufficiently different from one another that they will cause growth only in the one species or, at most, closely related species. For this reason, GH prepared from other animals (except, to some extent, from primates) is not effective in human beings. Therefore, the GH of the human being is called human growth hormone (hGH) to distinguish it from the others. In the past, because GH had to be prepared from human pituitary glands, it was difficult to obtain sufficient quantities to treat patients with GH deficiency, except on an experimental basis. However, hGH can now be synthesized by Escherichia coli bacteria as a result of successful application of recombinant DNA technology. Therefore, this hormone is now available in sufficient quantities for treatment purposes. Dwarfs who have pure GH deficiency can be completely cured if treated early in life.␣ Gigantism and Excess Growth Hormone Before Adolescence. Occasionally, the acidophilic, GH-producing cells of the anterior pituitary gland become excessively active, and sometimes even acidophilic tumors occur in the gland. As a result, large quantities of GH are produced. All body tissues grow rapidly, including the bones. If the condition occurs before adolescence, before the epiphyses of the long bones have become fused with the shafts, height increases so that the person becomes a giant—up to 8 feet tall. The giant ordinarily has hyperglycemia, and the beta cells of the islets of Langerhans in the pancreas are prone to degenerate because they become overactive owing to the hyperglycemia. Consequently, in about 10% of giants, fullblown diabetes mellitus eventually develops. In most giants, panhypopituitarism eventually develops if they remain untreated because the gigantism is usually caused by a tumor of the pituitary gland that grows until the gland is destroyed. This eventual general deficiency of pituitary hormones usually causes death in early adulthood. However, once gigantism is diagnosed, further effects can often be blocked by microsurgical removal of the tumor or by irradiation of the pituitary gland.␣ Acromegaly and Excess Growth Hormone After Ado- lescence. If an acidophilic tumor occurs after adolescence— that is, after the epiphyses of the long bones have fused with the shafts—the person cannot grow taller, but the bones can become thicker and the soft tissues can continue to grow. This condition, shown in Figure 76-9, is known as acromegaly. Enlargement is especially marked in the bones of the hands and feet and in the membranous bones, including the cranium, nose, bosses on the forehead, supraorbital ridges, lower jawbone, and portions of the vertebrae, because their growth does not cease at adolescence. Consequently, the lower jaw protrudes forward, sometimes as much as half an inch, the forehead slants forward because of excess development of the supraorbital ridges, the nose increases to as much as twice normal size, the feet require size 14 or larger shoes, and the fingers become extremely thickened so that the hands are almost twice normal size. In addition to these effects, changes in the vertebrae ordinarily cause a hunched back, which is known clinically as kyphosis. Finally, many soft tissue organs, such as the tongue, the liver, and especially the kidneys, become greatly enlarged.␣ UNIT XIV The general effects of adult panhypopituitarism are (1) hypothyroidism, (2) depressed production of glucocorticoids by the adrenal glands, and (3) suppressed secretion of the gonadotropic hormones so that sexual functions are lost. Thus, the picture is that of a lethargic person (from lack of thyroid hormones) who is gaining weight (because of lack of fat mobilization by growth, adrenocorticotropic, adrenocortical, and thyroid hormones) and has lost all sexual functions. Except for the abnormal sexual functions, the patient can usually be treated satisfactorily by administering adrenocortical and thyroid hormones.␣ Possible Role of Decreased Growth Hormone Secretion in Causing Changes Associated With Aging In people who have lost the ability to secrete GH, some features of the aging process accelerate. For example, a 50-year-old person who has been without GH for many years may have the appearance of a person aged 65 years or older. The aged appearance seems to result mainly from decreased protein deposition in most tissues of the body and increased fat deposition in its place. The physical and physiological effects are increased wrinkling of the skin, diminished rates of function of some of the organs, and diminished muscle mass and strength. As one ages, the average plasma concentration of growth hormone in an otherwise normal person changes approximately as follows: Age (years) ng/ml 5 to 20 6 20 to 40 3 40 to 70 1.6 Thus, it is possible that some of the normal aging effects result from diminished growth hormone secretion. In fact, some studies of growth hormone therapy in older people have demonstrated three important beneficial effects: (1) increased protein deposition in the body, especially in the muscles; (2) decreased fat deposits; and (3) a feeling of increased energy. Other studies, however, have shown that treatment of elderly patients with recombinant GH may produce several undesirable adverse effects including insulin resistance and diabetes, edema, carpal tunnel syndrome, and arthralgias (joint