Pharmacology PDF
Document Details
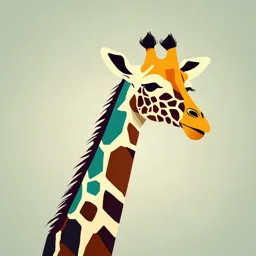
Uploaded by DauntlessGradient
A-B Tech
Tags
Summary
This document discusses the autonomic nervous system and endocrine system's coordination of bodily functions. It describes the nervous system's components including the central and peripheral nervous systems. This document also covers autonomic drugs, and chemical signaling in the ANS involving neurotransmitters.
Full Transcript
1. The autonomic nervous system (ANS) and endocrine system coordinate bodily functions. 2. Autonomic drugs mimic or alter ANS functions. 3. The nervous system consists of the central nervous system (CNS) and peripheral nervous system. 4. The peripheral nervous system is subdivided into the effere...
1. The autonomic nervous system (ANS) and endocrine system coordinate bodily functions. 2. Autonomic drugs mimic or alter ANS functions. 3. The nervous system consists of the central nervous system (CNS) and peripheral nervous system. 4. The peripheral nervous system is subdivided into the efferent and afferent divisions. 5. The efferent division includes the somatic nervous system and the ANS. 6. The ANS regulates vital bodily functions without conscious participation and controls smooth muscle, cardiac muscle, vasculature, and exocrine glands. 7. The ANS has two types of efferent neurons: preganglionic neurons located within the CNS and postganglionic neurons located in ganglia. 8. Afferent neurons play a role in reflex regulation and signaling the CNS. 9. The ANS is divided into the sympathetic and parasympathetic nervous systems, originating from different regions of the spinal cord. 10. The sympathetic system involves preganglionic neurons from the thoracic and lumbar regions, and postganglionic neurons extend to the innervated tissues. 1. Parasympathetic preganglionic fibers originate from cranial nerves III, VII, IX, X, and the sacral region of the spinal cord. 2. Parasympathetic preganglionic fibers have long projections, while postganglionic fibers are short and synapse near or on the target organs. 3. The enteric nervous system is a division of the ANS that controls the gastrointestinal tract independently of the CNS. 4. The sympathetic nervous system adjusts in response to stressful situations and is responsible for increasing heart rate, blood pressure, energy mobilization, and blood flow to skeletal muscles. 5. Sympathetic stimulation dilates pupils and bronchioles, reduces GI motility, and affects bladder and sexual organ function. 6. The "fight or flight" response involves sympathetic activation of effector organs and release of epinephrine and norepinephrine from the adrenal medulla. 7. The parasympathetic division maintains homeostasis and predominates in "rest-and-digest" situations. 8. The parasympathetic system activates specific organ innervation separately rather than discharging as a complete system. 9. The CNS receives sensory input from peripheral structures and integrates it with autonomic functions through reflex arcs. 10. Reflex arcs in the ANS involve sensory (afferent) input and motor (efferent) output to maintain bodily functions and respond to stimuli. 1. Most organs are innervated by both divisions of the ANS, but one system usually predominates in controlling the activity of a given organ. 2. Some effector organs, like the adrenal medulla, kidney, pilomotor muscles, and sweat glands, receive innervation only from the sympathetic system. 3. The efferent somatic nervous system differs from the ANS in that it directly controls skeletal muscles without the mediation of ganglia and is under voluntary control. 4. The sympathetic nervous system has a widespread distribution and innervates practically all effector systems in the body, while the parasympathetic division has a more limited distribution. 5. The parasympathetic division has more one-to-one interactions and limited branching, while the sympathetic division allows for a diffuse discharge. 6. Chemical signaling in the ANS involves neurotransmitters, hormones, and local mediators. 7. Neurotransmitters are released from nerve terminals and bind to specific receptors on target cells. 8. Common neurotransmitters in the ANS include acetylcholine and norepinephrine. 9. Acetylcholine mediates transmission across autonomic ganglia and at the neuromuscular junction in the somatic nervous system. 10. Cholinergic neurons release acetylcholine, while adrenergic neurons release norepinephrine. 1. Postganglionic fibers in the sympathetic nervous system are long and allow extensive branching to innervate multiple organ systems, allowing for a coordinated discharge. 2. Epinephrine (80%) and norepinephrine (20%) are released from the adrenal medulla and act as chemical messengers in effector organs. 3. Signal transduction in effector cells involves the binding of chemical signals to receptors, which activates enzymatic processes and leads to cellular responses. 4. Receptors in the ANS effector cells are classified as adrenergic (binding to epinephrine and norepinephrine) or cholinergic (binding to acetylcholine), with further subdivisions such as nicotinic or muscarinic. 5. Adrenergic receptors and cholinergic muscarinic receptors are G protein-coupled receptors, which mediate the effects of ligands by activating second messenger systems inside the cell, such as the adenylyl cyclase system and the calcium/phosphatidylinositol system. 1. Drugs affecting the autonomic nervous system (ANS) are divided into two groups: cholinergic drugs that act on receptors activated by acetylcholine (ACh), and adrenergic drugs that act on receptors stimulated by norepinephrine or epinephrine. 2. Cholinergic drugs can stimulate or block receptors of the ANS, while adrenergic drugs have similar actions on adrenergic receptors. 3. Cholinergic neurons use ACh as a neurotransmitter in various parts of the autonomic and somatic nervous systems, including the adrenal medulla, autonomic ganglia, postganglionic fibers of the parasympathetic division, and postganglionic sympathetic division of sweat glands. 4. Neurotransmission in cholinergic neurons involves steps such as synthesis of ACh, storage in vesicles, release, binding to receptors, degradation by acetylcholinesterase (AChE), and recycling of choline. 5. ACh binds to postsynaptic receptors on target cells, including muscarinic and nicotinic receptors, leading to biological responses within the cell mediated by second messenger molecules. 6. Muscarinic receptors belong to the class of G-protein-coupled receptors and recognize muscarine (found in certain mushrooms) but have weak affinity for nicotine. 7. Muscarinic receptors are found in autonomic effector organs such as the heart, smooth muscle, brain, and exocrine glands, with specific subtypes (M1, M2, M3) found on different tissues. 8. Muscarinic agonists stimulate muscarinic receptors on these tissues, but high concentrations may also show some activity at nicotinic receptors. 1. Acetylcholine (ACh) activates different receptors, such as M1, M3, and M2, which initiate various signaling pathways in response to its binding. 2. M1 and M3 receptors undergo a conformational change and interact with G-proteins, leading to the activation of phospholipase C and the production of second messengers IP3 and DAG. 3. IP3 causes an increase in intracellular Ca2+, which can stimulate or inhibit enzymes, while DAG activates protein kinase C. 4. M2 receptors in cardiac muscle stimulate a G-protein that inhibits adenylyl cyclase, resulting in a decrease in heart rate and force of contraction. 5. Nicotinic receptors recognize both ACh and nicotine and function as ligand-gated ion channels. 6. Activation of nicotinic receptors allows the entry of sodium ions, leading to depolarization of the effector cell. 7. Direct-acting cholinergic agonists, including choline esters and alkaloids like nicotine and pilocarpine, bind directly to muscarinic or nicotinic receptors to mimic the effects of ACh. 8. Muscarinic agents like pilocarpine and bethanechol preferentially bind to muscarinic receptors and have a longer duration of action compared to ACh. 9. ACh has various effects, including a decrease in heart rate and cardiac output, decrease in blood pressure through vasodilation, increased salivary and gastric secretions, bronchoconstriction, stimulation of bladder detrusor muscle for urination, and constriction of the pupillae sphincter muscle causing miosis. 10. Bethanechol is a muscarinic agonist used to stimulate intestinal motility, treat atonic bladder, neurogenic atony, and megacolon. Adverse effects can include generalized cholinergic stimulation, which may be managed with atropine sulfate. 1. Carbachol has both muscarinic and nicotinic actions and can stimulate and depress the cardiovascular and GI systems. It can also cause miosis and spasm of accommodation when applied to the eye. 2. Carbachol is rarely used due to its high potency, receptor nonselectivity, and relatively long duration of action. It is primarily used intraocularly for eye surgery and to lower intraocular pressure in glaucoma. 3. Pilocarpine is a tertiary amine that exhibits muscarinic activity and is used primarily in ophthalmology. 4. Pilocarpine produces rapid miosis, contraction of the ciliary muscle, and spasm of accommodation when topically applied to the eye. It is also effective in promoting salivation in patients with xerostomia. 5. Pilocarpine is the drug of choice for emergency lowering of intraocular pressure in glaucoma and can open the trabecular meshwork to increase drainage of aqueous humor. 6. Pilocarpine can cause adverse effects such as blurred vision, night blindness, and brow ache. Poisoning with pilocarpine can lead to excessive sweating and salivation. 7. Anticholinesterase agents, such as edrophonium, inhibit the degradation of ACh, leading to an accumulation of ACh in the synaptic space and activation of cholinergic receptors. 8. Edrophonium is a short-acting AChE inhibitor used in the diagnosis of myasthenia gravis. It can increase muscle strength in patients with myasthenia gravis but should be used with caution to avoid a cholinergic crisis. 9. Edrophonium may also be used to assess cholinesterase inhibitor therapy, differentiate cholinergic and myasthenic crises, and reverse the effects of nondepolarizing neuromuscular blockers. 10. The use of edrophonium has become limited due to the availability of other agents. 1. Most organs are innervated by both divisions of the ANS, but one system usually predominates in controlling the activity of a given organ. 2. Some effector organs, like the adrenal medulla, kidney, pilomotor muscles, and sweat glands, receive innervation only from the sympathetic system. 3. The efferent somatic nervous system differs from the ANS in that it directly controls skeletal muscles without the mediation of ganglia and is under voluntary control. 4. The sympathetic nervous system has a widespread distribution and innervates practically all effector systems in the body, while the parasympathetic division has a more limited distribution. 5. The parasympathetic division has more one-to-one interactions and limited branching, while the sympathetic division allows for a diffuse discharge. 6. Chemical signaling in the ANS involves neurotransmitters, hormones, and local mediators. 7. Neurotransmitters are released from nerve terminals and bind to specific receptors on target cells. 8. Common neurotransmitters in the ANS include acetylcholine and norepinephrine. 9. Acetylcholine mediates transmission across autonomic ganglia and at the neuromuscular junction in the somatic nervous system. 10. Cholinergic neurons release acetylcholine, while adrenergic neurons release norepinephrine. 1. Postganglionic fibers in the sympathetic nervous system are long and allow extensive branching to innervate multiple organ systems, allowing for a coordinated discharge. 2. Epinephrine (80%) and norepinephrine (20%) are released from the adrenal medulla and act as chemical messengers in effector organs. 3. Signal transduction in effector cells involves the binding of chemical signals to receptors, which activates enzymatic processes and leads to cellular responses. 4. Receptors in the ANS effector cells are classified as adrenergic (binding to epinephrine and norepinephrine) or cholinergic (binding to acetylcholine), with further subdivisions such as nicotinic or muscarinic. 5. Adrenergic receptors and cholinergic muscarinic receptors are G protein-coupled receptors, which mediate the effects of ligands by activating second messenger systems inside the cell, such as the adenylyl cyclase system and the calcium/phosphatidylinositol system. Cholinergic Antagonists 1. Cholinergic antagonists bind to cholinoceptors and block the effects of acetylcholine. 2. Selective blockers of muscarinic receptors are the most clinically useful cholinergic antagonists. 3. Anticholinergic agents, antimuscarinic agents, and parasympatholytics are terms used to describe these agents. 4. Ganglionic blockers preferentially act on nicotinic receptors in sympathetic and parasympathetic ganglia. 5. Neuromuscular blocking agents interfere with transmission of efferent impulses to skeletal muscles. 6. Antimuscarinic agents like atropine and scopolamine block muscarinic receptors and inhibit muscarinic functions. 7. Anticholinergic drugs have little or no action at skeletal neuromuscular junctions or autonomic ganglia. 8. Atropine is a tertiary amine that competitively binds to muscarinic receptors. 9. Atropine acts both centrally and peripherally and has various effects on different organs. 10. Atropine causes mydriasis, unresponsiveness to light, and cycloplegia in the eyes. 11. It can be used as an antispasmodic in the gastrointestinal tract. 12. Atropine has divergent effects on the cardiovascular system depending on the dose. 13. It blocks muscarinic receptors in the salivary glands, causing dry mouth. 14. Atropine is used in ophthalmic applications for its mydriatic and cycloplegic effects. 15. It is used as an antispasmodic agent in the GI tract. 16. Injectable atropine is used to treat bradycardia. 17. Atropine is sometimes used as an antisecretory agent in the respiratory tract prior to surgery. 18. Atropine is used in the treatment of organophosphate poisoning and certain types of mushroom poisoning. 19. It is readily absorbed, metabolized by the liver, and eliminated primarily in urine. 20. Adverse effects of atropine include dry mouth, blurred vision, tachycardia, urinary retention, and constipation. 1. Mechanism of action of nondepolarizing agents: They competitively block ACh at nicotinic receptors, preventing depolarization and muscle contraction. Cholinesterase inhibitors can reverse their action. 2. Actions of nondepolarizing agents: Muscles are paralyzed in a specific order, starting with small facial muscles and progressing to larger muscles. Recovery occurs in the reverse order. 3. Pharmacokinetics of nondepolarizing agents: They are administered intravenously and poorly absorbed from the gut. They are eliminated through various routes, depending on the specific agent. 4. Adverse effects of nondepolarizing agents: Generally safe with minimal side effects. Specific adverse effects vary among the different agents. 5. Drug interactions with nondepolarizing agents: Cholinesterase inhibitors can reverse their action but may cause depolarizing block at high doses. Halogenated hydrocarbon anesthetics enhance neuromuscular blockade. Aminoglycoside antibiotics and calcium channel blockers can increase neuromuscular blockade. 6. Mechanism of action of depolarizing agent (succinylcholine): It attaches to nicotinic receptors and causes sustained depolarization of the muscle cell. 7. Actions of succinylcholine: It produces muscle fasciculations followed by flaccid paralysis. Respiratory muscles are paralyzed last. 8. Therapeutic uses of succinylcholine: It is used for rapid endotracheal intubation and during electroconvulsive shock treatment. 9. Pharmacokinetics of succinylcholine: It is injected intravenously and rapidly metabolized by plasma cholinesterase. 10. Adverse effects of succinylcholine: It can induce malignant hyperthermia in susceptible patients. It can cause prolonged apnea in patients deficient in plasma cholinesterase or with atypical enzyme forms. It increases potassium release, which can be dangerous in certain patients. 11. Nondepolarizing agents have a competitive mechanism of action, while depolarizing agents cause sustained depolarization. 12. Muscle paralysis by nondepolarizing agents occurs in a specific order, starting with facial muscles and progressing to larger muscles. 13. Nondepolarizing agents are eliminated through various routes, depending on the specific drug. 14. Adverse effects of nondepolarizing agents vary among the different drugs. 15. Cholinesterase inhibitors can reverse the action of nondepolarizing agents but may cause depolarizing block at high doses. 16. Halogenated hydrocarbon anesthetics enhance neuromuscular blockade caused by nondepolarizing agents. 17. Aminoglycoside antibiotics and calcium channel blockers can increase neuromuscular blockade. 18. Succinylcholine causes sustained depolarization of muscle cells and produces muscle fasciculations followed by flaccid paralysis. 19. Succinylcholine is used for rapid endotracheal intubation and during electroconvulsive shock treatment. 20. Succinylcholine is rapidly metabolized by plasma cholinesterase and can cause adverse effects such as malignant hyperthermia, prolonged apnea, and hyperkalemia in certain patients. Adrenergic Agonists 1. Adrenergic drugs affect adrenergic receptors stimulated by norepinephrine or epinephrine. 2. Adrenergic receptors are activated by sympathomimetics and blocked by sympatholytics. 3. Sympathomimetics can directly activate adrenergic receptors or act indirectly by enhancing release or blocking reuptake of norepinephrine. 4. Adrenergic neurons release norepinephrine as the primary neurotransmitter. 5. Adrenergic drugs act on adrenergic receptors located presynaptically or postsynaptically. 6. Neurotransmission in adrenergic neurons involves synthesis, storage, release, receptor binding, and removal of norepinephrine. 7. Tyrosine is hydroxylated to form DOPA, which is then decarboxylated to form dopamine. 8. Dopamine is hydroxylated to form norepinephrine and stored in vesicles. 9. An action potential triggers calcium influx, vesicle fusion, and release of norepinephrine. 10. Norepinephrine binds to postsynaptic receptors on the effector organ or presynaptic receptors on the nerve ending. 11. Binding of norepinephrine triggers intracellular signaling through second messengers. 12. Norepinephrine can be removed by diffusion, metabolism by COMT, or reuptake into the neuron. 13. Reuptake involves a sodium-chloride-dependent norepinephrine transporter. 14. Recaptured norepinephrine can be sequestered, oxidized by MAO, or released again. 15. Adrenergic receptors can be classified into α and β receptors, with subtypes. 16. α-Adrenoceptors respond to epinephrine and norepinephrine, with α1 and α2 subtypes. 17. α1 receptors are present on postsynaptic membranes and mediate smooth muscle constriction through intracellular signaling. 1. α2 receptors are located on sympathetic presynaptic nerve endings and control the release of norepinephrine. 2. Stimulation of α2 receptors causes feedback inhibition and inhibits further release of norepinephrine. 3. α2 receptors are also found on presynaptic parasympathetic neurons and can inhibit acetylcholine release. 4. α2 receptors act as inhibitory autoreceptors on adrenergic neurons and inhibitory heteroreceptors on parasympathetic neurons. 5. α1 and α2 receptors are further subdivided into multiple subtypes, such as α1A, α1B, α1C, α1D, α2A, α2B, and α2C. 6. β receptors have a stronger response to isoproterenol compared to epinephrine and norepinephrine. 7. β receptors can be divided into three major subgroups: β1, β2, and β3. 8. Tissues with a predominance of β2 receptors, like the vasculature of skeletal muscle, are highly responsive to the effects of circulating epinephrine. 9. Adrenergically innervated organs and tissues have a predominant type of receptor, such as the heart containing predominantly β1 receptors. 10. Prolonged exposure to catecholamines can lead to desensitization of adrenergic receptors. 1. Noncatecholamines have longer half-lives due to the absence of catechol hydroxyl groups. 2. Phenylephrine, ephedrine, and amphetamine are examples of noncatecholamines. 3. Noncatecholamines have increased lipid solubility, allowing greater access to the central nervous system. 4. The nature of the substituent on the amine nitrogen determines the β selectivity of adrenergic agonists. 5. Epinephrine is more potent at β receptors than norepinephrine due to a substituent on the amine nitrogen. 6. Isoproterenol is a strong β agonist with little α activity. 7. Direct-acting agonists act directly on α or β receptors, mimicking the effects of sympathetic stimulation. 8. Indirect-acting agonists block reuptake or cause the release of norepinephrine from adrenergic neurons. 9. Mixed-action agonists stimulate adrenoceptors directly and enhance norepinephrine release. 10. Epinephrine interacts with both α and β receptors. 11. Epinephrine strengthens myocardial contractility and increases heart rate. 12. Epinephrine constricts arterioles in the skin and viscera but dilates vessels to the liver and skeletal muscle. 13. Epinephrine causes bronchodilation and inhibits the release of allergy mediators. 14. Epinephrine induces hyperglycemia by increasing glycogenolysis and glucagon release. 15. Epinephrine initiates lipolysis through β receptor activation in adipose tissue. 16. Epinephrine is used in the emergency treatment of bronchospasm and anaphylactic shock. 17. Epinephrine can be used to restore cardiac rhythm in cardiac arrest. 18. Epinephrine is added to local anesthetics to prolong their duration and promote local hemostasis. 19. Epinephrine is used in intraocular surgery to induce and maintain mydriasis. 20. Epinephrine has a rapid onset but brief duration of action, and it is rapidly metabolized and excreted in urine. 1. Epinephrine can cause adverse effects on the central nervous system (CNS) such as anxiety, fear, tension, headache, and tremor. 2. Epinephrine can trigger cardiac arrhythmias, especially when combined with digoxin. 3. Pulmonary edema can be induced by epinephrine due to increased afterload caused by its vasoconstrictive properties. 4. Patients with hyperthyroidism may have an enhanced response to epinephrine due to increased adrenergic receptors in the vasculature. 5. Inhalation anesthetics sensitize the heart to the effects of epinephrine, potentially leading to tachycardia. 6. Epinephrine increases the release of glucose stores and may require increased insulin dosages in diabetic patients. 7. Nonselective β-blockers can prevent the vasodilatory effects of epinephrine on β2 receptors, resulting in increased peripheral resistance and blood pressure. 8. Norepinephrine primarily stimulates α-adrenergic receptors, causing vasoconstriction and increasing peripheral resistance. 9. The baroreceptor reflex is activated by norepinephrine, leading to increased vagal activity and reflex bradycardia. 10. Norepinephrine is used to treat shock, particularly septic shock, by increasing vascular resistance and blood pressure. 11. Norepinephrine is administered intravenously, has a rapid onset of action, and a duration of 1 to 2 minutes. 12. Adverse effects of norepinephrine are similar to those of epinephrine, including vasoconstriction-related skin blanching and necrosis if extravasation occurs. 13. Phentolamine is an α receptor antagonist used to counter impaired circulation caused by norepinephrine. 14. Isoproterenol is a nonselective β1- and β2-adrenergic receptor agonist that stimulates the heart, increasing heart rate, contractility, and cardiac output. 15. Isoproterenol also dilates arterioles in skeletal muscle, resulting in decreased peripheral resistance. 16. Isoproterenol is a potent bronchodilator with similar adverse effects to epinephrine related to β receptor activation. 17. Dopamine activates α- and β-adrenergic receptors, causing vasoconstriction at higher doses and cardiac stimulation through β1 receptors at lower doses. 18. Dopamine is used to treat shock, hypotension, severe heart failure, and bradycardia unresponsive to other treatments. 1. Dobutamine is a synthetic, direct-acting catecholamine that primarily acts as a β1 receptor agonist. 2. Dobutamine increases heart rate and cardiac output without significant vascular effects. 3. It is used to increase cardiac output in acute heart failure and for inotropic support after cardiac surgery. 4. Dobutamine should be used with caution in atrial fibrillation as it increases atrioventricular conduction. 5. Adverse effects of dobutamine are similar to those of epinephrine. 6. Tolerance may develop with prolonged use of dobutamine. 7. Oxymetazoline is a direct-acting synthetic adrenergic agonist that stimulates α1 and α2 adrenergic receptors. 8. Oxymetazoline is used in nasal sprays and ophthalmic drops for decongestion and relief of redness in the eyes. 9. It produces vasoconstriction and decreases congestion by stimulating α receptors on blood vessels. 10. Oxymetazoline may cause nervousness, headaches, trouble sleeping, local irritation, and sneezing. 11. Phenylephrine is a direct-acting synthetic adrenergic drug that primarily binds to α1 receptors. 12. It is a vasoconstrictor used to raise blood pressure and induce reflex bradycardia in the treatment of certain conditions. 13. Large doses of phenylephrine can cause hypertensive headache and cardiac irregularities. 14. Midodrine is a prodrug that is metabolized to desglymidodrine, a selective α1 agonist. 15. Midodrine is used to treat orthostatic hypotension and should be administered three times daily. 1. Mirabegron is a β3 agonist that relaxes the detrusor smooth muscle and increases bladder capacity. 2. It is used for patients with overactive bladder. 3. Mirabegron may increase blood pressure and should not be used in patients with uncontrolled hypertension. 4. Mirabegron inhibits the CYP2D6 isozyme and may enhance the effects of other medications metabolized by this pathway. 5. Amphetamine is an indirect-acting adrenergic drug that increases blood pressure by α1 agonist action on the vasculature and β1 stimulatory effects on the heart. 6. Tyramine is found in fermented foods and can displace stored norepinephrine, leading to adrenoceptor activation. 7. Cocaine blocks the norepinephrine transporter, leading to enhanced sympathetic activity and potentiation of the actions of epinephrine and norepinephrine. 8. Ephedrine and pseudoephedrine are mixed-action adrenergic agents that enhance the release of stored norepinephrine and directly stimulate α and β receptors. 9. Ephedrine raises blood pressure by vasoconstriction and cardiac stimulation and is used to treat anesthesia-induced hypotension. 10. Ephedrine produces bronchodilation and mild CNS stimulation. 11. Pseudoephedrine is primarily used to treat nasal and sinus congestion and has restrictions due to its potential for illegal use in producing methamphetamine. Adrenergic Antagonists 1. Adrenergic antagonists, also known as adrenergic blockers or sympatholytics, bind to adrenoceptors and prevent their activation by agonists. 2. They are classified based on their affinities for α or β receptors in the sympathetic nervous system. 3. Adrenergic antagonists play a significant role in clinical medicine, primarily in the treatment of cardiovascular diseases. 4. α-Adrenergic blocking agents can block either α1 or α2 receptors, affecting blood pressure regulation. 5. Blocking α1 receptors reduces peripheral vascular resistance, leading to decreased blood pressure and reflex tachycardia. 6. Phenoxybenzamine is a nonselective, noncompetitive blocker of α1 and α2 receptors, used in the treatment of pheochromocytoma, Raynaud disease, and frostbite. 7. Phenoxybenzamine prevents vasoconstriction and increases heart rate and cardiac output. 8. Phentolamine competitively blocks α1 and α2 receptors and is used for the diagnosis and short-term management of pheochromocytoma. 9. Prazosin, terazosin, and doxazosin are selective competitive blockers of α1 receptors used for the treatment of hypertension. 10. These drugs lower blood pressure by relaxing arterial and venous smooth muscle, with minimal effects on cardiac output. 11. Tamsulosin, alfuzosin, and silodosin are other selective α1 antagonists used to treat benign prostatic hyperplasia. 12. The first dose of selective α1 antagonists may cause an exaggerated orthostatic hypotensive response, termed the "first-dose" effect. 13. Adjusting the first dose and administering the drug at bedtime can minimize the first-dose effect. 14. α1 antagonists may also improve lipid profiles and glucose metabolism in hypertensive patients. 15. However, they are not used as monotherapy for hypertension due to inferior cardiovascular outcomes. 16. Phenoxybenzamine can cause adverse effects such as postural hypotension, nasal stuffiness, nausea, vomiting, and inhibition of ejaculation. 17. Caution should be exercised when using phenoxybenzamine in patients with cerebrovascular or cardiovascular disease. 1. Adverse effects of α1-blockers like prazosin and doxazosin include dizziness, lack of energy, nasal congestion, headache, drowsiness, and orthostatic hypotension. 2. α1-blockers can have an additive antihypertensive effect when used with vasodilators like nitrates or PDE- 5 inhibitors. 3. "Floppy iris syndrome" can occur as an adverse effect of α1-blockers during intraoperative eye surgery. 4. Yohimbine is a selective competitive α2-blocker used as a sexual stimulant and for the treatment of erectile dysfunction, although its efficacy is not well-established. 5. β-blockers are competitive antagonists that act on β1 and β2 receptors, with nonselective β-blockers blocking both receptors. 6. Propranolol is a nonselective β-blocker that decreases cardiac output, peripheral vascular resistance, and bronchodilation. 7. β-blockers are used for various conditions, including hypertension, angina, myocardial infarction, heart failure, hyperthyroidism, and glaucoma. They can also be used prophylactically for migraine. 8. Propranolol has specific therapeutic uses in hypertension, angina pectoris, myocardial infarction, migraine, and hyperthyroidism. 1. Propranolol can cause significant bronchoconstriction due to blocking β2 receptors, making it contraindicated in patients with COPD or asthma. 2. Abruptly stopping β-blockers can lead to cardiac arrhythmias, so they should be tapered off gradually over a few weeks. 3. Propranolol may cause impaired sexual activity in male patients, although the exact reasons are unclear. 4. β-blockers can decrease glycogenolysis, glucagon secretion, and counterregulatory effects of catecholamines during hypoglycemia. 5. Propranolol can have various CNS-mediated effects, including depression, dizziness, lethargy, visual disturbances, and emotional lability. 6. Drugs like cimetidine, fluoxetine, paroxetine, and ritonavir can potentiate the antihypertensive effects of propranolol, while others like barbiturates, phenytoin, and rifampin can decrease its effects. 7. Timolol, nadolol, levobunolol, metipranolol, and betaxolol are nonselective β antagonists used in the treatment of glaucoma. 8. β-blockers decrease the secretion of aqueous humor in the eye and are effective in diminishing intraocular pressure. 9. Pilocarpine is the drug of choice for emergency lowering of intraocular pressure in an acute attack of glaucoma. 10. Acebutolol, atenolol, betaxolol, bisoprolol, esmolol, metoprolol, and nebivolol are selective β1 antagonists that minimize unwanted bronchoconstriction seen with nonselective agents in asthma patients. 11. These cardioselective β-blockers lower blood pressure in hypertension and increase exercise tolerance in angina. 12. Esmolol has a short half-life and is used intravenously to control blood pressure or heart rhythm in critically ill patients. 13. Nebivolol releases nitric oxide from endothelial cells, causing vasodilation in addition to its β-blockade. 14. The cardioselective β-blockers have fewer effects on pulmonary function, peripheral resistance, and carbohydrate metabolism compared to nonselective agents. 15. Acebutolol and pindolol are partial agonists with intrinsic sympathomimetic activity (ISA) that have a diminished effect on reducing heart rate and cardiac output compared to other β-blockers. 1. β-Blockers with intrinsic sympathomimetic activity (ISA) have fewer metabolic effects compared to other β-blockers and do not decrease plasma HDL levels. 2. Labetalol and carvedilol are nonselective β-blockers with α1-blocking actions, causing peripheral vasodilation and reducing blood pressure. Carvedilol also has additional benefits in heart failure. 3. Labetalol is used in the treatment of pregnancy-induced hypertension and as an alternative to methyldopa. Carvedilol, metoprolol, and bisoprolol are beneficial in stable chronic heart failure. 4. Reserpine, a rarely used agent, depletes biogenic amines by blocking their transport into storage vesicles, impairing sympathetic function. It has been used for hypertension and agitated psychotic states but has largely been replaced by newer agents. Drugs Affecting the Central Nervous System Drugs for Neurodegenerative Diseases 1. Drugs affecting the central nervous system (CNS) alter neurotransmission. 2. They can act presynaptically by influencing neurotransmitter production, storage, release, or termination. 3. Some drugs activate or block postsynaptic receptors. 4. Understanding CNS neurotransmitters is important for treating neurodegenerative disorders. 5. CNS neurons function similarly to the autonomic nervous system (ANS). 6. Neuronal transmission involves the release of neurotransmitters that bind to receptors on postsynaptic neurons. 7. The CNS has more complex circuitry and a greater number of synapses than the ANS. 8. The CNS uses multiple neurotransmitters, while the ANS primarily uses acetylcholine and norepinephrine. 9. Receptors in CNS synapses are coupled to ion channels. 10. Neurotransmitter binding results in the opening of ion channels and changes in ionic composition. 11. Excitatory pathways cause depolarization of the postsynaptic membrane. 12. Inhibitory pathways cause hyperpolarization of the postsynaptic membrane. 13. Most CNS neurons receive both excitatory and inhibitory input. 14. Neurodegenerative diseases include Parkinson's disease, Alzheimer's disease, multiple sclerosis (MS), and amyotrophic lateral sclerosis (ALS). 15. Parkinson's disease involves the destruction of dopaminergic neurons in the substantia nigra, leading to motor control issues. 1. Drugs that block dopamine receptors in the brain can produce parkinsonian symptoms. 2. The goal of Parkinson's disease treatment is to restore dopamine levels and counteract the effects of cholinergic neurons. 3. Available drugs for Parkinson's aim to maintain constant CNS dopamine levels but do not reverse neuronal degeneration. 4. Levodopa is a precursor of dopamine and restores dopaminergic neurotransmission in the brain. 5. Levodopa must be administered with carbidopa to prevent its peripheral metabolism and enhance its availability in the CNS. 6. Levodopa-carbidopa combination therapy is effective in reducing rigidity, tremors, and other symptoms of Parkinson's. 7. Levodopa is absorbed rapidly from the small intestine and has a short half-life, causing fluctuations in plasma concentration. 8. Fluctuations in motor response and the "on-off" phenomenon may occur due to levodopa's short half-life. 9. Levodopa should be taken on an empty stomach, and meals, particularly high-protein meals, can interfere with its CNS transport. 10. Levodopa can cause various adverse effects, including peripheral effects (nausea, vomiting, tachycardia) and central effects (hallucinations, dyskinesias, mood changes). 1. Pyridoxine (B6) reduces the effectiveness of levodopa when taken together. 2. Levodopa should not be taken with nonselective monoamine oxidase inhibitors (MAOIs) like phenelzine due to the risk of hypertensive crisis. 3. Levodopa can worsen symptoms in psychotic patients by increasing central catecholamines. 4. Cardiac patients should be monitored for arrhythmias when taking levodopa. 5. Antipsychotic drugs that block dopamine receptors are generally contraindicated in Parkinson's disease. 6. Low doses of atypical antipsychotics like quetiapine or clozapine may be used to treat levodopa-induced psychotic symptoms. 7. Selegiline selectively inhibits MAO-B, increasing dopamine levels in the brain when taken with levodopa. 8. Rasagiline is a more potent and irreversible inhibitor of MAO-B compared to selegiline. 9. Entacapone and tolcapone inhibit catechol-O-methyltransferase (COMT) to increase central uptake of levodopa. 10. Dopamine receptor agonists like bromocriptine, ropinirole, pramipexole, rotigotine, and apomorphine have a longer duration of action than levodopa and can be effective in patients with motor fluctuations. 1. Apomorphine, pramipexole, ropinirole, and rotigotine are nonergot dopamine agonists used to treat Parkinson's disease. 2. Ropinirole is also indicated for restless legs syndrome. 3. Pramipexole and ropinirole are available in oral form, while apomorphine and rotigotine are administered through injectable and transdermal delivery systems, respectively. 4. These dopamine agonists alleviate motor deficits in both levodopa-naive patients and those with advanced Parkinson's disease who are already on levodopa treatment. 5. Dopamine agonists can delay the need for levodopa in early Parkinson's disease and reduce the levodopa dosage in advanced stages. 6. Compared to levodopa, dopamine agonists have a lower frequency of dyskinesias but can cause adverse effects such as nausea, hallucinations, insomnia, dizziness, constipation, and orthostatic hypotension. 7. Pramipexole is mainly excreted unchanged in the urine and requires dosage adjustments in renal dysfunction. 8. Ropinirole metabolism may be inhibited by fluoroquinolone antibiotics and other inhibitors of the cytochrome P450 1A2 isoenzyme. 9. Amantadine is an antiviral drug with antiparkinsonian effects, which include increasing dopamine release, blocking cholinergic receptors, and inhibiting NMDA glutamate receptors. 10. Antimuscarinic agents like benztropine and trihexyphenidyl have a limited role in antiparkinsonism therapy and can induce mood changes, confusion, dry mouth, constipation, and visual problems. 1. Glutamate receptor stimulation is important for memory formation but overstimulation can lead to neuronal damage and apoptosis. 2. Memantine is an NMDA receptor antagonist used to treat moderate to severe Alzheimer's disease by blocking excessive calcium influx into neurons. 3. Multiple sclerosis (MS) is an autoimmune inflammatory demyelinating disease of the CNS. 4. Corticosteroids and chemotherapeutic agents have historically been used to treat acute exacerbations of MS. 5. Disease-modifying therapies for MS aim to decrease relapse rates and modify the immune response. 6. Interferon b1a and b1b modulate the immune response and have potential side effects such as depression and injection site reactions. 7. Glatiramer resembles myelin protein and may act as a decoy to T-cell attack in MS. 8. Fingolimod alters lymphocyte migration and carries a risk of bradycardia, infection, and macular edema. 9. Teriflunomide inhibits pyrimidine synthesis and may elevate liver enzymes. 10. Dimethyl fumarate reduces disease progression by altering the cellular response to oxidative stress. 11. Monoclonal antibodies like alemtuzumab, daclizumab, natalizumab, and ocrelizumab are used in MS treatment but have significant toxicities. 12. Symptomatic treatment for MS includes various drugs to manage spasticity, constipation, bladder dysfunction, and depression. 13. Dalfampridine, a potassium channel blocker, improves walking speeds in MS patients. 14. Amyotrophic lateral sclerosis (ALS) is characterized by progressive motor neuron degeneration. 15. Riluzole, an NMDA receptor antagonist, and edaravone, an antioxidant, are used to manage ALS. Anxiolytic and Hypnotic Drugs 1. Anxiety is a common mental disorder characterized by tension and uneasiness. 2. Severe anxiety may be treated with antianxiety drugs and/or psychotherapy. 3. Benzodiazepines are widely used anxiolytic drugs. 4. Benzodiazepines act on γ-aminobutyric acid (GABA) receptors in the central nervous system. 5. They reduce anxiety, induce sedation and hypnosis, and cause anterograde amnesia. 6. Benzodiazepines also have anticonvulsant and muscle relaxant properties. 7. Different benzodiazepines have small differences in their effects. 8. They are effective for treating anxiety associated with various disorders. 9. Benzodiazepines should be reserved for severe anxiety and used for short periods of time. 10. Longer-acting agents are preferred for prolonged treatment. 11. The antianxiety effects of benzodiazepines are less subject to tolerance than sedative and hypnotic effects. 12. Alprazolam is effective for panic disorders but may cause withdrawal reactions in some patients. 1. Benzodiazepine hypnotics are used to treat sleep disorders by decreasing sleep onset latency and increasing non-REM sleep stage II. 2. Triazolam is effective for individuals with difficulty falling asleep but has a higher risk of withdrawal and rebound insomnia. 3. Temazepam is useful for patients who experience frequent awakenings and have trouble staying asleep. 4. Flurazepam is rarely used due to its extended half-life, which can result in excessive daytime sedation and drug accumulation. 5. Clonazepam, lorazepam, and diazepam are used in the treatment of seizures and alcohol withdrawal. 6. Diazepam is effective in treating skeletal muscle spasms and spasticity from degenerative disorders. 7. Benzodiazepines are rapidly and completely absorbed after oral administration and distribute throughout the body, including the central nervous system. 8. The half-lives of benzodiazepines determine their duration of action and can be categorized as short-, intermediate-, or long-acting. 9. Benzodiazepines can lead to psychological and physical dependence if used in high doses for prolonged periods. 10. Adverse effects of benzodiazepines include drowsiness, confusion, ataxia, cognitive impairment, and potential interactions with other central nervous system depressants. Here's a 10-point summary based directly on the information provided in the text: 1. Buspirone is useful for the chronic treatment of generalized anxiety disorder (GAD) and has comparable efficacy to benzodiazepines. 2. Buspirone has a slow onset of action and is not effective for short-term or acute anxiety treatment. 3. The actions of buspirone are mediated by serotonin (5-HT1A) receptors, with some affinity for D2 dopamine receptors and 5-HT2A serotonin receptors. 4. Buspirone's mode of action differs from that of benzodiazepines, and it lacks anticonvulsant and muscle- relaxant properties. 5. The frequency of adverse effects with buspirone is low, with common effects being headache, dizziness, nervousness, nausea, and lightheadedness. 6. Sedation, psychomotor impairment, and cognitive dysfunction are minimal with buspirone, and dependence is unlikely. 7. Buspirone does not potentiate the central nervous system (CNS) depression caused by alcohol. 8. Barbiturates, which were once commonly used for sedation and sleep induction, have been largely replaced by benzodiazepines due to tolerance, physical dependence, overdose lethality, and severe withdrawal symptoms. 9. Barbiturates enhance GABAergic transmission by interacting with GABAA receptors, and their binding site on the receptor is distinct from that of benzodiazepines. 10. Barbiturates can depress the CNS, cause respiratory depression, and have therapeutic uses as anesthesia inducers, anticonvulsants, and sedative/hypnotics, although their use for insomnia is generally discouraged due to adverse effects and tolerance. 1. Barbiturates are well absorbed after oral administration and distribute throughout the body, including the brain, splanchnic areas, skeletal muscle, and adipose tissue. 2. Barbiturates readily cross the placenta and can have depressant effects on the fetus. 3. Barbiturates are metabolized in the liver, and inactive metabolites are excreted in urine. 4. Adverse effects of barbiturates include drowsiness, impaired concentration, mental and psychomotor impairment, nausea, and dizziness. 5. Barbiturates induce cytochrome P450 microsomal enzymes in the liver, which can affect the metabolism of other drugs. 6. Chronic barbiturate use can diminish the action of drugs metabolized by the CYP450 system. 7. Barbiturates are contraindicated in patients with acute intermittent porphyria. 8. Abrupt withdrawal from barbiturates can cause tremors, anxiety, weakness, restlessness, nausea and vomiting, seizures, delirium, and cardiac arrest. 9. Barbiturate withdrawal is more severe than opioid withdrawal and can result in death. 10. Zolpidem, zaleplon, and eszopiclone are nonbenzodiazepine hypnotics that have fewer residual effects on psychomotor and cognitive function compared to benzodiazepines. 1. Sedating antihistamines like diphenhydramine, hydroxyzine, and doxylamine can be used to treat mild situational insomnia but have undesirable adverse effects. 2. Sedating antidepressants with strong antihistamine properties, such as doxepin, trazodone, and mirtazapine, are sometimes used off-label for the treatment of insomnia. 3. Suvorexant is an orexin receptor antagonist that suppresses the wake drive and is used as a hypnotic. It can cause adverse effects similar to narcolepsy and cataplexy, including daytime somnolence and increased suicidal ideation. 4. Suvorexant is metabolized by CYP450 3A4, which means it may have drug interactions with CYP3A4 inducers or inhibitors. Antidepressants 1. Symptoms of depression include sadness, hopelessness, loss of pleasure in activities, changes in sleep patterns, appetite changes, loss of energy, and suicidal thoughts. 2. Mania is characterized by enthusiasm, anger, rapid thought and speech patterns, extreme self-confidence, and impaired judgment. 3. Most antidepressant drugs potentiate the actions of norepinephrine and/or serotonin in the brain. 4. The biogenic amine theory suggests that depression is due to a deficiency of monoamines, while mania is caused by an overproduction of these neurotransmitters. 5. The biogenic amine theory fails to explain the time course for therapeutic response. 6. Selective serotonin reuptake inhibitors (SSRIs) specifically inhibit serotonin reuptake. 7. SSRIs have little blocking activity at muscarinic, α-adrenergic, and histaminic H1 receptors. 8. SSRIs are the drugs of choice in treating depression due to their safety profile and fewer adverse effects. 9. Common SSRIs include fluoxetine, citalopram, escitalopram, fluvoxamine, paroxetine, and sertraline. 10. SSRIs block the reuptake of serotonin, leading to increased concentrations of the neurotransmitter. 11. SSRIs typically take at least 2 weeks to produce significant improvement in mood. 12. SSRIs are used to treat depression and other psychiatric disorders such as obsessive–compulsive disorder, panic disorder, and social anxiety disorder. 13. SSRIs are well absorbed after oral administration and have plasma half-lives ranging between 16 and 36 hours. 14. Fluoxetine has a much longer half-life (50 hours) and its active metabolite has a long half-life of about 10 days. 15. SSRIs may cause adverse effects such as headache, sweating, gastrointestinal effects, sexual dysfunction, changes in weight, and sleep disturbances. 16. Paroxetine and fluvoxamine are more sedating, while fluoxetine and sertraline are more activating. 17. Sexual dysfunction is common with SSRIs. 18. SSRIs should be used cautiously in children and teenagers due to the potential for suicidal ideation. 19. Overdose with SSRIs may cause seizures and serotonin syndrome. 20. Abrupt withdrawal of SSRIs may result in a discontinuation syndrome with symptoms such as headache, malaise, agitation, and changes in sleep pattern. 1. Venlafaxine inhibits serotonin and norepinephrine reuptake, with minimal inhibition of CYP450 enzymes. 2. Desvenlafaxine is the active metabolite of venlafaxine with a similar profile of clinical activity and side effects. 3. Duloxetine inhibits serotonin and norepinephrine reuptake and should be used with caution in patients with liver dysfunction. 4. Levomilnacipran is similar to milnacipran and is primarily metabolized by CYP3A4. 5. Atypical antidepressants include bupropion, mirtazapine, nefazodone, trazodone, vilazodone, and vortioxetine. 6. Bupropion is a weak dopamine and norepinephrine reuptake inhibitor and is used for depression and smoking cessation. 7. Mirtazapine enhances serotonin and norepinephrine transmission and causes sedation and weight gain. 8. Nefazodone and trazodone inhibit serotonin reuptake and have sedating effects. 9. Vilazodone is a serotonin reuptake inhibitor and a partial agonist at 5-HT1a receptors. 10. Vortioxetine inhibits serotonin reuptake, agonizes 5-HT1a receptors, and antagonizes 5-HT3 and 5-HT7 receptors. 11. Tricyclic antidepressants (TCAs) inhibit norepinephrine and serotonin reuptake and include imipramine, amitriptyline, clomipramine, doxepin, trimipramine, desipramine, nortriptyline, protriptyline, maprotiline, and amoxapine. 12. TCAs have different adverse effects compared to newer antidepressants. 13. TCAs are tertiary amines (imipramine, amitriptyline, clomipramine, doxepin, trimipramine) and secondary amines (desipramine, nortriptyline, protriptyline). 14. Maprotiline and amoxapine are related tetracyclic antidepressants that are also included in the class of TCAs. 15. Bupropion is metabolized by the CYP2B6 pathway and has a low risk of drug interactions. 16. Trazodone is commonly used off-label for insomnia and has a risk of priapism. 17. Nefazodone has a risk of hepatotoxicity. 18. Vilazodone has a similar adverse effect profile to SSRIs and may cause discontinuation syndrome if abruptly stopped. 1. TCAs and amoxapine inhibit the reuptake of norepinephrine and serotonin. 2. TCAs block various receptors, including serotonergic, α-adrenergic, histaminic, and muscarinic receptors. 3. TCAs improve mood in 50-70% of individuals with major depression. 4. TCAs are used to treat moderate to severe depression, panic disorder, bed-wetting in children, migraines, chronic pain, and insomnia. 5. TCAs have variable bioavailability due to first-pass metabolism in the liver. 6. Adverse effects of TCAs include blurred vision, dry mouth, urinary retention, constipation, cardiac conduction effects, orthostatic hypotension, sedation, weight gain, and sexual dysfunction. 7. TCAs should be used with caution in patients with bipolar disorder and suicidal patients. 8. TCAs have a narrow therapeutic index and can be lethal in overdose. 9. TCAs can interact with other drugs, exacerbate certain medical conditions, and require dietary restrictions. 10. MAOIs irreversibly or reversibly inhibit monoamine oxidase, leading to increased stores of norepinephrine, serotonin, and dopamine. 11. MAOIs have delayed antidepressant action and may cause agitation or insomnia. 12. MAOIs are used when other antidepressants are ineffective or not tolerated. 13. MAOIs have a high risk of drug-drug and drug-food interactions. 14. Selegiline administered as a transdermal patch may have fewer interactions. 15. MAOIs are considered last-line agents due to their interactions and dietary restrictions. 16. Selegiline is also used for the treatment of Parkinson's disease. 1. MAOIs are well absorbed after oral administration and undergo hepatic metabolism and rapid renal excretion. 2. MAOIs have severe and unpredictable side effects due to drug-food and drug-drug interactions, including a hypertensive crisis caused by consuming tyramine-containing foods. 3. Adverse effects of MAOIs include drowsiness, orthostatic hypotension, blurred vision, dry mouth, and constipation. 4. SSRIs should not be coadministered with MAOIs due to the risk of serotonin syndrome, and a washout period of at least 2 weeks is required before switching between these medications. 5. Serotonin-dopamine antagonists (SDAs) or atypical antipsychotics can be used as adjunctive treatments to antidepressants in partial responders. 6. Aripiprazole, brexpiprazole, quetiapine, and the combination of fluoxetine and olanzapine are approved as adjuncts in major depressive disorder. 7. Lithium salts are used for managing bipolar disorder and have a therapeutic efficacy of 60-80% in treating mania and hypomania. 8. Lithium has a low therapeutic index and can be toxic, with adverse effects including headache, dry mouth, tremors, dizziness, and sedation. 9. Antiepileptic drugs such as carbamazepine, valproic acid, and lamotrigine are approved as mood stabilizers for bipolar disorder. 10. Atypical antipsychotics, including risperidone, olanzapine, ziprasidone, aripiprazole, asenapine, cariprazine, and quetiapine, are used for the management of mania in bipolar disorder, and some medications are also approved for bipolar depression. Antipsychotic Drugs 1. Antipsychotic drugs are used to treat schizophrenia and other psychotic and manic states. 2. The use of antipsychotic medications involves a trade-off between alleviating symptoms and the risk of adverse effects. 3. Antipsychotic drugs do not cure chronic thought disorders but can reduce the intensity of hallucinations and delusions. 4. Schizophrenia is a chronic psychosis characterized by delusions, hallucinations, and disturbances in thought. 5. Antipsychotic drugs are divided into first- and second-generation agents. 6. First-generation antipsychotics block dopamine D2 receptors and are associated with extrapyramidal symptoms (EPS). 7. Second-generation antipsychotics have a lower incidence of EPS but a higher risk of metabolic adverse effects. 8. Second-generation agents are generally used as first-line therapy for schizophrenia. 9. Clozapine is effective for refractory patients but has serious adverse effects. 10. Antipsychotic drugs block dopamine and serotonin receptors in the brain. 11. Antipsychotic effects alleviate hallucinations and delusions. 12. Extrapyramidal effects include dystonias, Parkinson-like symptoms, akathisia, and tardive dyskinesia. 13. Antipsychotics have antiemetic effects and can cause anticholinergic effects. 14. Antipsychotics can cause orthostatic hypotension, sedation, increased prolactin release, and sexual dysfunction. 15. Weight gain is a common adverse effect of antipsychotics, particularly second-generation agents. 16. Individual patient response and comorbid conditions guide drug selection. 17. Differences in therapeutic efficacy among second-generation drugs have not been established. 18. Clozapine has a minimal risk of EPS but serious adverse effects. 19. Second-generation antipsychotics block both dopamine and serotonin receptors. 20. The clinical effects of antipsychotic drugs result from their blockade of dopamine and serotonin receptors, while adverse effects may stem from actions at other receptors. 1. Antipsychotics are the primary pharmacological treatment for schizophrenia, particularly the first- generation agents for positive symptoms and atypical agents for negative symptoms. 2. Antipsychotics can be used to prevent and treat drug-induced nausea. 3. Antipsychotics have various other uses, including managing agitated behavior, treating hiccups, managing Tourette disorder, autism-related irritability, bipolar disorder, and schizoaffective disorder. 4. Antipsychotics are absorbed differently, with some being affected by food intake. 5. Antipsychotics are metabolized by the liver, primarily by cytochrome P-450 enzymes. 6. Long-acting injectable formulations of antipsychotics are available for nonadherent patients or outpatients. 7. Adverse effects occur in about 80% of patients and include extrapyramidal effects, tardive dyskinesia, neuroleptic malignant syndrome, drowsiness, confusion, anticholinergic effects, orthostatic hypotension, hormonal disturbances, weight gain, and metabolic changes. 8. Extrapyramidal effects result from the imbalance between dopamine and cholinergic neurotransmission and can be managed with anticholinergic drugs or β-blockers. 9. Tardive dyskinesia is a motor disorder that can occur after long-term antipsychotic use and may be irreversible in some cases. Vesicular monoamine transporter inhibitors are used to manage tardive dyskinesia. 10. Neuroleptic malignant syndrome is a potentially fatal reaction characterized by muscle rigidity, fever, altered mental status, and other symptoms. 11. Antipsychotics can cause drowsiness, confusion, dry mouth, urinary retention, constipation, visual accommodation loss, lowered blood pressure, orthostatic hypotension, hormonal disturbances, and weight gain. 12. Glucose and lipid profiles should be monitored in patients taking antipsychotics, particularly second- generation agents. 13. Antipsychotics may lower the seizure threshold and should be used cautiously in patients with seizure disorders or withdrawal from alcohol. 14. Antipsychotics used in elderly patients with dementia-related behavioral disturbances and psychosis may increase the risk of mortality. 15. Antipsychotics used in patients with mood disorders should be monitored for worsening of mood, suicidal ideation, or behaviors. 16. Maintenance therapy with antipsychotics is recommended for patients who have had multiple psychotic episodes, with second-generation drugs potentially reducing the relapse rate. 17. Different antipsychotics have varying therapeutic uses and indications. Drugs for Epilepsy 1. Epilepsy is the fourth most common neurologic disorder globally, characterized by sudden, excessive, and synchronous discharge of cerebral neurons. 2. Medications are the most widely used treatment for epilepsy, with approximately 75% of patients achieving seizure control with one medication. 3. Seizures can be controlled with one medication in about 75% of patients, but some may require multiple medications or may never achieve complete seizure control. 4. The site of origin of abnormal neuronal firing determines the symptoms experienced during seizures. 5. Seizures can be triggered by changes in physiological factors such as blood gases, pH, electrolytes, blood glucose, as well as environmental factors like sleep deprivation, alcohol intake, and stress. 6. Various causes including genetic, structural, metabolic, and unknown factors can contribute to epilepsy. 7. Antiseizure medications may not be necessary if the underlying cause of a seizure can be determined and corrected. 8. Seizures are classified into focal and generalized seizures. 9. Focal seizures involve only a portion of one hemisphere of the brain and can progress to become bilateral tonic-clonic seizures. 10. Generalized seizures involve abnormal electrical discharges throughout both hemispheres of the brain and can be convulsive or nonconvulsive. 11. Different types of generalized seizures include tonic-clonic, absence, myoclonic, clonic, tonic, and atonic seizures. 12. Antiseizure medications work through mechanisms such as blocking voltage-gated channels, enhancing inhibitory impulses, and interfering with excitatory glutamate transmission. 13. Drug selection for epilepsy treatment is based on seizure classification, patient-specific variables, and drug characteristics. 14. Monotherapy with a single agent is usually initiated for newly diagnosed patients until seizures are controlled or toxicity occurs. 15. If seizures are not controlled with the first medication, alternative monotherapy or combination therapy may be considered. 16. Awareness of available antiseizure medications, their mechanisms of action, pharmacokinetics, drug interactions, and adverse effects is crucial for successful treatment. 17. Several new antiseizure medications have been approved, but their efficacy compared to older drugs is not significantly different. 18. Pharmacokinetic properties and common adverse effects of antiseizure medications should be considered. 19. Antiseizure medications have been associated with the risk of suicidal behavior and ideation. 20. Multiorgan hypersensitivity reactions, characterized by rash, fever, and systemic organ involvement, can occur with antiseizure medications. 21. Specific epilepsy syndromes with symptoms other than seizures exist but are beyond the scope of this chapter. 22. The International League Against Epilepsy provides the standard classification for seizures and epilepsy syndromes. 1. Benzodiazepines bind to GABA inhibitory receptors and are used for emergency or acute seizure treatment. 2. Clonazepam and clobazam may be prescribed as adjunctive therapy for specific types of seizures. 3. Diazepam can be administered rectally to interrupt prolonged generalized tonic-clonic seizures. 4. Brivaracetam is approved for treating focal-onset seizures in adults and has a high affinity for a synaptic vesicle protein. 5. The exact mechanism of action of brivaracetam is unknown, but it is well absorbed orally and metabolized by hydrolysis and CYP2C19. 6. Carbamazepine blocks sodium channels and is effective for treating focal seizures, generalized tonic- clonic seizures, trigeminal neuralgia, and bipolar disorder. 7. Carbamazepine induces its own metabolism, leading to lower blood concentrations at higher doses. 8. Eslicarbazepine is a prodrug converted to the active metabolite eslicarbazepine, approved for focal seizures in adults. 9. Eslicarbazepine is a voltage-gated sodium channel blocker and is eliminated via glucuronidation. 10. Ethosuximide reduces abnormal electrical activity by inhibiting T-type calcium channels and is effective for treating absence seizures. 11. Felbamate has multiple mechanisms of action and is reserved for refractory epilepsies due to the risk of aplastic anemia and hepatic failure. 12. Gabapentin is an analog of GABA and binds to voltage-gated calcium channels. 13. Gabapentin is used as adjunct therapy for focal seizures and postherpetic neuralgia. 14. Lamotrigine blocks sodium and calcium channels and is effective for a wide variety of seizure types. 15. Lamotrigine is metabolized primarily through the UGT1A4 pathway and interacts with other antiseizure medications. 16. Lacosamide stabilizes hyperexcitable neuronal membranes by affecting voltage-gated sodium channels. 17. Lacosamide is approved for adjunctive treatment of focal seizures. 18. The most common adverse events with lacosamide include dizziness, headache, and fatigue. 1. Oxcarbazepine is a prodrug that is rapidly converted to the 10-monohydroxy metabolite responsible for its anticonvulsant activity. 2. The metabolite, MHD, blocks sodium channels and modulates calcium channels. 3. Oxcarbazepine is approved for use in adults and children with focal seizures. 4. It is a less potent inducer of CYP3A4 and UGT than carbamazepine. 5. Hyponatremia is an adverse effect that limits its use in the elderly. 6. Perampanel is a selective α-amino-3-hydroxy-5-methyl-4-isoxazolepropionic acid antagonist used for adjunctive treatment of focal and generalized tonic-clonic seizures. 7. Perampanel has a long half-life and can be taken once daily. 8. It carries a warning for serious psychiatric and behavioral reactions. 9. Phenobarbital enhances the inhibitory effects of GABA-mediated neurons and is used primarily in the treatment of status epilepticus. 10. Primidone is metabolized to phenobarbital and phenylethylmalonamide, both with anticonvulsant activity. 11. Phenytoin blocks voltage-gated sodium channels and is effective for treating focal and generalized tonic- clonic seizures and status epilepticus. 12. Phenytoin induces CYP2C and CYP3A enzyme systems and the UGT enzyme system. 13. Long-term use of phenytoin may lead to CNS depression, gingival hyperplasia, peripheral neuropathies, and osteoporosis. 14. Pregabalin inhibits excitatory neurotransmitter release by binding to the α2δ site of voltage-gated calcium channels. 15. Pregabalin is effective for focal-onset seizures, diabetic peripheral neuropathy, postherpetic neuralgia, and fibromyalgia. 16. Topiramate has multiple mechanisms of action, including sodium channel blockade, calcium current reduction, carbonic anhydrase inhibition, and potential action at glutamate sites. 17. Topiramate is effective for focal and primary generalized epilepsy and is approved for migraine prevention. 1. Vigabatrin acts as an irreversible inhibitor of GABA-T, the enzyme responsible for GABA metabolism. 2. Vigabatrin is associated with visual field loss in a significant percentage of patients. 3. Vigabatrin is only available through physicians and pharmacies participating in the REMS program. 4. Status epilepticus is a life-threatening condition characterized by two or more seizures without recovery of consciousness in between. 5. Emergency treatment for status epilepticus involves the administration of fast-acting medications such as benzodiazepines, followed by slower-acting medications like phenytoin, fosphenytoin, divalproex, or levetiracetam. 6. Women with epilepsy need to consider the effects of antiseizure medications on contraception and pregnancy planning. 7. Several antiseizure medications can increase the metabolism of hormonal contraceptives and may have potential risks during pregnancy, requiring close monitoring and counseling. Anesthetics 1. Different levels of sedation are used to facilitate procedural interventions. 2. Sedation can provide benefits such as reduced anxiety, lack of awareness, muscle relaxation, suppression of reflexes, and analgesia. 3. Multiple categories of drugs are combined to achieve the desired level of sedation. 4. Drug selection for sedation depends on the procedure, patient characteristics, and medical conditions. 5. Preoperative medications provide anxiolysis, analgesia, and mitigate unwanted side effects. 6. Neuromuscular blockers are used to facilitate endotracheal intubation and muscle relaxation during surgery. 7. Potent general anesthetic medications are administered via inhalation or intravenously. 8. Sedation levels occur in a dose-related continuum from light sedation to general anesthesia. 9. Escalation to higher levels of sedation is characterized by changes in mentation, hemodynamic stability, and respiratory competency. 10. General anesthesia is a reversible state of CNS depression, causing loss of response to stimuli. 11. General anesthesia has three stages: induction, maintenance, and recovery. 12. Induction involves administering an IV agent like propofol to induce unconsciousness. 13. Maintenance of anesthesia involves monitoring vital signs and adjusting drug dosage to maintain the desired level of anesthesia. 14. Inhalation anesthetics are used primarily for maintenance of anesthesia and can be rapidly altered to adjust the depth of anesthesia. 15. Inhalation anesthetics are nonflammable and include nitrous oxide and volatile, halogenated hydrocarbons. 16. Potency of anesthetics is measured by the minimum alveolar concentration (MAC). 17. Factors such as age, hypothermia, and concurrent medications can affect the patient's sensitivity to anesthetics. 18. Uptake and distribution of inhalation anesthetics aim to achieve a constant and optimal brain partial pressure. 19. Alveolar wash-in and anesthetic uptake contribute to the time course of achieving steady-state levels of anesthesia. 20. Anesthetic gas transfer occurs as the partial pressure builds within the lungs. 1. The blood:gas partition coefficient determines the solubility of anesthetic in blood. 2. Anesthetics with low blood solubility, like nitrous oxide, induce anesthesia more rapidly. 3. Cardiac output (CO) affects the rate of induction of inhaled anesthetics. 4. Higher CO results in faster transport of the drug to the brain, while lower CO slows down induction. 5. The alveolar-to-venous partial pressure gradient affects the induction time. 6. If a large gradient persists, induction time is longer due to high tissue uptake. 7. Tissue type influences the time course of anesthetic uptake. 8. Highly perfused tissues (brain, heart, liver, etc.) reach steady state rapidly. 9. Skeletal muscles have moderately perfused tissue with a large storage capacity, leading to longer induction times. 10. Fat has poor perfusion but a large storage capacity, resulting in prolonged induction times. 11. Vessel-poor tissues (bone, ligaments, cartilage) have minimal impact on anesthetic distribution. 12. Washout refers to the exhalation of anesthetic gases from the body. 13. The time course of washout depends on factors such as uptake and equilibrium of the inspired anesthetic. 14. General anesthetics enhance the sensitivity of GABAA receptors. 15. Nitrous oxide and ketamine act on NMDA receptors instead of GABAA receptors. 16. Isoflurane produces hypotension, is not metabolized significantly, and has a pungent odor. 17. Desflurane has low blood solubility, rapid onset and recovery, and is administered via a heated vaporizer. 18. Sevoflurane has low respiratory irritation and is useful for inhalation induction, especially in pediatric patients. 1. Nitrous oxide is a sedative but cannot induce general anesthesia. 2. It is often used in dentistry at concentrations of 30% to 50%. 3. Nitrous oxide does not depress respiration and maintains cardiovascular hemodynamics and muscular strength. 4. It can be combined with other inhalational agents to establish general anesthesia. 5. Nitrous oxide rapidly moves in and out of the body due to its poor solubility in blood and tissues. 6. It can increase volume or pressure in closed body compartments and cause diffusion hypoxia during recovery. 7. Diffusion hypoxia can be overcome by delivering high concentrations of inspired oxygen. 8. Malignant hyperthermia (MH) is a life-threatening condition induced by halogenated hydrocarbon anesthetics. 9. MH causes uncontrolled increase in skeletal muscle oxidative metabolism. 10. Susceptibility to MH is often inherited and caution should be taken in individuals with certain conditions. 11. Dantrolene is given to block the release of Ca2+ and reduce heat production during MH. 12. IV anesthetics cause rapid induction of anesthesia and may be used for short procedures or as infusions for longer surgeries. 13. IV induction agents transfer from blood to the brain based on their unbound free concentration, lipid solubility, and degree of ionization. 14. Recovery from IV anesthetics is due to redistribution from the CNS and other vessel-rich tissues. 15. Skeletal muscle uptake of IV anesthetics leads to a fall in plasma concentration and allows drug diffusion out of the CNS. 16. Adipose tissue contributes less to early drug redistribution. 17. Reduced cardiac output diverts more blood to the cerebral circulation, requiring a reduced dose of IV anesthetics. 18. Decreased cardiac output prolongs the time for induction drugs to reach the brain. 19. Propofol is an IV sedative/hypnotic used for induction and maintenance of anesthesia. 20. Induction with propofol is smooth and occurs rapidly. 21. Propofol depresses the CNS but may contribute to excitatory phenomena and transient pain at the injection site. 22. It decreases blood pressure, reduces intracranial pressure, and has less impact on CNS-evoked potentials. 23. Supplementary analgesia is required with propofol as it does not provide analgesia on its own. 24. Propofol has antiemetic properties and a low incidence of postoperative nausea and vomiting. 25. Thiopental is an ultra-short-acting barbiturate used for anesthesia induction. 26. Barbiturates quickly enter the CNS and depress function but can also rapidly redistribute to other tissues. 27. Only about 15% of a dose of barbiturates is metabolized by the liver per hour. 28. Barbiturates tend to decrease blood pressure and decrease intracranial pressure. 29. Thiopental is no longer available in many countries, including the United States. 30. Methohexital is commonly used for electroconvulsive therapy. 1. Benzodiazepines like midazolam, diazepam, and lorazepam are used for sedation and amnesia during anesthesia. 2. Benzodiazepines enhance the effects of inhibitory neurotransmitters like GABA. 3. They have minimal cardiovascular effects but can cause respiratory depression. 4. Benzodiazepines are metabolized by the liver and have variable elimination half-lives. 5. Erythromycin can prolong the effects of midazolam. 6. Opioids like fentanyl, sufentanil, and remifentanil are commonly combined with other anesthetics for analgesia. 7. Opioids can cause hypotension, respiratory depression, nausea, and vomiting. 8. Naloxone can be used to reverse the effects of opioids. 9. Etomidate is a hypnotic agent used for anesthesia induction without analgesic activity. 10. It has little effect on the heart and systemic vascular resistance. 11. Etomidate is used in patients with cardiovascular dysfunction or acute critical illness. 12. It inhibits 11-β hydroxylase involved in steroidogenesis and may decrease plasma cortisol and aldosterone levels. 13. Ketamine is a short-acting anesthetic and analgesic that induces a dissociated state. 14. It stimulates central sympathetic outflow and is beneficial in hypovolemic or cardiogenic shock and asthma. 15. Ketamine is contraindicated in hypertensive or stroke patients. 16. Dexmedetomidine is a sedative with analgesic and anxiolytic effects used in intensive care and surgery. 17. It reduces anesthetic requirements without significant respiratory depression. 18. Dexmedetomidine is effective in blunting emergence delirium in pediatric patients. 19. Neuromuscular blockers are used to facilitate intubation and provide muscle relaxation during surgery. 20. Local anesthetics block nerve conduction of sensory and motor impulses. 21. They include drugs like bupivacaine, lidocaine, mepivacaine, ropivacaine, and tetracaine. 22. Adding epinephrine as a vasoconstrictor to local anesthetics decreases absorption, prolongs action, and reduces systemic toxicity. 1. The onset of action of local anesthetics is influenced by factors such as tissue pH, nerve morphology, concentration, pKa, and lipid solubility. 2. Local anesthetics with a lower pKa have a quicker onset of action. 3. Potency and duration of local anesthetics depend on lipid solubility. 4. Amide local anesthetics are primarily metabolized in the liver, while esters are metabolized by plasma cholinesterase. 5. Patients with pseudocholinesterase deficiency may metabolize ester local anesthetics more slowly. 6. True allergy to an amide local anesthetic is rare, while the ester procaine is more allergenic. 7. Local anesthetic systemic toxicity (LAST) can occur due to repeated injections or inadvertent IV injection. 8. Toxic blood levels of local anesthetics should be calculated based on weight, especially in children, the elderly, and women in labor. 9. Aspiration before every injection is crucial to prevent LAST. 10. Signs and symptoms of LAST are unpredictable and can include altered mental status, seizures, and cardiovascular instability. 11. Treatment for LAST may include seizure suppression, airway management, cardiopulmonary support, and lipid rescue therapy. 12. Adjuncts in anesthesia include drugs that affect gastrointestinal motility, postoperative nausea and vomiting (PONV), anxiety, and analgesia. 13. H2-receptor antagonists, proton pump inhibitors, and nonparticulate antacids can reduce gastric acidity in case of aspiration. 14. Dopamine receptor antagonists can be used to speed gastric emptying. 15. 5-HT3 receptor antagonists, anticholinergics, antihistamines, glucocorticoids, and neurokinin-1 antagonists are used to prevent PONV. 16. Transdermal scopolamine can be given preoperatively to patients at high risk for PONV. 17. Benzodiazepines, α2 agonists, and H1-receptor antagonists can be used to alleviate anxiety. 18. Benzodiazepines also induce anterograde amnesia. 19. Multimodal analgesia is becoming more common to reduce opioid consumption in surgical patients. 20. Nonsteroidal anti-inflammatory drugs (NSAIDs) are common adjuncts to opioids for pain control. 21. Acetaminophen can be used orally or intravenously for analgesia. 22. Gabapentin and pregabalin can reduce opioid consumption and have multiple uses in pain management. 23. Ketamine is used to reduce overall opioid consumption and is an NMDA antagonist. Opioids 1. Pain management is a major challenge in clinical medicine. 2. Pain can be acute or chronic and is the result of complex neurochemical processes. 3. Pain is subjective, relying on the patient's perception and description. 4. Nociceptive pain (e.g., arthritic pain) can be alleviated with nonopioid analgesics like NSAIDs. 5. Neuropathic pain responds best to anticonvulsants, tricyclic antidepressants, or serotonin/norepinephrine reuptake inhibitors. 6. Opioids can be considered for severe acute or chronic malignant/nonmalignant pain. 7. Opioids bind to specific receptors in the CNS and mimic the action of endogenous neurotransmitters. 8. Opioids have a range of effects and are primarily used to relieve intense pain. 9. Opioids can be abused due to their euphoric properties. 10. Opioid antagonists are important for reversing opioid overdose. 11. Opioids primarily act on μ, κ, and δ receptors in the CNS. 12. The analgesic properties of opioids are primarily mediated by μ receptors. 13. Opioids can cause respiratory depression, euphoria, and depression of the cough reflex. 14. Opioids can cause miosis (pinpoint pupils), emesis (vomiting), and affect the GI tract. 15. Morphine is a strong μ receptor agonist, while codeine is a weak μ opioid agonist. 16. Opioids exert analgesic effects by interacting with opioid receptors in the CNS. 17. Morphine and other opioids raise the pain threshold and alter the brain's perception of pain. 18. Morphine has various effects on respiration, cough reflex, pupil size, GI tract, cardiovascular system, histamine release, and hormonal balance. 19. Prolonged morphine use may lead to opioid-induced androgen deficiency. 20. Morphine may prolong the second stage of labor. 21. Morphine has a linear pharmacokinetic profile and is absorbed slowly when taken orally. 22. Morphine is conjugated in the liver and excreted as active metabolites. Common adverse effects of opioids include respiratory depression, constipation, and the need for caution in patients with liver or renal dysfunction. 1. Repeated use of morphine leads to tolerance to various effects, except for miosis (pupil constriction) and constipation. 2. Morphine and other agonists can cause physical and psychological dependence, with withdrawal symptoms that can be severe. 3. Morphine's depressant actions are enhanced when combined with CNS depressant medications like phenothiazines, MAOIs, and benzodiazepines. 4. Opioid prescribing guidelines advise against simultaneous use of opioids and benzodiazepines due to the dangerous combination. 5. Codeine is a naturally occurring opioid and a weaker analgesic compared to morphine, often used for mild to moderate pain. 6. Codeine is converted to morphine by the CYP2D6 enzyme, and individuals with high CYP2D6 activity may experience higher levels of morphine, potentially leading to overdose. 7. Codeine has been associated with life-threatening respiratory depression and death in children, particularly after tonsillectomy and/or adenoidectomy. 8. Dextromethorphan is a synthetic cough suppressant with no analgesic action and lower abuse potential compared to codeine. 9. Oxymorphone and oxycodone are semisynthetic analogs of morphine and codeine, respectively, with varying potencies. 10. Oxymorphone is approximately ten times more potent than morphine when given parenterally, but its oral potency drops to about three times that of morphine. 11. Oxycodone is approximately two times more potent than morphine and is available in immediate-release and extended-release formulations. 12. Hydromorphone is 4 to 7 times more potent than morphine when taken orally and is preferred in patients with renal dysfunction. 13. Hydrocodone is a weaker analgesic than hydromorphone, with similar oral efficacy to morphine and often combined with acetaminophen or ibuprofen. 14. Fentanyl is a synthetic opioid with 100-fold the analgesic potency of morphine, used for anesthesia and acute pain management. 15. Fentanyl has a rapid onset and short duration of action, and it is commonly administered intravenously or epidurally. 16. Sufentanil, alfentanil, remifentanil, and carfentanil are synthetic opioid agonists related to fentanyl, with varying potencies and uses. 17. Methadone is a synthetic opioid with variable equianalgesic potency compared to morphine, used for pain treatment, opioid withdrawal, and maintenance therapy. 18. Methadone has a longer duration of action and a milder but more protracted withdrawal syndrome compared to other opioids. 19. Methadone is well absorbed orally, has a long half-life ranging from 12 to 40 hours, and can accumulate with repeated dosing, potentially leading to toxicity. 20. Methadone can produce physical dependence, has less neurotoxicity than morphine, and can affect the QTc interval, requiring baseline and routine ECG monitoring. 1. Meperidine is a lower-potency synthetic opioid used for acute pain management. It acts primarily as a κ agonist with some μ agonist activity. 2. Meperidine has anticholinergic effects and is associated with an increased risk of delirium compared to other opioids. 3. Meperidine has an active metabolite called normeperidine, which can be neurotoxic and may lead to delirium, hyperreflexia, myoclonus, and seizures in patients with renal insufficiency. 4. Meperidine should only be used for short-term pain management (≤48 hours) due to its short duration of action and potential for toxicity. 5. Meperidine should not be used in elderly patients, those with renal or hepatic insufficiency, preexisting respiratory compromise, or those taking MAOIs. 6. Serotonin syndrome can occur when meperidine is combined with selective serotonin reuptake inhibitors (SSRIs). 7. Buprenorphine is a potent partial agonist at the μ receptor and an antagonist at the κ receptors. It has a longer duration of action and a lower abuse potential compared to full agonists. 8. Buprenorphine can displace full μ agonists, leading to withdrawal symptoms in opioid-dependent patients. 9. Buprenorphine provides a "ceiling effect" and has a lower risk of respiratory depression compared to full agonists, except when combined with CNS depressants like benzodiazepines. 10. Buprenorphine is available in various formulations and is approved for moderate to severe pain and medication-assisted treatment of opioid addiction. 11. Pentazocine acts as an agonist on κ receptors and a weak antagonist or partial agonist at μ receptors. It produces less euphoria than morphine but can cause respiratory depression, increased blood pressure, tachycardia, and hallucinations at higher doses. 12. Nalbuphine and butorphanol are mixed opioid agonist–antagonists that have limited roles in chronic pain treatment. They have a lower propensity for psychotomimetic effects compared to pentazocine. 13. Tramadol is a centrally acting analgesic that binds to the μ opioid receptor and inhibits norepinephrine and serotonin reuptake weakly. It has less respiratory-depressant activity than morphine. 14. Tramadol metabolism produces an active metabolite with a higher affinity for the mu receptor. Naloxone can only partially reverse tramadol toxicity. 15. Tramadol should be used with caution in patients with a history of seizures and can be associated with misuse and abuse. 16. Opioid antagonists bind to opioid receptors but do not activate the receptor-mediated response. They rapidly reverse the effects of agonists and precipitate opioid withdrawal symptoms in opioid-dependent patients. 1. Naloxone is a competitive antagonist at μ, κ, and δ receptors and can rapidly reverse the effects of morphine overdose, including respiratory depression and coma. 2. Naloxone can be administered intravenously, intramuscularly, subcutaneously, and intranasally, with varying onset times. 3. Oral naloxone has little to no clinical effect due to extensive first-pass metabolism. 4. Naloxone's half-life ranges from 30 to 81 minutes, and the duration of its effect depends on the opioid ingested and dosage form. 5. Higher doses and continuous administration of naloxone may be needed to reverse the effects of buprenorphine due to its high affinity for the mu receptor. 6. Naloxone is available in autoinjector and nasal inhaler forms for community distribution to treat opioid overdose. 7. Prescribers should educate patients and family members about the availability and proper use of naloxone products and emphasize the importance of calling emergency services in case of overdose. 8. Naltrexone has similar actions to naloxone but has a longer duration of action and can be taken orally. 9. A single oral dose of naltrexone can block the effects of injected heroin for up to 24 hours, and the intramuscular formulation can block the effects for 30 days. 10. Naltrexone can cause hepatotoxicity, so monitoring of hepatic function is recommended. CNS Stimulants 1. Psychomotor stimulants and hallucinogens are two groups of drugs that primarily stimulate the central nervous system (CNS). 2. Psychomotor stimulants, such as caffeine, cause excitement, euphoria, decreased fatigue, and increased motor activity. 3. Hallucinogens produce profound changes in thought patterns and mood, with little effect on the brainstem and spinal cord. 4. Methylxanthines, including caffeine, theophylline, and theobromine, are a subgroup of psychomotor stimulants found in tea, coffee, cocoa, and other products. 5. Methylxanthines act through mechanisms such as calcium translocation, inhibition of phosphodiesterase, and adenosine receptor blockade. 6. Caffeine stimulates the cortex and other areas of the brain, leading to decreased fatigue and increased mental alertness. 7. Caffeine has positive inotropic and chronotropic effects on the heart and mild diuretic action. 8. Methylxanthines stimulate gastric acid secretion and should be avoided by individuals with peptic ulcers. 9. Caffeine and its derivatives have therapeutic uses, such as bronchial relaxation and headache management. 10. Methylxanthines are well absorbed orally, distribute throughout the body, cross the placenta and are excreted in breast milk, metabolized in the liver, and excreted in urine. 11. Adverse effects of caffeine include insomnia, anxiety, agitation, and toxicity at high doses. 12. Nicotine is the active ingredient in tobacco and is the second most widely used CNS stimulant after caffeine. 13. Nicotine acts through ganglionic stimulation and blockade, affects the CNS and peripheral systems, and is highly lipid soluble. 14. Nicotine increases blood pressure, heart rate, and bowel activity, and it has adverse effects on hypertensive and peripheral vascular disease patients. 15. Nicotine is absorbed via various routes, including the oral mucosa, lungs, GI mucosa, and skin, and is metabolized and cleared from the body. 16. Adverse effects of nicotine include irritability, tremors, intestinal cramps, increased heart rate and blood pressure, and drug metabolism interactions. 17. Nicotine withdrawal symptoms include irritability, anxiety, restlessness, difficulty concentrating, headaches, insomnia, appetite changes, and GI upset. 18. Nicotine replacement therapies, such as transdermal patches and chewing gum, can help reduce withdrawal symptoms and aid in smoking cessation. 19. Varenicline is a partial agonist at neuronal nicotinic acetylcholine receptors and is used as an adjunct in smoking cessation therapy. 20. Cocaine is a highly addictive drug that blocks the reuptake of monoamines, leading to prolonged effects on the CNS and peripheral systems. 21. Chronic cocaine intake depletes dopamine and triggers craving for the drug. 22. Amphetamine and its derivatives, such as dextroamphetamine and methamphetamine, have effects similar to cocaine and act by releasing and inhibiting the reuptake of catecholamines. 23. Amphetamine elevates the levels of catecholamine neurotransmitters in synaptic spaces and has behavioral effects similar to cocaine. 24. The behavioral effects of amphetamine and its derivatives are similar to those of cocaine, despite different mechanisms of action. 25. MDMA (ecstasy) is a synthetic derivative of methamphetamine with stimulant and hallucinogenic properties. 26. MDMA acts by releasing serotonin, dopamine, and norepinephrine and has both stimulant and hallucinogenic effects. 27. The effects of amphetamine and MDMA are indirect and depend on elevating the levels of catecholamine neurotransmitters. 28. Amphetamine and its derivatives have neurologic and clinical effects similar to cocaine. 29. Amphetamine is available for prescription use, while MDMA is a recreational drug. 30. The addictive nature of amphetamine and its derivatives, as well as cocaine and nicotine, can lead to physical dependence and severe withdrawal symptoms. 1. Amphetamine stimulates the entire cerebrospinal axis, leading to increased alertness, decreased fatigue, depressed appetite, and insomnia. 2. Amphetamine acts on the adrenergic system, indirectly stimulating the receptors through norepinephrine release. 3. Amphetamine and its derivatives are used to treat hyperactivity in children, narcolepsy, and obesity. 4. High doses of amphetamine can cause psychosis and convulsions. 5. Medications like dextroamphetamine, methamphetamine, mixed amphetamine salts, and methylphenidate are used to improve attention span and alleviate behavioral problems in children with ADHD. 6. Lisdexamfetamine is a prodrug converted to dextroamphetamine for the treatment of ADHD. 7. Atomoxetine is a nonstimulant drug used for ADHD that selectively inhibits norepinephrine reuptake. 8. Narcolepsy can be treated with medications like mixed amphetamine salts, methylphenidate, modafinil, and armodafinil. 9. Modafinil promotes wakefulness with fewer psychoactive effects compared to other CNS stimulants. 10. Phentermine and diethylpropion are sympathomimetic amines used for appetite suppression in obesity management. 11. Amphetamine is well absorbed from the gastrointestinal tract, metabolized by the liver, and excreted in the urine. 12. Amphetamine abuse through IV injection or smoking can cause euphoria lasting longer than the effects of cocaine. 13. Amphetamines can lead to addiction, dependence, tolerance, and drug-seeking behavior. 14. Adverse effects of amphetamine usage include insomnia, irritability, weakness, dizziness, tremor, confusion, delirium, panic states, and suicidal tendencies. 15. Amphetamine can cause cardiovascular effects such as palpitations, arrhythmias, hypertension, anginal pain, and circulatory collapse. 16. Gastrointestinal effects of amphetamine include anorexia, nausea, vomiting, abdominal cramps, and diarrhea. 17. Patients with certain medical conditions or taking MAO inhibitors should not be treated with amphetamine. 18. Methylphenidate is a CNS stimulant used for the treatment of ADHD and narcolepsy, and it inhibits the reuptake of dopamine and norepinephrine.