Pharmacology 301 lecture notes.docx
Document Details
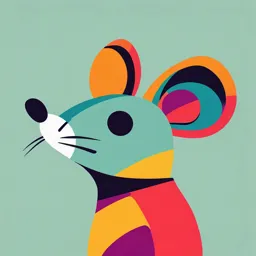
Uploaded by SteadfastWisdom924
Ladoke Akintola University of Technology
Full Transcript
**INTRODUCTION TO PHARMACOLOGY** **Introduction** **Pharmacology** is the branch of medical sciences that is concerned with the study of the uses, effects and modes of action of drugs. **A drug** can be defined as any natural, synthetic or endogenous molecule which can exert a physiological and/or...
**INTRODUCTION TO PHARMACOLOGY** **Introduction** **Pharmacology** is the branch of medical sciences that is concerned with the study of the uses, effects and modes of action of drugs. **A drug** can be defined as any natural, synthetic or endogenous molecule which can exert a physiological and/or biochemical effect on the cell, tissue, organ, or whole organism, OR a drug is any chemical substance that has the ability to alter the functions of a living system. In the field of [pharmacology](https://en.wikipedia.org/wiki/Pharmacology), [drug](https://en.wikipedia.org/wiki/Pharmaceutical_drug)s are also called medication or medicine and are used for the purpose of [treat](https://en.wikipedia.org/wiki/Pharmacotherapy)ing, curi[ng](https://en.wikipedia.org/wiki/Cure), [prevent](https://en.wikipedia.org/wiki/Preventive_healthcare)ing, or [diagnosing](https://en.wikipedia.org/wiki/Medical_diagnosis) a [disease](https://en.wikipedia.org/wiki/Disease) or to promote health and [well-being](https://en.wikipedia.org/wiki/Well-being). Traditionally, drugs were obtained through extraction from [medicinal plants](https://en.wikipedia.org/wiki/Medicinal_plants), but more recently also by [organic synthesis](https://en.wikipedia.org/wiki/Organic_synthesis). The interactions of a drug with a biological system (**the body**) are bidirectional; the drug interacts with the body and the body also interacts with the drug. **In recent times over 350 thousand drugs have been approved for one use or another worldwide in some countries there exists over 147 preparations of Diclofenac-sodium and 60 approved preparations of Paracetamol (Acetaminophen) are available. For most drugs only single international name exists although they may have different trade names.** From early in human history, pharmacologically active substances (e.g., from plants and animals) have been used to ward off or treat disease. Drugs can be molecules synthesized in the body (e.g., hormones or neurotransmitters \[e.g. dopamine, epinephrine, acetylcholine) or molecules *not* synthesized in the body (i.e., ***xenobiotics***, from the Greek *xenos*, meaning "stranger"). ***Poisons*** are drugs that almost exclusively have harmful effects. The terminology pharmacology originated from two words a) a Greek word ***pharmakon*** meaning a drug or poison and b) ***logos*** meaning a word or a discourse. The two main areas of pharmacology deal with the actions of drugs on the body (**pharmacodynamics**) and the fate of drugs in the body **(pharmacokinetics).** In broad terms, pharmacodynamics involves the interactions between chemicals (the drugs) and their biological [receptors](https://en.wikipedia.org/wiki/Receptor_(biochemistry)), while, pharmacokinetics involves the **a**bsorption, **d**istribution, **m**etabolism, and **e**xcretion (ADME) of chemicals from biological systems. Pharmacology usually overlaps with **pharmacy** which is the science of drug preparation; much of it deals with **clinical pharmacology**/**therapeutics** which is the use of drugs in the treatment of disease (by whatever route). **Toxicology** is the branch of pharmacology dealing with the \"undesirable\" effects of drugs on biological processes, or generally the study of poisons. The field of pharmacology also encompasses **a)** drug properties and the composition of drugs, **b)** synthesis of drugs and drug design, **c)** molecular and cellular [mechanisms](https://en.wikipedia.org/wiki/Mechanism_of_action) of drug action, **d)** signal transduction and cellular communication. **In recent times, the genomes of humans,** mice, and many other organisms have been decoded in considerable detail, opening the door to a number of new approaches to research as well as disease treatment. It has been known for centuries that certain diseases are inherited, and we now understand that individuals with such diseases have a heritable abnormality in their DNA. Our understanding of the human genome has afforded us the opportunity to define exactly which DNA base pairs involved in a number of hereditary disorders and also investigate the possibility of correcting the abnormality using **\"gene therapy,\"** i.e, insertion of an appropriate \"healthy\" gene into somatic cells. Also, it is known that some patients respond to certain drugs with greater than usual sensitivity; our understanding of genomics has now made it clear that such increased sensitivity is often due to a very small genetic modification that may result in decreased activity of a particular enzyme responsible for eliminating that drug. **Pharmacogenomics** (or pharmacogenetics) is the study of the genetic variations that cause individual differences in drug response. **Why are drugs important for health and scientific research?** **Discovery and development of drugs** (including immunotherapy/ vaccinations) has been a major factor that has increased life span and improved the quality of life. New scientific insights; in some cases, inferred from development of new mechanisms of action have been essential to this progress, together with controlled clinical trials, in particular and ***randomized*, *double-blind trials*** In parallel (especially in recent years) there has been the promotion of "alternative" and "complementary" treatments, many (most) of which have *not* undergone rigorous scientific validation. The search for "magic bullets" which are agents that treat disease or produce desirable effects but lack harm, and ways to improve such agents has driven scientific discovery for \>100 years. There are very few magic bullets\* with high benefits and very low risk. The challenge is to identify, test, approve and ultimately use drugs that ***maximize efficacy but minimize toxicity***. The fundamental anchor on which the study of medicine is based is ***Primum non nocere: "*First do no harm"** Because of problems (including deaths) that have occurred, governments use approval processes (which tend to emphasize safety\>efficacy) before drugs can be marketed and prescribed. **Pharmacodynamics** **Pharmacodynamics** (also described as what a drug does to the body) is the study of the biochemical, physiologic, and molecular effects of drugs on the body, and involves receptor binding (including receptor sensitivity), post-receptor effects, and chemical interactions. The pharmacologic response depends on the drug binding to its target. The concentration of the drug at the receptor site influences the drug's effect. A drug's pharmacodynamics can be affected by physiologic changes due to a) a disease, or a disorder b) aging, c) other drugs. **Disorders that affect pharmacodynamic responses** include genetic mutations, thyrotoxicosis, malnutrition, myasthenia gravis, Parkinson disease, and some forms of insulin-resistant diabetes mellitus. These disorders can change receptor-binding, alter the level of binding proteins, or decrease receptor sensitivity. Aging tends to affect pharmacodynamic responses through alterations in receptor binding or in postreceptor response sensitivity). Pharmacodynamic drug--drug interactions result in competition for receptor binding sites, or alter postreceptor response. **Drug receptors** When drugs enter the body, they get distributed in such a way that they get to their site of action; which in most cases is a macromolecular entity called a **receptor**. **Receptors** are located in the target tissue or organ. Receptors are macromolecules involved in chemical signalling between and within cells; and they may be located on the cell surface membrane, nucleus or within the cytoplasm. For a drug to exert an effect; a number of them bind to specific receptors on the surface or interior of cells. However, many other cellular components and non-specific sites exist which serve as sites of drug action; unusual targets of drug action include; 1) **Water:** as observed in osmotic diuretics like mannitol which are not reabsorbed by the kidney, hence creating an osmotic load within the in the renal tubule which result in the obligate excretion of water. Also laxatives like magnesium sulphate act in the intestines by using the same principle. 2) **Hydrogen ions:** ammonium chloride is sometimes used to acidify the urine. When administered orally, the liver metabolizes ammonium ion to urea, while the chloride (Cl) is excreted in the urine. The loss of Cl;^-^results in an obligatory loss of H^+^ in the urine, thus lowering pH. 3) **Metal ions:** chelating agents like **ethylenediaminetetraacetic acid (**EDTA) may be used to bind divalent cations like lead (Pb^2+^). Metal ions are also drug targets in cases of poisoning. 4) **Enzymes** also serve as targets for many therapeutically useful drugs. Drugs may inhibit enzymes by via **competitive, non-competitive, or irreversible** blockade at a **substrate** or **cofactor** binding site. Digitalis glycosides increase myocardial contractility by inhibiting the membrane enzyme, **Na^+^-K^+^-ATPase**. Antimicrobial and antineoplastic drugs commonly work by inhibiting enzymes which are critical to the functioning of the cell. In order to be effective, these drugs must have at least some **selective toxicity** toward bacterial or tumour cells. This usually means that there is a unique metabolic pathway in these tumour cells or some difference in enzyme selectivity for a common metabolic pathway. An example of this is the inhibition of folate synthesis by sulfonamides. These drugs are effective antibacterial agents because the bacteria depend upon folate synthesis, while the host doesn\'t. 5) **Nucleic acids** are targets for antimetabolites and some antibiotics. In the case of 5fluorouracil, the compound acts as a counterfeit substitute for uracil and becomes incorporated into a faulty mRNA. 6) Some drugs, like general anaesthetics, appear to act by **non-specific binding to a macromolecular receptor** target. These drugs are thought to alter the function of membrane proteins, in part, by disorganising the structure of the surrounding lipid membranes. **Drug Receptor interactions** **Drug-receptor bonds** They are of 3 major types: a) covalent b) electrostatic and c) hydrophobic. **Covalent bonds** are very strong bonds, that are not readily broken. An example of a drug that uses a covalent mechanism of action is aspirin, which forms a covalent bond with its target enzyme, cyclooxygenase. Aspirin works in two ways; as an analgesic for pain relief and anti-inflammation, by preventing production of the cyclooxygenase produced substance, prostaglandins. An anti-clotting agent, or blood "thinner," by preventing the production of thromboxane A2, another cyclooxygenase produced substance. The logic behind covalently bound drugs is their duration of action. The only way to reverse the effects of aspirin would be to synthesize new enzymes, in new platelets, a process that would take several days to complete. **Electrostatic bonds** are much more common type of bond in drug-receptor interactions, and are weaker than the covalent bonds. They can either be: relatively strong ***ionic linkages*** between permanently charged molecules (eg. electrostatic interaction between Na+ and Cl-), weaker ***hydrogen bonds*** that occur in highly polar molecules, and very weak induced-dipole interactions such as ***Van Der Waals forces***. **Hydrophobic** interactions referred to interactions between molecules in which the interactions are less driven by molecule to molecule attraction, and more by the tendency of molecules to wish to avoid the aqueous (water) environments. These bonds are quite weak and are usually found in the interactions between highly lipid-soluble drugs and lipids in the cell membranes. **Receptor activation** **Activated receptors** directly or indirectly regulate cellular biochemical processes (these are post-receptor events e g, ion conductance, protein phosphorylation, DNA transcription, enzymatic activation/inhibition). Molecules (eg, drugs, hormones, neurotransmitters) that bind to a receptor are called **ligands**. The binding can be specific and reversible. A ligand may activate or inactivate a receptor; activation may increase or decrease a particular cell function. Each ligand may interact with multiple receptor subtypes. Few, if any drugs are absolutely specific for one receptor or subtype; but most have relative selectivity. **Selectivity** is the degree to which a drug acts on a given site relative to other sites; selectivity relates largely to physicochemical binding of the drug to cellular receptors. A drug's ability to affect a given receptor is related to **the drug's affinity** (probability of the drug occupying a receptor at any given instant) and **intrinsic efficacy or activity** (degree to which a ligand activates receptors and leads to cellular response). A drug's affinity and activity are determined by its chemical structure. The pharmacologic effect is also determined by the duration of time that the **drug-receptor complex persists** (residence time). The lifetime of the drug-receptor complex is affected by **dynamic processes** (**conformational changes**) that control the rate of drug association and dissociation from the target. A longer residence time explains a prolonged pharmacologic effect. Drugs with long residence times include finasteride and darunavir. A longer residence time can be a potential disadvantage when it prolongs a drug\'s toxicity. For some receptors, transient drug occupancy produces the desired pharmacologic effect, whereas prolonged occupancy causes toxicity. Physiologic functions (eg, contraction, secretion) are usually regulated by multiple receptor-mediated mechanisms, and several steps (eg, receptor-coupling, multiple intracellular 2nd messenger substances) may be interposed between the initial molecular drug--receptor interaction and ultimate tissue or organ response. Thus, several dissimilar drug molecules can often be used to produce the same desired response. The ability of a drug to bind to a receptor is influenced by **external factors** as well as by **intracellular regulatory mechanisms**. Baseline receptor density and the efficiency of stimulus-response mechanisms vary from tissue to tissue. Drugs, aging, genetic mutations, and disorders can increase (upregulate) or decrease (downregulate) the number and binding affinity of receptors. For example, clonidine downregulates alpha~2~-receptors; thus, rapid withdrawal of clonidine can cause hypertensive crisis. Chronic therapy with beta-blockers upregulates beta-receptor density; thus, severe hypertension or tachycardia can result from abrupt withdrawal. Receptor **up-regulation and down-regulation affects adaptation** to drugs (eg, desensitization, tachyphylaxis, tolerance, acquired resistance, post withdrawal supersensitivity). Ligands bind to precise molecular regions, called **recognition sites**, on receptor macromolecules. The binding site for a drug may be the same as or different from that of an endogenous agonist (hormone or neurotransmitter). Agonists that bind to an adjacent site or a different site on a receptor are sometimes called **allosteric agonists**. Nonspecific drug binding also occurs---i.e, at molecular sites not designated as receptors (eg, plasma proteins). Drug binding to such nonspecific sites, such as binding to serum proteins, prohibits the drug from binding to the receptor and thus inactivates the drug. Unbound drug is available to bind to receptors and thus have an effect. **Types of Drug-Receptor Interactions** **Agonists** Agonists activate receptors to produce the desired response. **Conventional agonists** increase the proportion of activated receptors. **Inverse agonists** stabilize the receptor in its inactive conformation and act similarly to competitive antagonists. Many hormones, neurotransmitters such as acetylcholine, histamine, norepinephrine and drugs like morphine, phenylephrine, isoproterenol, benzodiazepines and barbiturates act as agonists. Antagonists prevent receptor activation. Preventing activation has many effects. Antagonists **increase cellular function** if they block the action of a substance that normally decreases cellular function. Antagonists **decrease cellular function** if they block the action of a substance that normally increases cellular function. **Antagonists** Receptor antagonists can be classified as reversible or irreversible. **Reversible antagonists** readily dissociate from their receptor; **irreversible antagonists** form a stable, permanent or nearly permanent chemical bond with their receptor (eg, by alkylation). **Pseudo-irreversible** antagonists slowly dissociate from their receptor. In **competitive antagonism,** binding of the antagonist to the receptor prevents binding of the agonist to the receptor. In **non competitive antagonism,** agonist and antagonist can be bound simultaneously, but antagonist binding reduces or prevents the action of the agonist. In **reversible competitive antagonism,** agonist and antagonist form short-lasting bonds with the receptor, and a steady state among agonist, antagonist, and receptor is reached. Such antagonism can be overcome by increasing the concentration of the agonist. For example, naloxone (an opioid receptor antagonist that is structurally similar to morphine), when given shortly before or after morphine, blocks morphine's effects. However, competitive antagonism by naloxone can be overcome by giving more morphine. **Structural analogs of agonist molecules** frequently have agonist and antagonist properties; such drugs are called **partial (low-efficacy) agonists, or agonist-antagonists**. For example, pentazocine activates opioid receptors but blocks their activation by other opioids. Thus, pentazocine provides opioid effects but blunts the effects of another opioid if the opioid is given while pentazocine is still bound. A drug that acts as a partial agonist in one tissue may act as a full agonist in another. **Dose-response relationships** The **dose--response relationship**, or **exposure--response relationship**, describes the change in effect on a living [organism](https://en.wikipedia.org/wiki/Organism) caused by different degrees of exposure to a substance or different doses of a drug following a defined period of exposure. This dose response relation- ships vary with individuals and with period of exposure e.g. a small dose may have significant effect, while a large dose may be fatal. Although dose and exposure are often interchangeably used, in the area of [**clinical pharmacology**](https://en.wikipedia.org/wiki/Clinical_pharmacology) (which is the study of drugs as they relate to the management of diseases), the two names are separated. **Dose** refers to the quantity of a drug administered to an individual; however, **exposure** refers to the time-dependent concentration (often in reference to blood or plasma) or concentration-derived parameters such as AUC (area under the concentration curve) and C**~max~** (peak level of the concentration curve) of the drug after its administration. The study of dose response, and the science of developing dose--response models, is central to determining \"safe\", \"hazardous\" and (where relevant) beneficial levels and dosages for drugs, pollutants, foods, and other substances to which humans or other [organisms](https://en.wikipedia.org/wiki/Organism) are exposed. Dose--response relationships generally depend on the exposure time and exposure route (e.g., inhalation, dietary intake); quantifying the response after a different exposure time or for a different route leads to a different relationship and possibly different conclusions on the effects of the drug or chemical under consideration. This limitation is caused by the complexity of biological systems and the often unknown biological processes operating between the external exposure and the adverse cellular or tissue response. **Dose-response curves** Data from **dose-response** relationships are presented in the forms of graphs called **dose-response curves**. A **dose--response curve** is a simple X--Y graph that depicts the relationship between the magnitude of a substance or stressor (e.g. concentration of a toxicant, amount of a drug, temperature, intensity of radiation) and the response of the receptor, usually in an organism or population being studied. The response observed may be a physiological or biochemical response, or even death (mortality); and thus the data generated may be counts (or proportion, e.g., mortality rate), ordered descriptive categories (e.g., severity of a lesion), or continuous measurements (e.g., blood pressure). A number of effects or endpoints can be studied, often at different organizational levels (e.g., population, whole animal, tissue, cell). In clinical pharmacology, the measured dose (usually in milligrams, micrograms, or grams per kilogram of body-weight for oral exposures or milligrams per cubic meter of ambient air for inhalation exposures) is generally plotted on the X axis while the **measured effect** (response)is plotted on the y-axis. Other dose units include moles per body-weight, moles per animal, and for dermal exposure, moles per square centimetre. In some cases, it is the logarithm of the dose (Log~10~) that is plotted on the X axis, and in such cases the curve is typically S shaped also known as sigmoidal, with the steepest portion in the middle. Biologically based models using dose are preferred over the use of log (dose) because the logarithm can visually imply a threshold dose when in fact there is none. Because a drug effect is a function of dose and time, such a graph depicts the dose-response relationship independent of time. Measured effects are frequently recorded as maximal at time of peak effect or under steady-state conditions (eg, during continuous IV infusion). The response to a drug may also be quantified at different organisational levels. The first point along the graph where a response above zero (or above the control response) is reached is usually referred to as a threshold-dose. At higher doses, undesired side effects appear and grow stronger as the dose increases. The more potent a particular substance is, the steeper this curve will be. In quantitative situations, the Y-axis often is designated by percentages, which refer to the percentage of exposed individuals registering a standard response (which may be death, as in LD~50~). Such a curve is referred to as a **quantal dose-response curve**, distinguishing it from a **graded dose-response curve**, where response is continuous (either measured, or by judgment). A dose-response curve has features that vary according to a) potency (location of curve along the dose axis), b) maximal efficacy or ceiling effect (greatest attainable response) and c) slope (change in response per unit dose) Biologic variation (variation in magnitude of response among test subjects in the same population given the same dose of drug) also occurs. Dose-response curves of drugs studied under identical or standard conditions can help compare the pharmacologic profiles of the drugs. https://upload.wikimedia.org/wikipedia/commons/thumb/7/70/DoseResponse000.svg/320px-DoseResponse000.svg.png **Figure 2.1:** Semi-log plots of two agonists with different Kd; the blue curve indicates a more potent agonist than the green curve (higher response for the same dose). **Uses of information from a dose-response curve** This information deduced from a dose-response curve helps determine the dose necessary to achieve the desired effect. Dose-response, which involves the principles of pharmacokinetics and pharmacodynamics, determines the required dose and frequency as well as the therapeutic index for a drug in a population. **The therapeutic index** (ratio of the minimum toxic concentration to the median effective concentration) helps determine the efficacy and safety of a drug. Increasing the dose of a drug with a small therapeutic index increases the probability of toxicity or ineffectiveness of the drug. However, these features differ by population and are affected by patient-related factors, such as pregnancy, age, and organ function (eg, estimated glomerular filtration rate (GFR); kidney function is assessed by the measured or estimated GFR). **Pharmacokinetics** **Pharmacokinetics** answers the question about what the body does to a drug, or the fate of a drug within the body. Both pharmacokinetics and pharmacodynamics help to explain the relationship between the dose and **response**, which is the drug\'s effects. **Routes of drug administration** Before drugs can be absorbed, they must first be administered; and while most drugs can be administered by different routes, drug- and patient-related factors determine the selection of routes for drug administration. The factors are: **1)** Characteristics of the drug. **2)** Emergency/routine use. **3)** Site of action of the drug---local or systemic. **4)** Condition of the patient (unconscious, vomiting, diarrhoea). **5)** Age of the patient. **6)** Effect of gastric pH, digestive enzymes and first-pass metabolism. **7)** Patient's/doctor's choice (sometimes) **1.) Local routes:** It is the simplest mode of administration of a drug at the site where the desired action is required. Systemic side effects are minimal. **i) Topical:** Drug is applied to the *skin* or *mucous membrane* at various sites for local action. **a)** ***Oral cavity**:* as a suspension, e.g. nystatin; as a troche which are small **lozenges** that dissolve between the cheek and gum over a period of about 30 minutes. As it dissolves, the drug e.g. clotrimazole (for oral candidiasis) is gradually absorbed into the blood stream; as a cream that could be applied to the lips acyclovir (for herpes labialis); as ointment and jelly, e.g. 5% lignocaine hydrochloride (for topical anaesthesia); as a spray, e.g. 10% lignocaine hydrochloride (for topical anaesthesia). **b) *GI tract:*** As tablet that is not absorbed, e.g. neomycin (for sterilization of gut before surgery). **c)** ***Rectum and anal canal**:* **i) *Enemas*** which entails administration of drug into the rectum in liquid form; an evacuant enema is used for evacuation of bowel example include the soap water enema (soap acts as a lubricant and water stimulates the rectum). Retention enema involves injecting a solution into the rectum and holding for a specific period of time an example is the use of methylprednisolone in the treatment of ulcerative colitis. **ii. *Suppository*** which involves the administration of the drug in a solid form into the rectum, e.g. bisacodyl for evacuation of bowels. **d)** ***Eye, ear and nose**:* As drops, ointments and sprays (for infection, allergic conditions, etc.), e.g. gentamicin eye/ear drops. e) ***Bronchi***: As inhalation, e.g. salbutamol, ipratropium bromide, etc. (for bronchial asthma and chronic obstructive pulmonary disease). **f) *Skin***: As ointment, cream, lotion or powder, e.g. clotrimazole (antifungal) for cutaneous candidiasis. **ii) Intra-arterial route:** This route is rarely employed. It is mainly used during diagnostic studies such as coronary angiography and for the administration of some anticancer drugs, e.g. for treatment of malignancy involving limbs. **iii.) Administration of the drug into some deep tissues** by injection, e.g. administration of triamcinolone directly into the joint space in rheumatoid arthritis. **b.) Systemic Routes** Drugs administered by this route enter the blood and produce systemic effects. **Enteral Routes:** It includes oral, sublingual and rectal routes**. a)** **Oral Route:** It is the most common and acceptable route for drug administration. Dosage forms are tablet, capsule, syrup, mixture, etc., e.g., paracetamol tablet for fever, omeprazole capsule for peptic ulcer are given orally. Its advantages are that it is safer, cheaper, painless, and convenient for repeated and prolonged use and that it can be self-administered. The *disadvantages include its n*ot being suitable for emergency as onset of action of orally administered drugs is slow. It is not suitable for use in unpalatable and highly irritant drugs, unabsorbable drugs (e.g. aminoglycosides), drugs that are destroyed by digestive juices (e.g. insulin), drugs with extensive first-pass metabolism (e.g. lignocaine). The oral route of administration is also not suitable for use in unconscious patients, uncooperative and unreliable patients and patients with severe vomiting and diarrhoea. **b) Sublingual Route:** The preparation is kept under the tongue. The drug is absorbed through the buccal mucous membrane and enters the systemic circulation directly, e.g. nitroglycerin for acute anginal attack and buprenorphine for myocardial infarction.. Its advantages include quick onset of action, drug action can be terminated by spitting out the tablet, it bypasses first-pass metabolism and self-administration is possible. *Disadvantages are that it* is not suitable for use with drugs that are irritant and lipid-insoluble, as well as drugs with bad smell and taste. **c)** **Rectal Route** Drugs can be given in the form of solid or liquid. 1. **Suppository:** It can be used for local (topical) effect (as mentioned above) as well as systemic effect, e.g. indomethacin for rheumatoid arthritis. 2. **Enema:** *Retention enema* can be used for local effect (as above) as well as systemic effect. The drug is absorbed through rectal mucous membrane and produces systemic effect, e.g. diazepam for status epilepticus in children. **Parenteral Routes. Routes** of administration other than enteral route are called parenteral routes. *Advantages include* short onset of action, hence it is suitable for emergency and also useful in: patients who are unconscious, uncooperative, and unreliable, are vomiting and/or have diarrhoea. It is suitable for use in drugs that are irritant, have high first-pass metabolism, drugs not absorbed orally. And drugs destroyed by digestive juices. *Disadvantages include the need for* aseptic conditions; preparations should be sterile making these drugs expensive. They also requires invasive techniques that are painful. cannot be usually self-administered and can cause local tissue injury to nerves, vessels, etc. **Inhalation** Volatile liquids and gases are given by inhalation for systemic effects, e.g. general anaesthetics. *Advantages include q*uick onset of action, dose required is usually less, so systemic toxicity is minimized, quantity of drug administered can be regulated. *Disadvantages include l*ocal irritation which may cause increased respiratory secretions and bronchospasm. **Injections a)** **Intradermal route:** The drug is injected into the layers of the skin, e.g. Bacillus Calmette--Guerin (BCG) vaccination and drug sensitivity tests. It is painful and only a small amount of the drug can be administered. **b)** **Subcutaneous (s.c.) route:** The drug is injected into the subcutaneous tissues of the thigh, abdomen and arm, e.g. adrenaline, insulin, etc. *Advantages include the possibility of s s*elf-administration (e.g. insulin), depot preparations can be inserted into the subcutaneous tissue, e.g. norplant for contraception. *Disadvantages are i)* It is suitable only for nonirritant drugs; ii) drug absorption is slow; hence it is not suitable for emergency, **c) Intramuscular (i.m.) route:** Drugs are injected into large muscles such as deltoid, gluteus maximus and vastus lateralis, e.g. paracetamol, diclofenac, etc. A volume of 5--10 mL can be given at a time. *Advantages are: a)a*bsorption is more rapid compared to oral route, b) mild irritants, depot injections, soluble substances and suspensions can be given by this route. *Disadvantages are a) a*septic conditions are needed, b) intramuscular injections are painful and may cause abscess, c) self-administration is not easy, d) there may be nerve injury, **d)** **Intravenous (i.v.) route:** Drugs are injected directly into the blood stream through a vein. Drugs are administered as: i) ***Bolus*:** Single, relatively large dose of a drug injected rapidly or slowly as a single unit into a vein. e.g. i.v. ranitidine in bleeding peptic ulcer, ii) ***Slow intravenous injection*:** e.g., i.v. morphine in myocardial infarction, iii) ***Intravenous infusion*:** e.g. dopamine infusion in cardiogenic shock; mannitol infusion in cerebral oedema; fluids infused intravenously in dehydration. *Advantages are a) b*ioavailability is 100%, b) quick onset of action; therefore, it is the route of choice in emergency, e.g. intravenous diazepam to control convulsions in status epilepticus, c) large volume of fluid can be administered, e.g. intravenous fluids in patients with severe dehydration, d) highly irritant drugs, e.g. anticancer drugs can be given because they get diluted in blood, e) hypertonic solution can be infused by intravenous route, e.g. 20% mannitol in cerebral oedema. C) By i.v. infusion, a constant plasma level of the drug can be maintained, e.g. dopamine infusion in cardiogenic shock. *Disadvantages are: a) o*nce the drug is injected, its action cannot be halted, b) local irritation may cause **phlebitis**, c) self-medication is difficult) strict aseptic conditions are needed, d) extravasation of some drugs can cause injury, necrosis and sloughing of tissues, e) depot preparations cannot be given by i.v. route. *Precautions that need to be taken include a) d*rug should usually be injected slowly, b) Before injecting, make sure that the tip of the needle is in the vein. **Absorption of Drugs** **Drug absorption** is the movement of a drug into the bloodstream following administration. Drug absorption is determined by the drug's physicochemical properties, formulation, and route of administration. Dosage forms such as tablets, capsules, solutions, which is made up of the drug and other ingredients, are formulated to be administered by various routes (oral, buccal, sublingual, rectal, parenteral, topical, inhalational). Inspite of the route of administration, drugs must be in solution to be absorbed. Thus, solid forms like tablets must be able to disintegrate and deaggregate. Unless administered intravenously (IV), a drug must also cross several semipermeable cell membranes before it reaches the systemic circulation. Absorption of drugs also affects its bioavailability, which is how quickly and how much of a drug reaches its intended target (site) of action. **Transport across cell membranes:** Cell membranes are biologic barriers that selectively inhibit passage of drug molecules. The membranes are composed primarily of a bimolecular lipid matrix, which determines membrane permeability characteristics. Drugs may cross cell membranes by; a) passive diffusion, b) facilitated passive diffusion, c) active transport, d) pinocytosis. At times various globular proteins embedded in the matrix function as receptors and help transport molecules across the membrane **Passive mechanisms** involve the movement of molecules across the cell membrane and does not require energy. It is dependent on the permeability of the cell membrane. There are three main kinds of passive transport; diffusion, osmosis and facilitated diffusion. **Active mechanisms** require the cell to use energy, usually in the form of ATP. It creates a charge gradient in the cell membrane. e.g. in the mitochondrion, hydrogen ion pumps pump hydrogen ions into the inter-membrane space of the organelle as part of making ATP. Active transport is **selective, requires energy expenditure, and may involve transport against a concentration gradient**. Active transport seems to be limited to drugs structurally similar to endogenous substances (eg, ions, vitamins, sugars, amino acids). These drugs are usually absorbed from specific sites in the small intestine. **Passive diffusion :** Drugs diffuse across a cell membrane from a region of high concentration (GI fluids) to one of low concentration (blood). Diffusion rate is directly proportional to the gradient but also depends on the molecule's lipid solubility, size, degree of ionization, and the area of the absorptive surface. Because the cell membrane is composed of lid rich membranes (lipoid), lipid-soluble drugs diffuse rapidly. Small molecules tend to penetrate membranes more rapidly than larger ones. Majority of the drugs available for use are weak organic acids or bases, that exist in un-ionized and ionized forms in an aqueous environment. The un-ionized form is usually lipid soluble (lipophilic) and diffuses readily across cell membranes. The ionized form has low lipid solubility (but high water solubility---ie, hydrophilic) and high electrical resistance and thus cannot penetrate cell membranes easily. The proportion of the un-ionized form present (and thus the drug's ability to cross a membrane) is determined by the environmental pH and the drug's p*K*~a~ (acid dissociation constant). The p*K*~a~ is the pH at which concentrations of ionized and un-ionized forms are equal. When the pH is lower than the p*K*~a~, the un-ionized form of a weak acid predominates, but the ionized form of a weak base predominates. Thus, in plasma (pH 7.4), the ratio of un-ionized to ionized forms for a weak acid (eg, with a p*K*~a~ of 4.4) is 1:1000; in gastric fluid (pH 1.4), the ratio is reversed (1000:1). Therefore, when a weak acid is given orally, most of the drug in the stomach is un-ionized, favouring diffusion through the gastric mucosa. For a weak base with a p*K*~a~ of 4.4, the outcome is reversed; most of the drug in the stomach is ionized. Theoretically, weakly acidic drugs (eg, aspirin) are more readily absorbed from an acid medium (stomach) than are weakly basic drugs (eg, quinidine). However, whether a drug is acidic or basic, most absorption occurs in the small intestine because the surface area is larger and membranes are more permeable. **Facilitated passive diffusion:** Certain molecules with low lipid solubility like glucose penetrate membranes more rapidly than expected. How this occurs has been explained using facilitated passive diffusion which is a carrier-mediated transport system. A **carrier molecule** in the membrane combines reversibly with the substrate molecule outside the cell membrane, and the carrier-substrate complex diffuses rapidly across the membrane, releasing the substrate at the interior or internal surface of the membrane. In such cases, the membrane transports only substrates with a relatively specific molecular configuration, and the availability of carriers limits the process. The process does not require energy expenditure, and transport against a concentration gradient cannot occur. **Active transport:** This is drug passage that is facilitated by an energy-dependent membrane carrier mechanism such that transport can occur against a concentration gradient; transporters include the family of ATP-dependent proteins, It exhibits structural selectivity and it is saturable, It also obeys Michaelis-Menten kinetics: if drug concentration is high enough to saturate carrier mechanism, kinetics are zero-order (rate of transport is constant). **Exocytosis/ Pinocytosis:** In pinocytosis which like active transport requires energy expenditure, fluid or particles are engulfed by a cell. The cell membrane invaginates, encloses the fluid or particles, then fuses again, forming a vesicle that later detaches and moves to the cell interior. Pinocytosis probably plays a small role in drug transport, except for protein drugs. **Factors responsible for the transfer of drugs across membranes** The absorption, distribution, metabolism, and excretion of a drug all involve its passage across cell membranes. Mechanisms by which drugs cross membranes and the physicochemical properties of molecules and membranes that influence this transfer are important for understanding the disposition of drugs in the human body. The characteristics of a drug that predict its movement and availability at sites of action are its molecular size and shape, degree of ionization, relative lipid solubility of its ionized and nonionized forms, and its binding to serum and tissue proteins. In most cases, a drug must traverse the plasma membranes of many cells to reach its site of action. Although barriers to drug movement may be a single layer of cells (intestinal epithelium) or several layers of cells and associated extracellular protein (skin), the plasma membrane represents the common barrier to drug distribution. **The plasma membrane** consists of a bilayer of amphipathic lipids with their hydrocarbon chains oriented inward to the centre of the bilayer to form a continuous hydrophobic phase and their hydrophilic heads oriented outward. Individual lipid molecules in the bilayer vary according to the particular membrane and can move laterally and organize themselves with cholesterol (*e.g.,* sphingolipids), endowing the membrane with fluidity, flexibility, organization, high electrical resistance, and relative impermeability to highly polar molecules. Membrane proteins embedded in the bilayer serve as receptors, ion channels, or transporters to transduce electrical or chemical signalling pathways and provide selective targets for drug actions. These proteins may be associated with caveolin and sequestered within caveolae, they may be excluded from caveolae, or they may be organized in signalling domains rich in cholesterol and sphingolipid not containing caveolin. **Passive membrane transport:** Drugs cross membranes either by passive processes or by mechanisms involving the active participation of components of the membrane. In passive transport, the drug molecule usually penetrates by diffusion along a concentration gradient by virtue of its solubility in the lipid bilayer. Such transfer is directly proportional to the magnitude of the concentration gradient across the membrane, to the lipid--water partition coefficient of the drug, and to the membrane surface area exposed to the drug. The greater the partition coefficient, the higher is the concentration of drug in the membrane, and the faster is its diffusion. After a steady state is attained, the concentration of the unbound drug is the same on both sides of the membrane if the drug is a nonelectrolyte. For ionic compounds, the steady-state concentrations depend on the electrochemical gradient for the ion and on differences in pH across the membrane, which may influence the state of ionization of the molecule disparately on either side of the membrane. **Weak Electrolytes and Influence of pH:** Most drugs are weak acids or bases that are present in solution as both the non-ionized and ionized species. The non-ionized molecules usually are more lipid-soluble and can diffuse readily across the cell membrane. In contrast, the ionized molecules usually are unable to penetrate the lipid membrane because of their low lipid solubility. Therefore the transmembrane distribution of a weak electrolyte is determined by its p*K*~a~ and the pH gradient across the membrane. The p*K*~a~ is the pH at which half the drug (weak electrolyte) is in its ionized form. To illustrate the effect of pH on distribution of drugs, the partitioning of a weak acid (p*K*~a~ = 4.4) between plasma (pH = 7.4) and gastric juice (pH = 1.4) is depicted in Figure 1--2. It is assumed that the gastric mucosal membrane behaves as a simple lipid barrier that is permeable only to the lipid-soluble, non-ionized form of the acid. The ratio of non-ionized to ionized drug at each pH is readily calculated from the Henderson--Hasselbalch equation: \ [**log** **(**protonated**)/(**unprotonated**)** **=** pK**a** **−** pH]{.math.display}\ **Factors that influence diffusion across cellular membranes** In general terms, diffusion can be usefully described by Fick\'s First Law of Diffusion, which states that *\"the molar flux due to diffusion is proportional to the concentration gradient\".* *\ *[**J=D** **×**dφ**÷**dx ]{.math.inline} *where J is \"diffusive flux\", the magnitude and direction of the flow of a substance from one compartment to another; dφ is the concentration difference and dx is the distance for diffusion (or the thickness of the membrane).* This distance variable is present in the one-dimensional application of the equation, and more clinically relevant models end up also including the surface area. D is a *diffusion coefficient* which is influenced by solution temperature, viscosity of the fluid, and the size of the molecules. **Diffusion through lipid and aqueous solutions are slightly different,** depending on drug properties. Specifically, the pH and pKa of the drug will influence the lipid-water partition coefficient of a drug. The higher the partition coefficient, the more drug can cross the membrane. The pKa and pH interplay also determines whether or not the drug will be ionised, and therefore whether it will be influenced by electrochemical gradients on top of concentration gradients. Because the viscosity of human extracellular fluid is fairly stable and the temperature does not fluctuate by more than a couple of degrees, we can now narrow down the list of things which influence drug diffusion to a short list:1) Drug molecule size and shape 2) Concentration gradient 3) Membrane thickness, 4) Surface area of the membrane 5) pKa of the drug, and 6) pH of the solution. **Concept of first pass metabolism** If a drug is injected directly into the bloodstream, all of it (100%) is available for immediate distribution to tissues. However, this is not usually the case for other modes of administration; for example, following oral drug administration which involves absorption via the portal circulation, the drug must **first pass** through the liver (the primary site of drug metabolism- **biotransformation**), in the process some of the drug may therefore be metabolized before it reaches the systemic blood, this is known as **\"first-pass\" metabolism; a process which** reduces the **amount of drug available (bioavailability) for distribution to tissues to** less than 100%. **Bioavailability** is defined as the percentage of administered drug that reaches the systemic circulation. Once the drug enters into the bloodstream, a portion of it may exist as **free drug** (dissolved in plasma water), some of the drug will be reversibly **taken up by red cells** and some will be **reversibly** **bound to plasma proteins**. For many drugs, the bound forms can account for 95-98% of the total amount of drugs in circulation. This is important because it is the free drug which traverses cell membranes and produces the effect. It is also important because protein-bound drug can act as a reservoir which releases drug slowly, and thus prolongs its action of drugs. The **unbound or free drug** usually follows its concentration gradient in entering into peripheral tissues **(drug distribution)**. In a number of cases, the tissue contains the target site for drug action (receptor); while in others, the tissue is not affected by the drug. Sites of non-specific binding also act as further reservoirs for the drug. The **volume of distribution** determines the **equilibrium concentration** of drug after a specified dose. Tissue-bound drug eventually re-enters the circulation. **Drug distribution** Once a drug enters into the systemic circulation (by **absorption** or **direct administration**), it is distributed into interstitial and intracellular fluids. Each organ or tissue can receive different quantities of the drug and the drug can remain in the different organs or tissues for varying amounts of time. The distribution of a drug between tissues is dependent on a) **vascular permeability**, b) **regional blood flow**, c) **cardiac output and perfusion rate of the tissue**, d) **the ability of the drug to bind tissue and plasma proteins**, e) **lipid solubility** f) **pH partition -**which is the tendency for acids to accumulate in basic fluid compartments, and bases to accumulate in acidic compartments also plays an important role in drug distribution (electric charge is known to decrease the membrane permeability of substances, so once an acid enters a basic fluid and becomes electrically-charged, then it cannot escape that compartment with ease and therefore accumulates, and vice versa with bases). Drugs are easily distributed in highly-perfused organs like the liver, heart and kidney, and distributed in small quantities in less perfused tissues like muscle, fat and peripheral organs. Drugs can also be moved from the plasma to the tissue until the equilibrium is established (for unbound drug present in plasma). The **volume of distribution** (V~D~), which is also known as **apparent volume of distribution,** describes the relationship that exists between concentration of a drug and the amount of drug in the body. It is defined as the distribution of a drug between plasma and the rest of the body after oral or parenteral administration. **The V~D~ of a drug represents the degree to which a drug is distributed within the tissues of the body rather than the plasma.** V~D~ correlates directly with the amount of drug distributed into tissue; a higher V~D~ indicates a greater amount of tissue distribution. The main factors that affect volume of distribution include a) an organism\'s physical volume, b) the removal or excretion rate of the drug and c) the degree to which a drug binds with plasma proteins and / or tissues. **Drug biotransformation or metabolism** Biotransformation means chemical alteration of chemicals such as nutrients, amino acids, toxins, and drugs in the body. The metabolism of a drug or toxin in a body is an example of a biotransformation process. The body typically deals with a foreign compound by making it more water-soluble, to increase the rate of its excretion through the urine. **Drugs can undergo one of four potential biotransformation** processes a) active drug to inactive metabolite, b) active drug to active metabolite, c) inactive drug to active metabolite, d) active drug to toxic metabolite. Biotransformation may sometimes produce metabolites with a great deal of activity. Occasionally, we administer a parent drug which is inactive (a pro-drug) and only the metabolite has activity. Sites of drug biotransformation include a) **liver which** contributes to both the pre-systemic and the systemic elimination of many drugs, b) **other tissues,** like the i) intestinal mucosa cells also contribute to the pre-systemic elimination of a number of drugs and ii) renal tubular cells, c) t**he colon, by bacteria** as observed in azo reduction and hydrolytic reactions. The pathways of drug biotransformation can be divided into: i) phase I and ii) phase II reactions **Phase І reactions** include oxidative, reductive, and hydrolytic reactions. In these types of reaction, a polar group is either introduced or unmasked; making the drug molecule **more water-soluble** allowing for excretion. Reactions in phase I are also non-synthetic and result in the production of less active metabolites. The majority of these metabolites are generated by a common hydroxylating enzyme system known as **Cytochrome P450**. **Phase II** reactions involve covalent attachment of small polar endogenous molecule like glucuronic acid, sulfate, or glycine to form water-soluble compounds; a process also known as a ***conjugation reaction*.** The majority of phase II reactions are catalysed by transferases. The final compounds have a larger molecular weight. **Drug excretion** Generally, the liver metabolizes most drugs into inactive or less active compounds which are more readily excreted. These metabolites and some of the parent compound may be excreted in the bile and eventually may pass out of the body in the faeces. Alternatively, some of the drug may be reabsorbed again, farther down the GI tract (**the enterohepatic cycle**). Parent drug and metabolites in the bloodstream may then be excreted: most are filtered by the kidney, where a portion undergoes reabsorption, and the remainder is excreted in the urine. Some drugs are actively secreted into the renal tubule. Another route of drug excretion is the lung: Drugs like alcohol and the anaesthetic gases are eliminated by this route. Smaller amounts of drug are eliminated in the sweat, tears and breast milk. **Concept of drug nomenclature** The term **drug nomenclature** implies the different names that can be used in drug identification. Generally drugs have three different names; 1) Chemical name 2) Non Proprietary name 3) Proprietary name. A **chemical name** is assigned usually when a new chemical entity (NCE) is developed. It is the name given to drug in accordance with rules of chemical nomenclature established by International Union of Pure and Applied Chemistry (IUPAC). It is useful for chemists or technical personnel as it provides the precise arrangement of atoms and atomic groups in the molecule. It is not used to identify the drug in a clinical or marketing situation. A **non proprietary** name is a short name given to a drug that is not subject to proprietary rights (rights relating to an owner or ownership). The non-proprietary name is usually concise and meaningful. There are two classes of non proprietary names; a) **approved name** which is the name given to a drug by bodies like United States Adopted Name Council (USAN) and British Approved Name (BAN), soon after its introduction. The **official name** is the name approved by the National Pharmacopeia Commission and included in the official book i.e. Pharmacopeia. The official name must be identical with approved name. A **proprietary name** is the name given to a drug by the pharmaceutical firm which sells the drug. Thus a single drug is sold under many proprietary names by different firms. They are written with capital initial letter and are often further distinguished by superscript R in circle ® Clinicians usually described drug by their proprietary names. An example is paracetamol whose **chemical name** is N-(4-hydroxyphenyl)acetamide, **non-proprietary name**: **a) approved Name**: British Approved Name (BAN) is paracetamol while the United States Adopted Name (USAN) is acetaminophen. Its **official name** is acetaminophen, while its **proprietary name**s include Panadol, Calpol, Adol or Tylenol. **Drug classification** **Drug classification** is essential because it allows several drugs to be reduced to manageable groups. There is homogenous system for classifying drugs that suits all purposes; drugs are generally classified depending to suit the different uses.or discussants for example a Chemist, Pharmacologist, Pharmacist or Clinician. In pharmacology and medicine in general drugs to allow for homogeneity drugs are classified using the [Anatomical Therapeutic Chemical Classification System](https://en.wikipedia.org/wiki/Anatomical_Therapeutic_Chemical_Classification_System) (ATC); which is the most widely used drug classification system; it assigns drugs a unique [ATC code](https://en.wikipedia.org/wiki/ATC_code). The ATC code is an alphanumeric code that assigns drugs to specific drug classes within the ATC system. Another major classification system is the [Biopharmaceutics Classification System](https://en.wikipedia.org/wiki/Biopharmaceutics_Classification_System) (BCS) which classifies drugs according to their solubility and permeability or [absorption](https://en.wikipedia.org/wiki/Absorption_(pharmacokinetics)) properties. **Drugs can be classified based on** a) [chemical structures](https://en.wikipedia.org/wiki/Chemical_structure) or nature, b) [mechanism of action](https://en.wikipedia.org/wiki/Mechanism_of_action) (for example the ability to bind to the same [biological target](https://en.wikipedia.org/wiki/Biological_target)), c) [mode of action](https://en.wikipedia.org/wiki/Mode_of_action), d) treatment of the same disease. 1) **Classification of drugs based on their chemical nature** divides drugs into a) **inorganic drugs** like metals and their Salts (Ferrous Sulphate, Zinc Sulphate, Magnesium Sulphate, non metals such as sulphur. b) **organic drugs** including i) Alkaloids like atropine, morphine, strychnine), ii) Glycosides such as digitoxin, digoxin), iii) Proteins like insulin, oxytocin) iv) **others** like esters, amide, alcohol, and glycerides. **2) Classification based on source** a) **natural** sources, for example drugs from i) **plants** like morphine, atropine, digitoxin ii**) animal**s like bovine Insulin, iii) **micro-organism** like penicillin b) **synthetic sources** like sulphonamide, c) **Semi-synthetic** sources like amoxicillin, ampicillin, doxycycline and d) Biosynthetic Sources like recombinant Human erythropiotin, Recombinant bovine somattotropine 3) **Classification of drugs based on the target organ** on which they act groups drugs into region such as a) **central nervous system** acting drugs like diazepam, phenobarbitone, b) drugs acting on the **respiratory System** like bromhexaine, c) drugs acting on **cardiovascular system** like digitoxin, digoxin, d) drugs acting on the **gastrointestinal system** like omeprazole, kaoline, e) Drugs acting on **Urinary System** like magnesium sulphate, lasix and f) drugs acting on **reproductive system** like oxytocin, oestrogen. 4) **Classification based on mode of action** for example **Inhibitors of bacterial cell wall synthesis** such as penicillin, **inhibitor of bacterial protein** **synthesis** like tetracycline or **calcium channel blockers** like verapamil or nifedipine. **5) Classification based on therapeutic use** groups drugs broadly based on their clinical effects or uses into antimicrobials/antibacterials (penicillin, streptomycin, quinolones, macrolides), antihypertensive (clonidine, hydralazine, enalpril), antidiarrheals (lopramide, kaoline), antiemetics (domperidone, meclizine and metoclopramide). **6) Classification based on physiological system** for example sympathomimetics like adrenaline, noradrenaline), parasympathomimetics such as carbachol, pilocarpine, neostigmine), neuromuscular blockers like suxamethonium, gallamine. **Characteristics of drugs** **Drugs can either be synthesized** within the body, in which case they are called *hormones*, or **chemically** synthesized outside the body. Drugs generally act on regulator molecules, known as **receptors**, which literally receives the agonist or antagonist molecule, and sends the signal to the body system it regulates, changing it to the liking of the agonist (activate) or antagonist (inhibit). For a drug to elicit a biological or physiological change it must have certain characteristics that make it appropriate for interacting with a receptor. ***Drugs that are artificially delivered must have the following characteristics to be effective pharmacologically***. **A)** The drug must have a very specific size, shape, atomic configuration and electrical charge to be able to interact with the receptor. **B)** drug must have the necessary properties to travel to its site of action or receptor from its site of administration. **C)** It must be easily inactivated or excreted from the body once it has been used for its purpose. **Physical and Chemical Nature of Drugs** Any drug given to the body can either be a solid (eg. aspirin), liquid (eg. ethanol) or gas (nitrous oxide). The physical nature of the drug determines how the drug is administered to the body. Furthermore, drugs administered can also be carbohydrates, lipids or proteins, and this also affects their route of administration. The addition of a simple chemical group exerts a large difference on the overall nature of the drug and its interactions with receptors and enzymes; an example is observed with acetylcholine, which is usually hydrolyzed by acetylcholinesterase. When a methyl or a CH3 group is added however, the resultant methacholine is much more resistant to the effect of acetylcholineesterase. Furthermore, if an amine group is substituted for a methyl group, then the resultant carbachol is completely resistant to the effects of acetylcholineesterase. **Drug Size** **The molecular size of a drug** can vary from very small like lithium ion, (MW of 7) to very large such as alteplase (MW 59,050). It is important to remember that a drug has to be sufficiently unique in order to have effects at receptors, which are very specific for size, charge, shape and atomic configuration. It is has been observed that a drug must be of a certain size to have sufficiently unique characteristics that allow it to bind to a receptor. This size is approximately 100MW. Thus, *the lower limit of drug size, 100MW*, is determined by the need for the drug to be sufficiently unique and interact with the receptor. **All drugs must be able** to undergo ***diffusion* to move from the compartment** in which they are administered to the **compartments in which they are needed**. If the drug size is too large, then there is no way for the drug to diffuse into compartments, and the ability to diffuse decreases. Small drugs are able to fit through the small pores and into compartments where they can be used. Larger drugs just can't fit. It has been reported that the *upper limit of drug size then, is determined by the need for the drug to be able to be transported or moved within the body*. Thus, drugs larger than 1000MW do not diffuse readily, and thus most drugs are below 1000MW. **Drugs larger than 1000MW** can still be used, but must be directly injected into the compartment where they act, so as to minimize the distance the drug must move, or be transported. An example is alteplase (MW 59,050) which is a clot dissolving drug that is injected directly to the vascular compartment by an intravenous or intra-arterial administration. In summary, the drug size must be high enough to be unique to a receptor (this determines the lower limit of drug size, 100 MW -- a drug ideally should not be lower than this), but must be low enough to still be able to move properly to the target cells (this determines the upper limit of drug size, 1000MW -- a drug ideally should not be higher than this, as the transport of the drug will be negatively impacted. **Drug shape** The shape of the drug is an important factor in defining the nature of the drug-receptor interaction. The three-dimensional shape of the drug is thought to interact with a complementary structural binding region of the receptor, typically a protein. The specific nature of the interaction defines whether the drug acts as an agonist promoting a change in cellular function or as an antagonist which blocks the receptor usually resulting in no direct biological effect. Conceptually, it is important to think of a receptor as a lock, and the enzyme as the key that "activates" the lock. For this to occur, the drug has to be a perfect shape to "fit" into the receptor. Chemically, a drug has to have a certain *configuration* that allows it to be chemically active and thus, react with a drug. Hence, the drug must be of a *specific shape that allows it to interact with the receptor.* ***Drug receptor shape*** ***Chirality (stereoisomerism)*** **Chiral refers to molecule** with a centre of three-dimensional asymmetry. Chirality or \"handedness\" originates from Greek word cheir meaning the hand; representing molecules which are not superimposable on their mirror image while achiral molecules can be superimposed on their mirror image. A chiral molecule has no plane of symmetry, and rotates plane-polarized light. All molecules are chiral when they have one stereogenic (asymmetric) carbon. Stereoisomer refers to isomers that have the substituent bonding pattern but have different 3D arrangement of the atoms. Stereoisomers may appear as **enantiomers or diastereomers.** Many drugs, (50%) are chiral (existing as enantiomeric pairs). Enantiomers (molecules having opposite shapes) are pairs of molecules existing in forms that are mirror images of each other (right-& left-hand) but that cannot be superimposed. Enantiomers are chemically identical, but may be distinguished by the direction in which they rotate polarized light, either dextro (d or +) or levo (l or -). The light rotational effect is determined in solution. Chemical identity is associated with **true enantiomers**, i.e. associated with a single asymmetric molecular centre. If the molecule has 2 asymmetric centers, the molecule is referred to as a diasteriomer. Diastereomers have differing arrangement of atoms in space. One example would be Cis-Trans isomers. The comparison between *cis*- and *trans*-2-butene is an example of a pair of diastereomers that do not have asymmetric carbon atoms. Drugs with two asymmetric centers have four diasteriomers (e.g., labetalol, trandate, normodyne): an alpha and beta-receptor antagonist). **U**sually one enantiomer will be more effective than its mirror structural image, suggesting a more complementary fit between the drug with its receptor binding region. Examples are observed with S(+)- methacholine enantiomer which is 250 times more potent than the R(-) enantiomer form. Ketamine (Ketalar): (+) enantiomer is more potent and less toxic then the (-) enantiomer; however, the drug is used as the racemate (includes both enantiomeric forms). **It's easier for a right hand to fit into a right sided glove, than the left hand.** The importance of chirality is observed with thalidomide which is has left and right-handed molecular forms. One form causes sedation, while the other form is responsible for foetal abnormalities. Although the primary therapeutic use for thalidomide today is in treatment of leprosy, in particular a disease complication called erythema nodosum leprosum, thalidomide appears effective in treating certain cancers. **Drug permeability** Permeability across biological membranes is a key factor in the absorption and distribution of drugs. Poor permeability can arise due to a number of structural features, as well as membrane-based efflux mechanisms. This can lead to poor absorption across the gastrointestinal mucosa (in orally administered drugs) or poor distribution throughout the body. The membrane lipid bilayer is the most important barrier for drug permeation since there are many lipid barriers separating body compartments a) Lipid: aqueous drug partition coefficients describes the ease with which a drug moves between aqueous and lipid environments b) Ionization state of the drug is an important factor: charged drugs diffuse-through lipid environments with difficulty. c) pH and the drug pKa **(dissociation or ionization constant which is the pH at which half of the substance is** **ionized and the other half unionized)** are important in determining the ionization state, and influence significantly transport (ratios of lipid-to aqueous-soluble forms for weak acids and bases described by the Henderson-Hasselbalch equation. \ [**log** **(**protonated**)/(**unprotonated**)** **=** pK**a** **--** pH]{.math.display}\ Uncharged forms are lipid-soluble while charged forms are soluble in aqueous solution and are not lipid soluble so they pass through biological membranes with difficulty. **Drug design** **Drug design**, also referred to as **rational drug design** is the [inventive](https://en.wikipedia.org/wiki/Invention) process of discovering new [medications](https://en.wikipedia.org/wiki/Medications) based on the knowledge of a [biological target](https://en.wikipedia.org/wiki/Biological_target). This drug would most likely be an [organic](https://en.wikipedia.org/wiki/Organic_compound) [small molecule](https://en.wikipedia.org/wiki/Small_molecule) that either activates or inhibits the function of the biological target which is usually a [biomolecule](https://en.wikipedia.org/wiki/Biomolecule) such as a [protein](https://en.wikipedia.org/wiki/Protein), the effect of this potential drug then results in a [therapeutic](https://en.wikipedia.org/wiki/Therapeutic_effect) benefit to the [patient](https://en.wikipedia.org/wiki/Patient). Drug design involves the design of molecules that are complementary in [shape](https://en.wikipedia.org/wiki/Shape) and [charge](https://en.wikipedia.org/wiki/Electric_charge) to the biomolecular target with which they interact and therefore will bind to it. Drug design frequently but not necessarily relies on [computer modelling](https://en.wikipedia.org/wiki/Molecular_modelling) techniques. When a computer--based modelling is used it is referred to as **computer-aided drug design**. Drug design may also rely on the knowledge of the three-dimensional structure of the biomolecular target in this case this is known as **structure-based drug design**. **General Pharmacology Practicals Topics** 1. **Introduction to pharmacology practicals** 2. **Routes of drug administration** 3. **Test of analgesia/acute toxicity** 4. **Animal ethics and good laboratory practice** **ANIMAL ETHICS AND GOOD LABORATORY PRACTICE** OBJECTIVES At the end of the session the student shall be able to : 1\. Realize the importance of using animals for pre-clinical testing 2\. Justify the need for adhering to proper standards of maintenance and care in the use of animals for research and teaching 3\. Understand the principles of good laboratory practice and its importance in the conduct of experiments. Visit to animal house or demonstration of the different types of animals used for laboratory studies Students in the Batch A discuss the use of animals in research and teaching. They are asked to select three speakers who will speak for five minutes each. Batch B students are asked to speak against the use of animals in research and teaching. They are asked to select three speakers who will speak for five minutes each. They are given 30 minutes preparation time. After the debate another 30 minutes are spent on open comments from students and a focussed group discussion. Important points to note in animal care: 1\. Maintenance of stock 2\. Separate housing of species 3\. Separation of pregnant, just delivered, pups, sick animals. 4\. Maintaining room temperature 5\. Feeding practice (pellets, greens etc.,) 6\. Need to use inbred strains in experiments 7\. Disposal of dead animals **ROUTES OF ADMINISTRATION OF DRUGS** OBJECTIVES At the end of the practical group work the student shall be able to: 1\. Measure the required volume of a drug in a syringe using aseptic techniques. 2\. Administer drugs through the subcutaneous, intramuscular and intravenous routes. 3\. Appreciate how the route of administration influences the onset of action of a drug. Task: Measure the following volumes of the solution from the given ampoule/vial. i. 0.1ml (ii) 1.2ml (iii) 4.5ml (iv) 8.0ml COMPONENTS OF TASK \- Choosing the appropriate syringe for the volume \- Choosing the appropriate gauge needle for the desired route \- Expelling air bubbles \- Aseptic technique while opening the packet/handling the syringe \- Withdrawing from a vial/breaking an ampoule \- Short bevel for iv route, long bevel for im Task: 2 (a) Inject 0.04 ml into the given animal subcutaneously. \(b) Inject 1 ml into the provided model (intramuscularly). COMPONENTS OF TASK \- Cleaning the site \- Choosing the site \- Withdrawing to see if in vein \- Pressure on puncture site \- Aseptic technique