PHYL1010 012 Respiratory System PDF
Document Details
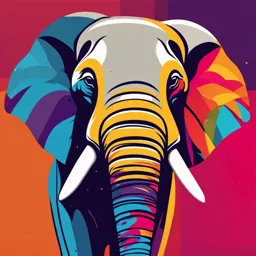
Uploaded by Mastiff
UWI, Mona
Andre S. Bowers, Ph.D.
Tags
Summary
This document is a set of lecture notes on the respiratory system, covering functions, synthesis, defense mechanisms, and more. The notes are organized into different sections for easy navigation and learning.
Full Transcript
Audio insert = slide 102 Andre S. Bowers, Ph.D. Physiology Section, Dept. Of Basic Medical Sciences, UWI, Mona Overview – main functions oPH regulation: ✓chemoreceptors linked to CO2 conc. ✓roughly 60% as bicarbonate ions ✓increase CO2 results in increased H2CO3 H20 + CO2 ---- H2CO3 + H2+ + HCO3- Ov...
Audio insert = slide 102 Andre S. Bowers, Ph.D. Physiology Section, Dept. Of Basic Medical Sciences, UWI, Mona Overview – main functions oPH regulation: ✓chemoreceptors linked to CO2 conc. ✓roughly 60% as bicarbonate ions ✓increase CO2 results in increased H2CO3 H20 + CO2 ---- H2CO3 + H2+ + HCO3- Overview – functions ✓CO2 converted to HCO3- in capillaries and shifted to plasma. ✓HCO3- transported to lungs – reduced to CO: ▪ marginal amt. free (5% ?) ✓Small quantity of CO2 present as carbaminohaemoglobin (10% ?). ✓Failure to effectively remove bicarbonate ions by lungs (as CO2) (and kidneys) results in relative acidosis. Overview – functions ▪ Compensatory mechanisms include increasing the rate of ventilation. ▪ Le Chatelier’s principle – any chemical system at equilibrium that undergoes a change in temperature, pressure or concentration, will experience a shift in order to minimize the loss of homeostasis. O2 + CO2 transport Overview – synthesis ❑Pulmonary endothelium principal func. structural in origin: ✓not metabolically inert ✓some substances prod: ▪ ACE II ▪ neutrophils ▪ chemokines ▪ surfactant. Overview – synthesis ▪ Involved in the fibrinolytic system: endothelial fibrinolysin activator --- Plasminogen --Fibrinolysin ▪ Also has roles in the coagulation cascade: source of heparin (inhibits) thromboplastin (promotes): prothrombin----thrombin ▪ WHO’s List of Essential Medicines Synthesis ❑Endocrine & metabolic: ✓Pulmonary neuroendocrine system – airway endocrine epithelial cell + assoc. nerves cells (PNEC): ▪ neuroepithelial bodies (NEB) (Van Lommel, A., 2001) ✓Growth & differentiation of neonatal lungs. ▪ PNEC – secrete amine (serotonin) & peptides (bombesin) Synthesis ❑Endocrine & metabolic: ✓NEB – O2-sensitive chemoreceptors: ▪ local + reflex-mediated regulatory control ▪ common @ airway bifurcation ▪ airway growth and dev. Storage ❑Functions as a reservoir for blood: ✓able to distend and recruit pulmonary capillaries; pulmonary beds under-perfused at rest increased CO2, sections are recruited to cope with added pressure, thereby prevent increased pulm. arterial flow ✓adjusts preload of the left heart ✓optimizes cardiac output Pleural Cavities ❑ Layer of serous membrane, between the visceral and the parietal (outer – chest wall) pleura: ✓ borders endothoracic cavity ✓ ‘potential space’ – due to closeness of contact. ❑ Contains lubricant secreted by the serous membranes: ✓ prevents friction between membranes ✓ allows for easy movement over each other during breathing. Organization of the Pleural Cavities Synthesis – defense ❑Physical barrier to blood borne substances: ✓protects against emboli - systemic infarcts. ✓particulate matter may reach alveoli. ❑Patent foramen ovale widespread – up to 25%. ✓demonstrable during Valsalva manoeuvre and coughing as flow between atria. Defense ✓functionality may be affected with foramen ovale - air emboli can enter systemic circ: ▪ possible ventilation-perfusion mismatch. ▪ neutrophil activation – increased permeability – oedema. ▪ Mechanism of acute lung injury. ❑Pulmonary epithelium uniquely positioned towards antigens: ✓SA:V-conferred adaptability potential hazard ✓> 10,000 L air --- 50-100 m2 alveoli. ❑Mucociliary escalator - pseudo-stratified ciliated columnar epithelium: ✓from posterior 2/3 nasal cavity --- respiratory bronchioles. ✓goblet cells – abundant mucopolysaccharide ✓dorsal to low-viscosity serous fluid. ✓coordinated cilia beating within serous fluid at a frequency of 10 – 15 Hz. ✓moves mucus towards pharynx – 1 mm/min in peripheral airways; 20 mm/min in trachea. ✓hence, mucus blanket = muco-ciliary escalator ✓stimulus – GC detect inflammatory mediators & irritant gases. ✓particles >5µm deposited by sedimentation in larger airways and nose ❑ Particulate matter: ✓2 – 5 µm particles deposition in lower airways. ✓ particles < 2 µm may reach the alveoli: ▪ Up to 80% removed in expired air ▪ < 20% deposited in accordance with Brownian motion: random haphazard particle collisions in a liquid or gas with walls of container. ❑Abnormalities in cilia motility &/or mucus production: ciliary motility affected by smoking, dehydration, anaesthesia, dysmotility syndrome & extreme temperatures opioids and atropine oPulmonary alveolar macrophage: ✓amoeboid cells ✓engulf particles reaching alveoli ✓deposited on mucociliary escalator ✓affinity for bacteria ✓t-cell activation, immunomodulation and antigen presentation. ✓sensitive against cigarette, asbestos & silica ✓release lysosomal prod. into extracellular matrix ✓causes inflammation fibrosis ❑Immune mediators : ✓epithelial cells secrete several substances: ▪ mucins, defensins, NO, lysozyme and lactoferrin ✓cytokines and chemokines also secreted: ▪ TNF-α, IL-1β, GM-CSF ▪ platelet activating factor – recruit these inflammatory mediators to site of inflammation. ✓immunoglobulins esp. Iga offer protection against infection: ▪ also maintains mucosal integrity. ❑Clearance of inhaled chemicals: ✓double edged sword of large alveolar SA:V ✓also increases absorptive potential of noxious chemicals/antigens. ✓determinants of their final destination include: solubility, conc., size and pulmonary metabolism both stage I and II metabolism occur in the lungs. ❑Both sympathetic & parasympathetic divisions. ❑Parasympathetic – vagal origin ✓ airway constriction, vessel dilation & increased glandular secretion. ✓ excitatory (cholinergic) motor neuron – Ach ❑Sympathetic – thoracic and cervical ganglia. ✓ airway dilation etc. ❑Pulmonary plexus: ✓ hilus of lung ✓ Pulmonary branch of vagus nerve + sympathetic trunk ✓ fibres follow bronchi, blood vessels, submucosal glands & goblet cells. ❑Devoid of voluntary motor innervation or pain fibres. ✓ pleura has some nociceptive receptors. The Alveoli ❑ Cup-shaped sacs lined by epithelium ✓ supported by thin elastic basement membrane. ✓ reminiscent of bunch of grapes. ✓ each grape represents an alveolus. ❑ Alveolar sacs are 2 or more alveoli that share a common opening. ❑ Primary site of gaseous exchange down concentration gradients. Alveoli and respiratory membrane Properties of gases ❑Atm. Pressure – combined pressure exerted by the constituent gases in a mixture of atm. gas: ✓760 mm Hg or 14.7 lbs./square inch (PSI). ✓Resp. meas. assume an atm. pressure = 0 ✓10 mm Hg = 10 mm Hg atm. pressure. ✓Resp. pressure meas. as cm H20 du to low atm. values. ✓1 mm Hg = 1.35 cm H20 ❑Partial pressure (P) – pressure exerted by a single gas in a mixture of gases. Gases ❑Water vapour like other gases – P affected by temperature: ✓in alveoli = 47 mm Hg ✓P of other gases = 760 – 47 = 713 mm Hg ❑Relative Humidity – amt. of moisture in air compared with max. amt. required to cause condensation – 100%. ❑Gases move from area of higher P outside the body and into the lungs. Pulmonary pressures ❑Airway pressure: ✓trachea, bronchi, bronchioles and the oral and nasal passages ❑Intra-alveolar pressure: ✓within alveoli – together with airway pressure = intrapulmonary pressure. ✓Intrapulmonary = 0 mm Hg just before inspiration glottis open and there is no air flow ❑Intrapleural: ✓within pleural cavity ✓always –ve relative to alveolar pressure ✓-4 mm Hg with open glottis ✓alveoli spaces open to atm. Pulmonary pressures ❑Intrathoracic pressure: ✓pressure within thoracic cavity. ✓heart and great vessels also exposed to this. ✓approximates pleural pressure. ❑Valsalva manoeuvre – forced expiration against a closed glottis: ✓increases intra-thoracic and intrapleural pressures. Gas Laws ❑ Basic atmospheric conditions: ✓atmospheric pressure is 760 mm Hg. ✓atmospheric components: nitrogen = 78,09 % oxygen = 20.95 % carbon dioxide = 0.039 % argon 0.93 % Boyle’s Law ❑ Characterizes the relationship between pressure and volume ❑ There is an inverse relationship between the pressure and volume of a gas in a system: ❑P1V1 = P2V2 Boyle’s Law ❑ Vol. gas/unit time = area/thickness x constant x (P1 – P2): ✓ PV = k ❑ Changes in intrapulmonary pressure occur as a result of changes in lung volume. ✓pressure inversely prop. to vol. ❑ Underscores kinetics of ventilation: i. increased lung vol. decreases intrapulmonary pressure: ▪ -ve rel. to environ - air moves into lungs. ii.decreased lung vol., raises intrapulmonary pressure above atmospheric: ▪ +ve pressure - air expelled rel. to vol. contraction. ❑ Boyle’s Law – early spirometry ✓thoracic expansion (vessel vol.) requires a pressure drop to maintain equilibrium thus, intrathoracic press. below 760 mmHg ✓ thoracic contraction --- pressure rise more +ve than environ Boyle’early experiments: Dalton’s Law ❑Law of gas partial pressures: ✓each gas in a mixture of gases exert a pressure independent of other constituent gases: ▪ total mixture = sum of parts ❑Hence, atm. pressure (760 mm Hg) & rel. conc. of diff. gases in air --- total atm. impact /per gas ✓PO2 conc. air = 20.95% ✓760 mmHg (Patm.) x % 0.21 gas in atmosphere PO2 = 760mmHg x 21% (.21) = 160 mm Hg Dalton’s Law ❑ Net gas diffusion across a fluid membrane is: ✓ proportional to the p.p. differential ✓ proportional to membrane s.a. ✓ inversely to membrane thickness ❑ Determinants of the rate of diffusion: ✓length of diffusion barrier ✓conc. gradient ✓molecule size ✓temperature Ideal gas law ❑ Ideal Gas law: ✓ pressure + vol. of gas is directly related to the temp. and the particle conc. ✓ PV = nRT n = moles of gas T = absolute temp. R = universal gas constant @ 8.3145 J/K·mol Diffusion ❑Respiratory portions of lungs – alveolar--capillary membrane. ❑Consistent with Fick’s Law of diffusion: ✓volume of gas crossing alveolar-cap’/time proportional to: ▪ ▪ ▪ ▪ Vgas P1 – P2 Surface area: volume (SA) diffusion coefficient (D) ✓diffusion, however, is inversely prop. to membrane thickness (T). Diffusion - modifiers ❑Diffusion capacity: ✓high O2 administration increases the p.p. difference. ✓diseases that calcify lung parenchyma: ▪ affect S.A. of respiratory surfaces ✓conditions that increase alveolar-cap’ thickness. ✓gas properties – mol. wgt., solubility ▪ det. diffusion coef. – gas transfer rate/p.p ▪ CO2 more soluble than O2 – 20x faster CO – diffusion capacity estimator ❑Higher affinity for haemoglobin – 210X ❑Diffusion is not a limiting factor as observed with CO2 and O2. ❑Negligible CO present in venous blood. ❑Mechanisms effectively clear respiratory membranes – lower p.p. ❑Single breathe test: ✓inhale dilute CO ✓10-sec breathe-hold Gas transport + exchange ❑ Sequence of events resulting in gas transport from environ ---- cell. ✓complex coordinated homeostatic process. ❑ Terrestrial vertebrates it includes 4 phases: I. Ventilation: inspiration & expiration II. External respiration: gas transfer from naso-oral cavities ---- alveolar-capillary barrier. III. Transport of gases by the blood ▪ oxyhaemoglobin Gas transport + exchange IV. Internal respiration: Gas exchange between blood and tissue fluid: ✓ cellular respiration in cells causes the tissue fluid to have a lower PO2 and a higher PCO2 than the blood. ✓ O2 released & moves from high to low press in tissue cells fluid and eventually in the tissue cells. ✓ CO2 moves down its conc. gradient in ‘venular’ microcirculation & ultimately, across ventilation-perfusion. Gas transport + exchange ❑ Cellular respiration ✓ independent metabolic process. ✓ is the process whereby an organism uses oxygen and food to produce energy (ATP), water and carbon dioxide ✓ glucose + O2 ------- ATP + H2O + CO2 ❑ Therefore gaseous exchange culminates in oxygen transport and delivery to tissues, then cells for cellular respiration. Intrapulmonary pressures ❑Lungs & chest are both elastic – opposing pull: ✓without the thoracic cavity, lung capacity would be lower ✓chest expands w/ lung resection ❑Opposing pull create a drag on visceral and parietal layers: ✓precipitates a –ve pleural pressure ✓expansion --- more -ve than in the deflating lung. ✓in absence of –ve force attaching lungs to chest wall - collapse. ❑Intrapleural pressure become > atm. = expels air: ✓– coughing, forced expiration. External Respiration Internal Respiration Thoracic cavity + respiratory muscles ❑Ventilation necessitates a closed compartment: ✓lungs and airways lie in thoracic cavity with heart, great vessels and oesaphagus. ✓outer wall of 12 rib pairs, sternum, thoracic vertebrae and intercostals. ✓bounded at top by neck & diaphragm at the bottom. ❑Breathing involves thoracic pressure and vol. expansion & contraction: ✓mechanical recoil & extension of the thoracic wall w/ lungs and associated muscles Other considerations ❑Lung compliance. ❑ Alveolar surface tension & surfactant. ❑ Influence of airway diameter ❑ Minute respiration (ventilation rate * tidal volume) & anatomical dead space ✓ leading to a more accurate idea of alveolar ventilation rates ❑ Changes in ventilation patterns Other considerations Lung compliance ❑Refers to the distensibility of upon inspiration. ❑The change in lung volume per unit change in intrapulmonary pressure: ✓specifically, for every cm/H20 rise in intrapulmonary pressure, the lung expands by 200 mL. ✓> 1 cm/H20 required to expand the noncompliant lung ❑Determined by lung elasticity, surface tension, H20 content & rib cage distensibility. Lung compliance ❑Elastin fibres – easily stretched + aids inflation. ❑Collagen fibres – not as elastic and opposes inflation. ❑Interstitial lung disease & fibrosis – low compliance: ✓ elastin replaced by stiff collagen fibres. ✓ deposition due to scarring. ❑Emphysema – high compliance lung recoil affected: ✓ > compliance = > inflating potential ✓ deflation is compromised by aberrant recoil: ▪ leucocyte-mediated tissue degradation Surface tension ❑Closely associated with lung compliance. ❑Energy req. to raise S.A. by 1 unit area. ❑Alveolar film – cohesive inter-molecular forces in surface fluid – causes ‘tension’: ✓Fluid in bulk phase, random dist. + mol. collision ▪ involves all possible directions ✓on surface of membrane layer: ▪ cohesive forces act only downwards ▪ tensile force – ‘threshold’ or ‘yield’ Surface tension ❑Therefore, directly opposes distensibility. ❑Inter-mol. cohesion more pronounced in fluid than gases. ❑Type 2 alveolar cells – synthesize metabolite that reduces alveolar surface tension. ❑Above normal S.T. causes film to contract hinders lung inflation: Surface tension ❑Surfactant - ✓WHO’s Model List of Essential Medicines. ✓DPPC: ▪ amphipathic ▪ bridges divide between alveoli-film and duct- air interface ▪ hydrophilic component --- alveoli fluid ▪ hydrophobic tail --- air in duct/resp. bronchioles Surface tension ❑Type II pneumocytes – phospholipid, protein + neutral lipids. ❑Minimize the effect caused by cohesive attraction of fluid molecules within alveoli surface monolayer: ✓tensile force on inner-face fluid surface is interrupted: ✓lowers attractive forces caused by H+ bonding by becoming interspersed between H20 molecules Surface tension 1. Type I cells – approx. up to 95% total S.A.: ✓continuous w/ epithelia of pulm. Capillaries ✓hence, structural - ≈ 90% epithelium ✓squamous – adept for high S.A. ✓gas exchange layer cells – thin layer (25 nm): ▪ organelles displaced 2. Type II – secretory via lamellar bodies ✓surfactant - aqueous phase ✓cell division possible ✓exocytic release 3. Alveolar macrophage Surface tension Surfactant Surfactant ❑ Hydrophilic head towards fluid monolayer where it disrupts the potency of intermolecular cohesion: ✓hydrophobic tails towards air-space ✓micelle formation at threshold (CMC) ❑Alveolar radius inversely related to surfactant efficacy or, alternatively, capacity to reduce surface tension. Intramolecular water tension Surfactant ❑Compliance & ease of breathing: ✓lung inflation requires more energy without. ✓prevents alveoli from sticking together during expiration. ✓keep alveoli dry – protects against oedema: ▪ H20 sequestered from capillaries with increased ST. ▪ alveolar contraction. ✓stabilizes alveolar inflation: ▪ changes shape rel. to size. ▪ surfactant more compressed in smaller alveoli. Surface tension ❑Law of Laplace – Describes rel. btw pressure + wall tension within sphere: ✓tension at air-H2O interface and wall tension: ✓P inversely & directly related to r & ST, resp. ✓inward P cause - interface ‘curvature ▪ lowered by pulmonary surfactant. ✓net inward cohesion-driven mol. force: ▪ opposing - +ve from liquid or –ve from air Pressure tension relationship - Law of Laplace Area tension relation ship Airway resistance ❑Vol. of gas reaching respiratory portions determined by: ✓pressure difference btw environ & atm. ✓inversely related to resistance within vessels. ❑Hence, resistance = inspiration or expiration pressure: resistance to airflow. ❑ Described by Poiseuille in circular tubes: R = 1/r4 ❑Total R = sum of press. Through resp. tree: ▪ func + struc. boundaries Types of airflow ❑Laminar – occurs in small vessels where flow in low and streamlined: ✓airflow is parallel to sides of vessel ✓peripheral air exposed to > R ✓flow in middle of conduit faster. ❑Turbulent: chaotic airflow as mol. collide, move laterally & change direction and velocities: ✓driven by flow rate, vessel radii & molecular interaction. ✓high flow vessels s.a. the trachea. ✓basis for auscultation. Airway compression – forced expiration ❑No change in R during tidal breathing: ✓Changes significantly with exertion ✓Airflow requirement - distending (intrapulmonary) > compressing forces (intrathoracic) ✓Forced expiration - transpulmonary pressure falls due to asymmetrical rise in intrathoracic pressure compared with airway pressure. ▪ R on outflowing air causes further fall in airway P. ▪ Above a certain threshold, the external intrathoracic pressure will compress the collapsible airways. Lower respiratory tract ❑ Gas exchange: ✓extensive SA = 1 x 105 m2 of type I alveolar cells (simple squamous epithelium) ✓ associated network of pulmonary capillaries ▪ 80-90% of the spaces between alveoli are perfused by pulmonary capillaries ✓exchange distance roughly 1 µm from alveoli to blood. ❑ Protection: ✓free alveolar macrophages - dust cells ✓surfactant – mechanical – type I pneumocytes Exchange membrane - properties ❑High volume of blood through immense capillary network. ❑Mass volume displacement in pulmonary circ.: ✓pulmonary circulation = 5L/min through lung ✓whilst, systemic circulation = 5L/min through entire body. Ventilation ❑Gas exchange in respiratory system. ❑Movement of air in and out of the body: ✓ inspiration - movement of air into respiratory tracts ✓ expiration = movement of air out of respiratory tracts ✓ respiratory cycle - one inspiratory-expiratory manoeuvre ❑Principle of Inspiration: ✓ Physiology: contraction of the inspiratory muscles causes increase in the thoracic cavity, allowing air to enter the respiratory tract ✓ physics: fall in thoracic cavity pressure below atm. press. causes a pressure drop in the respiratory tract, creating a pressure gradient which causes molecular movement to favor moving into the respiratory tract Ventilation ❑ Pressure gradients est. by changes in the size of thorax: ✓ muscle contraction & relaxation ❑ Remember Boyle’s law: the volume of gas varies inversely with pressure at a constant temperature. Inspiration ❑ Contraction of the diaphragm and external intercostals – cause thoracic cavity expansion: ✓expansion of the thorax results in decreased intrapleural pressure - decreased alveolar pressure. ✓air moves into the lungs when alveolar pressure drops below atm. pressure. ✓compliance: ability of pulmonary tissues to stretch - distensibility Balloon Model of Inspiration Mosby items and derived items © 2010, 2007, 2003 by Mosby inc., an affiliate of Elsevier Inc. Mechanism of inspiration Mosby items and derived items © 2010, 2007, 2003 by Mosby inc., an affiliate of Elsevier Inc. Expiration - mechanism ❑ Passive process that begins when the inspiratory muscles are relaxed - decreases thoracic volume: ✓increases intrapleural pressure. ✓increases alveolar pressure above the atm. pressure. - air moves out of the lungs. ✓pressure between parietal and visceral pleura is always less than alveolar and atmospheric pressures: diff. btw intrapleural and alveolar press. – transpulm. press. always –ve – otherwise collapsible ✓elastic recoil tendency to return to a smaller size after stretch Balloon Model of Expiration Mosby items and derived items © 2010, 2007, 2003 by Mosby inc., an affiliate of Elsevier Inc. Mechanism of expiration Mosby items and derived items © 2010, 2007, 2003 by Mosby inc., an affiliate of Elsevier Inc. Recall, pressures important in ventilation Thoracic volume due to muscular & diaphragmatic contraction & relaxation Pulmonary pressures ❑ Intrapleural normally –ve than atm.: ✓press. varies with insp./exp. stage, atm. + i.p. vol. ✓resting intrapleural press. is sub-atmospheric: ▪ recoil of chest + lungs ✓transpulm. press. favours passive vol. expansion: ▪ Ptp = Palv – Pip Pulmonary pressures ❑Generally: Ptp = +ve ✓Palv = -ve - +ve ✓Pip = -ve ✓ ❑Pneumothorax – air inside pleural space: ✓Ptp = 0 ; Palv = Pip ✓elasticity-induced collapse ✓also w/ resection ✓Pt = - elastance Quiet (tidal) inspiration ❑ Active process: ✓ contraction of diaphragm, increases thoracic volume vertically. ✓ contraction of parasternal and internal intercostals, increases thoracic volume laterally. ❑ Pressure changes: ✓ alveolar changes from 0 to –3 mm Hg. ✓ intrapleural changes from –4 to –6 mm Hg. ✓ transpulmonary pressure = +3 mm Hg. Quiet expiration ❑Quiet expiration is a passive process. ✓after stretched --- lungs recoil. ✓smaller vol. --- increased alveolar press above atm. --pushes air out. ❑Pressure changes: ✓intrapulmonary pressure changes from –3 to +3 mm hg. ✓intrapleural pressure changes from –6 to –3 mm hg. ✓transpulmonary pressure = +6 mm Hg. Modifications to ventilation ❑ Both inspiratory & expiratory manoeuvre can be modified: ✓forced or active inspiration ✓forced or active expiration ❑ The larger and quicker the expansion of the thoracic cavity, the larger the gradient: ✓faster air transport down pressure gradient ✓not a correlate of gas exchange Ventilation - Distribution ❑Reflects the effect of compliance, body composition and gravitational forces. ✓ more –ve at apex in orthostatic position. ✓ become more ventilated as a result – less compliant ❑Since compliance = vol. change/change in intrapleural ✓ less in distended lung ✓ smaller in alveoli with less air ❑Some air remain in alveoli: ✓ may increase in the elderly. ✓ lower elastic recoil eg. emphysema. ✓ > residual volume. O2 and CO2 transport ❑Blood serve as media for gas transport. ❑Transported as complexed or in dissolved states. ✓Co2 also transported as HCO3-. ❑Arterial blood utilized in meas. of blood gases. ❑Oxygen: ✓98 – 99% as oxy-haemoglobin ✓remainder in dissolved state ✓permeates capillary wall + cell mem. ✓cellular metabolism ✓Po2 reflects each component. Alveolar-capillary transfer Hb conc. and O2 affinity ❑ Haemoglobin - reduced and oxygenated forms. ❑A Hb conc. of 15 g/dL when fully saturated carries 20.1 mL of O2/dL of blood. ❑O2 saturation vary between 95 – 100% upon left heart exit ✓ Falls to about 75% in venous blood returned to the right heart. ❑Solubility coef. Of O2 in plasma is low – 0.003%. ✓Henry’s Law: p.p. of O2 in atm. is proportional to dissolved O2 concentration Conc.-pressure relationship O2 transport ❑Hence, at 95 mmHg roughly 0.29 mL of O2 is dissolved in every dl of blood. ❑Hence, >3% of O2 transported in dissolved state. ❑CO poisoning and dissolved O2? ❑Hyperbaric chamber – 100% O2 at high atm. pressure. Hb + O2 transport ❑Same old principle – hb binds at high affinity and releases when reduced. ✓polypeptide chains with iron-containing heme group. ❑O2 binds to each heme group via iron atom – 4 mol. of O2 ❑Cooperative binding – each Hb bound increases the affinity for the successive Hb mol. ❑Hb unloading is effected in a similar fashion. ❑Oxygen-haemoglobin dissociation curve – describes relationship between SpO2 and p.p. of O2 Hb – globin chains + heme O2-hb-dissociation curve ❑Sigmoidal curve – slope represents O2 release at lower Po2. ✓ @ higher plateau – depicts oxygen binding in pulm. system. ❑Curve shape due to conformational changes to Hb. ❑At plateau Hb is almost fully saturated – increasing PAO2 has no effect. ❑Steep portion shows that rel. small decreases in PO2 causes considerable increase in Hb unloading. ❑Hb adapted to function as a buffer system: ✓ change in Hb affinity for O2 to suit metabolic needs. ✓ represented by change in curve position – left vs. right shift Hb-dissociation curve Dissociation curve - determinants ❑Red blood cell 2,3-diphosphoglycerate (DPG) – intermediate metabolite ✓ reduction in Hb O2 affinity ✓ promotes unloading ✓ increased by altitude, hypoxia and exercise ❑Binding is increased with: ✓ HCO3✓ temp. reduction ✓ decrease CO2 conc. ❑Unloading favoured by: ✓ acidosis ✓ increased CO2 ✓ fever CO2 transport ❑Transported in three forms throughout the body 1. native state - 10% 2. as HCO3- 60% 3. as carbaminohaemoglobin 30% ❑Dissolved CO2 and HCO3- determine acid-base balance. ❑Majority diffuses into erythrocytes, forming HCO3and H2CO3+. CO2 + H2O = H+ + H2CO3- CO2 transport ❑The process is catalyzed by carbonic anhydrase. ✓increases reaction rate, x5000. ❑H2CO3 converted to H+ HCO3-: ✓H+ combines with Hb – buffer system. ✓bicarbonate ion diffuses into plasma in place of cl-. ✓venous water and cl- > arterial blood. ❑CO2 dissolved in plasma is a function of: ✓partial pressure ✓solubility coefficient (0.03 mL/dL/mm Hg Pco2) CO2 transport ❑Haemoglobin-CO2 bonding is reversible – loose bonds. ✓affinity increases in absence of oxygen. ✓moreover, solubility 20 > O2. ❑Moves in opposite direction of O2 transport. ✓ eventually expelled by lungs. ❑Loading of O2 in lungs displaces CO2. ❑Binding is determined by Hb acidity: ✓CO2 bonding with Hb enhances its acidity. ✓thus, in lung > tendency for carbaminohaemoglobin complex. modulates CO2 alveolar release ✓tissue Hb acidity Basic spirometer Lung function testing ❑Volumes & air in lungs must remain constant for normal exchange of oxygen and carbon dioxide: ✓Spirometer: instrument used to measure the volume of air ✓Tidal volume (TV): amount of air exhaled after normal inspiration, 500 ml ✓Expiratory reserve volume (ERV): largest volume of additional air that can be forcibly exhaled past the TV(1000-1200 ml is normal ERV) Lung function testing ✓Inspiratory reserve volume (IRV): amount of air that can be forcibly inhaled after normal inspiration (normal IRV is 3300ml) ✓Residual volume: amount of air that cannot be forcibly exhaled (1200 ml) Lung function testing ❑ Lung capacities: sum of two or more pulmonary volumes: ✓Vital capacity (VC): the largest volume of air that can be moved in and out of the lungs; IRV + TV + ERV ▪ determinants include - size of the thoracic cavity and posture ✓Functional residual capacity (FRC): the amount of air at the end of a normal respiration: ▪ ERV + RV Lung function testing ✓Total lung capacity (TLC): the sum of all four lung volumes; the total amount of air a lung can hold ✓Inspiratory capacity (IC): the maximal amount of air an individual can inspire after a normal expiration; TV + IRV Lung function testing ❑ Alveolar ventilation: volume of inspired air that reaches the alveoli ❑Alveoli must be properly ventilated for adequate gas exchange. ❑Anatomical dead space: air in passageways that do not participate in gas exchange. ❑Physiological dead space: anatomic dead space plus the volume of any nonfunctioning alveoli (as in pulmonary disease) Lung function testing ❑Pulmonary air flow: rates of air flow into and out of the pulmonary airways: ✓Total minute volume: volume/min. (ml/min): ✓ VT x RR = 6000 ml/min ✓Forced expiratory volume (FEV) or forced vital capacity (FVC): volume of air expired per second during forced expiration (as a percentage of VC) ✓Flow-volume loop: top of the loop represents expiratory flow volume: ▪ bottom of loop = inspiratory flow volume ▪ apex = PEFR Volumes + capacities Ventilatory rhythm Lung disorder classification_c ❑ Restrictive disorder: – VC is reduced. – FVC is normal. ❑ Obstructive disorder: – VC is normal. – FEV1 is < 80%. NORMAL FEV1 = 3.0L FVC = 4.2L FEV1/FVC = 80% OBSTRUCTIVE FEV1 = 0.9L FVC = 2.3L FEV1/FVC = 40% RESTRICTIVE FEV1 =1.8L FVC = 2.3L FEV1/FVC = 90% Tidal ventilation oAir moves in and out of the body via the same route. ✓All terrestrial vertebrates. oThe lungs are not completely emptied during each breathing cycle. oThe air entering mixes with used air remaining in the lungs. oThis help to conserve water, but decreases gas-exchange efficiency. Determining lung capacity o A spyrometer can be used to determine how much air enters the lungs. o Your lungs has a volume of +/- 5 liters. o During a normal breath, only 0.5 liters of air is exchanged – This air is known as tidal volume. o During forced breathing, as much as 3.5 liters of air can be exchanged, this is known as vital capacity. (The fitter you are, the higher your vital capacity.) o +/- 1.5 liters of air always remains in the lungs – this air is known as residual air/volume. Dead space oThat component of the respiratory tract that is not involved with gaseous exchange. o Large respiratory vessels: ✓ Not thin enough to allow gas movement – multicellular layers ✓ Conducting zone - nose, trachea, bronchi -- broncial tree) oAptly referred to as the anatomic dead space. ✓ Morphologically determined. oIt is about a 1/3 of the tidal vol. - 150 mL. Dead space oPossible determinants?? ✓ Length + diameter of vessels ✓ Birds (longer + wider) than mammals ✓ Giraffe? o Tidal volume (TV) oIncreased dead space - increasing lung vol., old age, neck extension, jaw protrusion, bronchodilators, anaesthesia mask and snorkel. oDecreased dead space - endotracheal intubation, bronchoconstrictors, hyperventilation (fall in lung vol.) Dead space oAlveoli - sites of gas exchange. oAlveolar dead space - due to an absence of perfusion: ✓Ventilation without perfusion. ✓Wholly + partially collapsed alveoli. ✓Interstitial fibrosis oAnatomic + alveolar = physiological dead space. Dead space oAlveolar deaddisturb space the - increased incapacity@ lung disease states which diffusion alveolar-capillary membrane: ✓Increased alveolar dead space due to a relative lack of alveoli distensibility. ✓Consequently, gases are unable to readily traverse alveoli. ✓Thus minimizing the vol. of O2 flowing to these areas. ✓Alveolar ventilation plummets as a result. ✓Evidenced in - pulmonary oedema, interstitial lung disease, embolism & hypotension. Dead space & TV oTidal volume is the vol. of air inspired & expired in each respiratory cycle: ✓Approx. 500 mL oVary by anthropometric measures: ✓age, sex and race. ✓Weight and height oThe tidal volume (expired air) - equal to alveolar ventilation + dead space ventilation Va = Vt - Vd. Dead space & TV oSo, how would the concomitant rise in DS and fall in TV affect gas exchange? oIncreasing the TV, while DS remain constant? oHence, lowering the depth of respiration and the dead space has the same effect on respiratory effort. oHow would increasing trachea length affect respiration? Ventilation/perfusion (V/Q) ratio oMeasure of the balance between the vol. of O2 delivered to the respiratory system (by ventilation) and the vol. of blood reaching the alveoli (by perfusion). oQuantifies the efficacy of V/Q. oThe lungs are imperfect – asymmetric distribution of V/Q: ✓V/Q varies from apex to base ✓Apex (1/0); Mid (1/1) and Base (0/1). Ventilation/perfusion (V/Q) ratio oIt normally varies btw 0.8 - 0.9. oThe volume of oxygen supplied to the pulmonary capillaries is generally just enough to fully saturate the blood: ✓1 mL blood can contain 200 mL of O2, while 1 mL dry air has about 210 mL of O2. Ventilation/perfusion (V/Q) ratio oWhen there is a mismatch between vent and perfusion, we have what is known as ventilation perfusion mismatch. oIf DS is increased or decreased beyond normal, exchange is affected and there is vent/perf mismatch. ✓Ex: Moving from supine to the orthostatic position: the force of gravity cause more blood to flow to the base of the lung. Ventilation also increased, but non-linearity. Hence, V/Q oVQ also affected by the anatomical positioning of the lungs. Perfusion - distribution oPulmonary blood flow driven by body composition and gravity: ✓Causes differences in different capillaries. oStanding position – apex of lung above heart level: ✓Exceed perfusion capacity of MAP. ✓Anterior is thus less perfused than base. oSupine - Base and apex at same level: ✓Perfusion become more uniform throughout. ✓Flow in dependent positions may exceed apices. ✓R/Q mismatch. Ventilation/perfusion matching oHypoxia elicit marked vasoconstriction: ✓Occurs below 60 mm Hg. ✓Local flow abolished at very low levels. ✓During localized hypoxia flow is directed towards the hypoxic regions. ✓Generalized hypoxia results in vasoconstriction throughout the lungs. Triggered by lung disease and high altitude fall in O2. ✓Prolonged vasoconstriction causes PH and increased workload on right heart. Ventilation/perfusion - shunts oRefers to the movement of blood from the right to the left circulation without entering the pulmonary circulation. oTwo types – anatomic and physiologic shunts. oAnatomic – moves from venous to arterial while bypassing lungs. oPhysiologic – inadequate oxygen supply to oxygenate particular vol. of blood in pulmonary capillaries. ✓ V/Q mismatch. ✓ Destructive lung disease interfering with air movement through the lungs. V/Q matching (530) o Lung exchange properties ensure there’s a symmetric distribution of air and blood. o Modlated by both the dead space air and physiological shunts: ✓ Dead space causes > ventilation without perfusion: Pulmonary embolism – impaired blood flow to some areas. ✓ Shunts cause > perfusion without ventilation: Airway obstruction o Pulm. Arterial blood reflects blood from areas having normal ventilation and perfusion: ✓ Also from shunt areas. ✓ Dead space areas also included. Respiratory Physiology o Regulation of respiration: ✓ Integration of neural inputs by pons and medulla: ✓ Medulla – controls ventilator rhythm ✓ Pons - coordinates respiratory rate, excitation and inhibition (pneumotaxic centre). o Homeostasis: maintenance of CO2, O2 and H+: ✓ Compensation of acid-base disturbances – pCO2 ✓ Fall in pO2 ✓ Rise in pCO2 potentiates the fall in O2. Fig. 4 The regulation of respiration Respiratory centre oNormal breathing rate for adults: 12 – 20 ventilations per minute. oRespiratory Center in the Medulla Oblongata controls breathing. oRespiratory center send impulses through the phrenic nerve to the diaphragm & the intercostal nerve ✓Intercostal muscles either contract or relax ✓Contraction during inspiration ✓Relaxation during expiration Breathing - Nervous control Brain Respiratory center automatically regulates breathing Intercostal nerves stimulate the intercostal muscles Intercostal muscles Pheric nerve stimulates the diaphragm Diaphragm Oxygen transport oThe principal oxygen transporter is haemoglobin ✓red pigment protein oOxygen combines with hemoglobin to form oxyhemoglobin. Hb + O2 = HbO2 oA small amount of oxygen is transported in solution in the blood plasma. Haemoglobin oConsist of 4 polypeptide chains (protein – 2 alpha and 2 beta). oEach chain is associated with a heme group. oEach heme group contains an iron atom. Each red blood cell carries 250 million Hb molecules = 1 Billion Oxygen molecules Carbon dioxide transport oMost CO2 is transported as bicarbonate ions (HCO3-) oCO2 binds with water to form carbonic acid (H2CO3) CO2 + H2O = H2CO3 oThen carbonic acid dissociates to form hydrogen and bicarbonate ions. H2CO3 = H+ + HCO3- Carbon dioxide transport oA small amount of carbon dioxide is transported by the Hemoglobin molecules in the form of carbaminohaemoglobin (HbCO2). o CO2 + Hb = HbCO2 oThe higher the amount of hydrogen ions in the blood the lower the pH. ✓Hydrogen ions bond with the globin part of Hb to keep the pH normal in the blood. Pulmonary diseases oLower respiratory tract disorders caused by exposure to infectious pathogens &/or polluted air: ✓Pneumonia ✓Pulmonary Fibrosis ✓Pulmonary Tuberculosis ✓Emphysema ✓Bronchitis ✓Asthma Pneumonia o Bacteria streptococcus can cause pneumonia. o Infiltration of alveoli by products of inflammation and scarring. Pulmonary fibrosis o Fibrous connective tissue builds up in lungs, reducing their elasticity and Oxygen diffusion. Tuberculosis oCaused by a bacillus bacterium Emphysema o Alveoli burst and fuse into enlarged air spaces. – Surface area for gas exchange is reduced. Bronchitis o Airways are inflamed due to infection (acute) or due to an irritant (Chronic). Coughing brings up mucus and pus. Asthma o Airways are inflamed due to irritation and bronchioles constrict due to muscle spasms. Smoking and lung disorders oSmoking causes almost 90% of all lung cancers and is also a major cause of emphysema. Healthy normal lung of a nonsmoker Lung of a smoker Lung with large tumors causing lung cancer