HVAC Simplified PDF - Design of HVAC Systems
Document Details
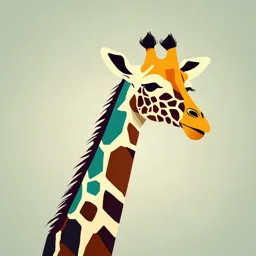
Uploaded by WiseMatrix
2012
Stephen P. Kavanaugh
Tags
Summary
This book details the design of heating, ventilating, and air-conditioning (HVAC) systems, focusing on energy efficiency and economic value. It covers equipment selection, comfort, building assemblies, and calculation procedures and includes helpful spreadsheet programs on a CD.
Full Transcript
This text on the design of heating, ventilating, and air-conditioning (HVAC) systems emphasizes energy efficiency and economic value. Topics include equipment selection and specification, comfort and indoor air quality, building assemblies, heating a...
This text on the design of heating, ventilating, and air-conditioning (HVAC) systems emphasizes energy efficiency and economic value. Topics include equipment selection and specification, comfort and indoor air quality, building assemblies, heating and cooling loads, electrical and control systems, and design of air distribution and water distribution systems. The author has assembled information from ASHRAE Handbooks, ASHRAE standards, and manufacturers’ data and presents design HVAC HVAC Simplified procedures commonly used by professional engineers, including an overview of ASHRAE Standard 62.1-2004 mandates for ventilation air system design. This book includes a CD containing a suite of complementary spreadsheet programs that incorporate design and computation procedures from the text. These programs Simplified include psychrometric analysis, equipment selection, heating and cooling load calculation, an electronic “ductulator,” piping system design, a ductwork cost calculator, and programs to evaluate building system demand and energy efficiency. The CD is Windows and Mac compatible and is Single User only. The intended audience for this book includes novice HVAC engineers who did not complete an undergraduate HVAC course, engineers moving into HVAC from other fields, undergraduate engineering students in design-oriented curriculums, and non- engineering professionals with strong building-related experience. The author, Stephen P. Kavanaugh, PhD, Fellow ASHRAE, is a professor of mechanical engineering at the University of Alabama where he teaches HVAC. Stephen P. Kavanaugh Kavanaugh ISBN 978-1-931862-97-4 Product code: 90439 02/12 Errata noted in the list dated 02/13/2012 have been corrected. HVAC Simplified.indd 1 2/13/2012 5:38:29 PM HVAC Simplified Stephen P. Kavanaugh American Society of Heating, Refrigerating and Air-Conditioning Engineers, Inc. This publication was prepared in cooperation with TC 7.1, Integrated Building Design. About the Author Stephen P. Kavanaugh, PhD, Fellow ASHRAE, has been a professor of mechanical engineering at The Univer- sity of Alabama since 1985, where he teaches HVAC and is faculty advisor for the ASHRAE Student Chapter as well as a Habitat for Humanity Student Affiliate. Kavanaugh is co-author of Ground-Source Heat Pumps—Design of Geothermal Systems for Commercial and Institutional Buildings, published by ASHRAE in 1997. He has presented over 100 engineering seminars for more than 2,500 designers on the topics of energy efficiency, ground-source heat pumps, and HVAC. He maintains the Web site www.geokiss.com, where there is more information about HVAC and ground-source heat pump design tools. He is past chair and current program chair of ASHRAE Technical Committee 6.8, Geothermal Energy, as well as past chair of ASHRAE Technical Committee 9.4, Applied Heat Pumps and Heat Recovery. Kavanaugh is also a Fellow of the American Society of Mechanical Engineers and a board member and past president of Habitat for Humanity—Tuscaloosa. Any updates/errata to this publication will be posted on the ASHRAE Web site at www.ashrae.org/publicationupdates. This reprint incorporates all errata dated March 25, 2008, and previous. HVAC Simplified ISBN 10: 1-931862-97-4 ISBN 13: 978-1-931862-97-4 ©2006 American Society of Heating, Refrigerating and Air-Conditioning Engineers, Inc. 1791 Tullie Circle, NE Atlanta, GA 30329 www.ashrae.org All rights reserved. Printed in the United States of America ASHRAE has compiled this publication with care, but ASHRAE has not investigated, and ASHRAE expressly disclaims any duty to investigate, any product, service, process, procedure, design, or the like that may be described herein. The appearance of any technical data or editorial material in this publication does not constitute endorsement, warranty, or guaranty by ASHRAE of any product, service, process, procedure, design, or the like. ASHRAE does not warrant that the information in the publication is free of errors, and ASHRAE does not necessarily agree with any statement or opin- ion in this publication. The entire risk of the use of any information in this publication is assumed by the user. No part of this book may be reproduced without permission in writing from ASHRAE, except by a reviewer who may quote brief passages or reproduce illustrations in a review with appropriate credit; nor may any part of this book be repro- duced, stored in a retrieval system, or transmitted in any way or by any means—electronic, photocopying, recording, or other—without permission in writing from ASHRAE. Library of Congress Cataloging-in-Publication Data Kavanaugh, Stephen P. HVAC simplified / Stephen P. Kavanaugh. p. cm. ISBN 1-931862-97-4 (softcover) 1. Heating. 2. Ventilation. 3. Air conditioning. I. Title. TH7011.K38 2005 697--dc22 2005037135 ASHRAE STAFF SPECIAL PUBLICATIONS PUBLISHING SERVICES Mildred Geshwiler David Soltis Editor Manager Christina Helms Tracy Becker Associate Editor Graphic Applications Specialist Cindy Sheffield Michaels Jayne Jackson Editorial Assistant Production Assistant Michshell Phillips PUBLISHER Administrative Assistant W. Stephen Comstock Contents Preface..................................................................................... vii Author’s Note.................................................................................ix Acknowledgments.............................................................................xi 1 Introduction to HVAC....................................................................... 1 2 HVAC Fundamentals: Refrigeration............................................................ 5 3 HVAC Fundamentals: Heat Transfer........................................................... 19 4 HVAC Fundamentals: Psychrometrics.......................................................... 27 5 HVAC Equipment, Systems, and Selection...................................................... 37 6 Comfort, Air Quality, and Climatic Data........................................................ 67 7 Heat and Moisture Flow in Buildings.......................................................... 81 8 Cooling Load and Heating Loss Calculations and Analysis........................................ 103 9 Air Distribution System Design.............................................................. 121 10 Water Distribution System Design............................................................ 145 11 Motors, Lighting, and Controls.............................................................. 169 12 Energy, Costs, and Economics............................................................... 189 Appendix A Units and Conversions............................................................. 209 Appendix B Refrigerant Pressure-Enthalpy Charts and Sea Level Psychrometric Chart.................... 213 Appendix C Floor Plans...................................................................... 219 Appendix D Developing Engineering Tools with Computer Spreadsheets............................... 227 Nomenclature—HVAC Terms, Abbreviations, and Subscripts......................................... 229 Index...................................................................................... 231 v Preface The text contains twelve chapters, including three short chapters that review the fun- damentals of refrigeration, heat transfer, and psychrometrics. Information from the ASHRAE Handbook—Fundamentals is summarized and supplemented with items from industry sources. The remaining chapters assemble information from ASHRAE hand- books, standards, manufacturer data, and design procedures commonly used by profes- sional engineers. Topics include equipment selection and specification, comfort and IAQ, building assemblies, heating and cooling loads, air distribution system design, water distri- bution system design, electrical and control systems, design for energy efficiency, and design for economic value. A suite of complementary spreadsheet programs that incorporate design and compu- tation procedures from the text are provided on the CD that accompanies this book. These programs include psychrometric analysis, equipment selection, heating and cooling load calculation, an electronic “ductulator,” piping system design, a ductwork cost calculator, and programs to evaluate building system demand and energy efficiency. Future updates to these programs can be found at www.ashrae.org/updates. TARGET AUDIENCES Novice HVAC engineers that did not complete an HVAC course during their under- graduate program Engineers moving into the HVAC field from other areas Undergraduate engineering students in design-oriented curriculums Non-engineering professionals with strong building-related experience DISTINGUISHING FEATURES The text places an emphasis on design and the energy and economic evaluation of results. A large amount of information is provided so that instructors and students do not have to frequently consult supplemental documents in order to complete HVAC system design. For example, one section provides an overview of the revised 2004 ASHRAE Standards 62.1 and 62.2 ventilation air system design mandates, a feature not even included in the ASHRAE Handbooks. Also, a large amount of manufacturer performance data is provided with correction methods when conditions vary from rated values. Performance data are provided for packaged ACs, RTUs, air and water heat pumps, furnaces, chillers (six prod- uct lines), compressors, cooling towers, hydronic coils, pumps, fans, electric motors, light- ing products, and other equipment. The text concludes with a chapter on design evaluation methods. Sections in this chapter include HVAC system demand computations, a discussion of simplified energy analysis, operating costs (including tables of maintenance, repair, and replacement costs), a presentation of methods to compute system installation costs (with references to more detailed sources), and economic analysis methods. vii Author’s Note This text is an attempt to provide an understanding of fundamental HVAC concepts and to extend these principles to the explanation of simple design tools used to create building systems that are efficient and provide comfortable and healthy environments. My decision to include the term “Simplified” in the title is rooted in my own preference for simple and creative systems. However, this philosophy was perhaps better expressed by ASHRAE Presidential member Bill Coad when he quoted Albert Einstein: “Everything should be as simple as possible, but no simpler.”1 Certainly, there are procedures and tools that provide higher levels of accuracy and detail than those I have included in this text. There are also some that are more straight- forward and less complicated. However, I have attempted to provide a middle ground with regard to the level of difficulty by avoiding oversimplifications that are not suited to design and more complex methods that are more suited to analytical research. More importantly, I encourage readers to extend this philosophy of simplification to building designs and HVAC systems. In some applications, complexity cannot be avoided. However, options also exist to extend complex technologies to modest buildings in which the added benefit is not economically justifiable for a client with limited financial and staffing resources. The engineer must apply the pearl of wisdom of Einstein to best meet the needs of 21st Century. Steve Kavanaugh December 2005 1. Coad, W.J. 1996. Indoor Air Quality: A Design Parameter. ASHRAE Journal 38(6):39–47. ix Acknowledgments I wish to thank the cadre of volunteer reviewers that were assembled by the cognizant ASHRAE technical committee, Integrated Building Design (TC 7.1), which provided a wealth of corrections and suggestions on a very compacted time schedule. Core committee members include Charles E. Gulledge III (Chair), Bill Williams, Dennis Knight, Peter Gryc, Bert Phillips, Robert McDowell, Jesse Sandhu, Stirling Walkes, and Chris Ott. Additionally, the members of the ASHRAE Publications Committee and staff have provided encouragement and support for this effort. I also wish to thank my employer, The University of Alabama, for providing a sabbatical for the Spring 2004 semester, during which I completed the first five chapters of this work. I also wish to thank the many stu- dents; my mentors Drs. Jerald Parker, Harry Mei, and Gene Martinez; and my ASHRAE colleagues, who have encouraged me to do a better job of presenting the ideas and con- cepts that have helped me learn. Finally, I wish to express my special gratitude to Barbara Hattemer McCrary, my former student who became my teacher in the use of spreadsheets as an engineering tool, and Kevin Rafferty, who reviewed the entire book and has provided a wealth of technical and philosophical insight throughout our many years of collabora- tion. xi 1 Introduction to HVAC The challenges presented to engineers in the heat- man and the highest standards of professional ing, ventilating, and air-conditioning (HVAC) industry conduct; have much in common with those in other technical …place service before profit, the honor and fields. HVAC engineers face constraints imposed by rap- standing of the profession before professional idly changing technologies, increased liability, a height- advantage, and the public welfare above all ened emphasis on short-term profitability, complexity in other considerations.... codes and standards, and an overabundance of informa- tion and misinformation. However, the public nature of The ASHRAE Code of Ethics (ASHRAE 2004a) states: the HVAC industry also presents many opportunities for As members of a Society, the engineer to be creative and influential. “organized and operated for the exclusive The successful HVAC engineer must be technically purpose of advancing the arts and sciences of competent and conscientiously diligent in pursuit of heating, refrigeration, air conditioning, and optimum designs. However, in the 21st century, engi- ventilation, the allied arts and sciences, and neers must also be persuasive, to ensure their expertise related human factors for the benefit of the is respected, passionate in their concern for the public general public” [ASHRAE 2004b], health and environment, and open to all perspectives in We recognize that honesty, fairness, courtesy, pursuit of high quality and economically viable solu- competence and integrity must characterize tions. HVAC engineers encounter a wide variety of indi- our conduct. With the foregoing in mind viduals ranging from owners of modest homes to Our efforts shall be directed at all employees of large industrial complexes. They must times to the enhancement of the communicate and appreciate the needs of the broadest public health, safety and welfare. range of people because their clients include everyone Our services shall be offered only in who inhabits buildings. areas of our competence. In the real world there are many ethical conflicts, Our products shall be offered only and although ethical lapses in legal, political, medical, in areas of their suitability. and other professions are often more publicized, the Our public statements shall be HVAC industry is not without the potential for malprac- issued only in an objective and tice, conflict of interest, and dishonesty. Examples of truthful manner. inferior workmanship and unnecessarily inflated costs Our endeavors shall carefully avoid are evident in the industry. Rules, codes, standards, and conflicts of interest and the appear- guidelines evolve with input from those having self- ance of conflicts of interest. serving agendas as well as those seeking the common The confidentiality of clients’ and good. Organizations such as the American Society of employers’ business affairs, propri- Heating, Refrigerating and Air-Conditioning Engineers etary information, and procedures (ASHRAE) and the National Society of Professional shall be respected. Engineers (NSPE) offer guidance to members for ethical behavior. A summary is offered here as a starting point Approved by the Board of Directors for HVAC design. June 22, 1986 ENGINEERS’ CREED AND ASHRAE CODE OF ETHICS THE BUILDING INDUSTRY ENVIRONMENT An excerpt from the Engineers’ Creed of the The HVAC engineer operates in a world in which National Society of Professional Engineers (NSPE) technology is rapidly changing and conventional roles, calls on the engineer to: social behavior, working relationships, and partnerships … give the utmost performance; are being challenged and reinvented. Figure 1.1 is an … participate in none but honest enterprise; organizational chart of conventional working relation- … live and work according to the laws of ships for a large building project. The building owner’s HVAC Simplified principal point of contact is with an architect. Architects building type, location, and accessories (Means 2004). are the lead designers and project managers. They will Although the architects are responsible for designing a hire the engineers to design the building components in building that is within budget, this may not be possible their areas of expertise. A mechanical design firm is typ- if the owners desire more than they can afford. ically responsible for the HVAC systems and interre- lated plumbing systems (water, hot water, gas or oil Two Design Processes piping, steam distribution, fire sprinklers, waste water, etc.). Design and construction teams often include many In the plan-bid process, the owner or developer additional specialists not noted in Figure 1.1. hires the architect and the architect hires engineers to Design consists of design the building and provide drawings and specifica- tions for contractor and subcontractor bidding. Steps can communicating with the client(s) to determine be taken to prequalify suitable contractors and subcon- needs and requirements, tractors, or the invitation to provide bids can be open to assessing the situation and performing computa- any firm. Qualified general contractors, in cooperation tions to determine the most appropriate systems and with subcontractors, review drawings and submit com- options, petitive bids. If the low bid is acceptable, building pro- taking steps to comply with building codes and reg- ceeds. ulations, and communicating design results and intent with draw- A building has a good chance of being a long-last- ings and specifications. ing, low-maintenance, quality structure, which is com- pleted on time and within budget, if An important component in this process is to attempt to design the HVAC system within the prelimi- 1. the architect is a good project manager who designs nary budget allotment. Tools exist to pre-estimate build- buildings to meet the customer’s needs (rather than ing costs from historical data that can be adjusted for making an architectural statement), Figure 1.1 Plan-bid (a.k.a. owner-architect) building team structure. 2 Introduction to HVAC 2. who has hired competent engineers that provide tractors work for the design-build firm. Subcontractors thorough, code-compliant drawings and specifica- can also be outside firms. tions in a timely manner, This arrangement fosters better cooperation among 3. so that experienced and qualified contractors can participants, and the cost of change orders is the respon- provide sound bids. sibility of the design-build firm. However, the level of checks and balances among architects, engineers, and If the low bid is too high, the building is “value- contractors is reduced, which may leave the owner-cus- engineered,” or downsized, to meet the available budget. tomer more at risk. Savvy owners will likely hire a Great care and open communication are necessary to building professional to oversee the work of the design- make adjustments that do not alter the building’s build firm. intended functions and operating characteristics. Although the time schedule is often abbreviated, design drawings and specifications must be modified with THE ROLE OF AN HVAC ENGINEER thoughtful consideration being given to the impacts of Successful engineering firms consistently obtain lower-cost substitutions. The project is then re-bid and projects, which ensures steady income during normal initiated. However, costs may skyrocket due to many business cycles. These firms develop a positive reputa- noncompetitive bid “change orders” that are required tion by establishing trust with architects, construction because of the lack of time available to thoroughly rede- managers, and owners. Their reputation is enhanced by sign the building to meet the lower budget. a record of promptness, dependability, and completing Figure 1.2 is the design-build alternative to the projects on time and within budget. HVAC engineers in plan-bid method of designing and constructing build- such a firm do the following: ings. In this situation, the owner hires a design-build contractor who employs either in-house architects and 1. Establish relationships with other design team engineers or hires external firms. The project manager is members to maximize cooperation and coordination the design-build general contractor and all the subcon- of system integration and construction. Figure 1.2 Design-build organization chart. 3 HVAC Simplified 2. Review the plans and divide the building into zones. 10. Select related accessories. This includes, but is not Large zones are typically less expensive (the ther- limited to, humidification equipment, supplemental mostat is located in a central room; all other areas/ dehumidification equipment, ventilation air pre- rooms receive air, but comfort is dictated by condi- cooling/preheating equipment, vibration and noise tions where the thermostat is located). Having many mitigation systems, and seismic control precautions. smaller zones is more expensive because more indi- 11. Select control systems and specify operating vidual units (or terminals) and thermostats are sequences and control strategies. required. Balancing is more difficult, but comfort in 12. Redo steps 2 through 11 if bids are too high. most areas is better since there is more control. How much can the client afford? (Simple example: 13. Review testing and balancing (TAB) and/or com- Should you have one or two units for a two-story missioning reports to ensure systems are installed as house?) specified and operate as designed to best meet the owner’s needs, resulting in a safe, healthy, and com- 3. Calculate required heat loss and gain for each fortable working environment that operates in an zone. A lot of information is necessary regarding efficient and environmentally sustainable manner. thermal and moisture transmission properties of the structure, regional design criteria (temperature, The Challenge humidity levels, latitude, elevations, wind speed, etc.), the number of people occupying the building Building systems, code requirements, and design and their schedules, desired indoor conditions to procedures are becoming increasingly complex. Some maximize comfort and productivity, the lighting engineers may be tempted to default design responsibili- loads, the type of heat/moisture-generating equip- ties to equipment vendors (equipment selection, control ment in the building, the required amount of fresh specification, etc.) and contractors (ductwork quality, air or infiltration, and the proposed location of the piping material, etc.). This can place the customer at risk ductwork. In some cases this includes communicat- if the vendor chooses equipment that is more expensive ing with the architect or construction manager. than necessary, with proprietary components that cannot 4. Select the systems and related equipment to heat, be replaced or added with competitively priced alterna- ventilate, air-condition, and humidify or dehumidify tives. The engineer should do the design work rather each zone. This often involves decisions to either than allowing contractors to dictate specifications and (1) select higher-cost equipment that may use less procedures that can compromise the long-term quality energy and provide higher levels of comfort or (2) of the building. opt for less expensive equipment that is more eco- Good engineers must consider input from owners, nomically and environmentally costly and may not vendors, and contractors, but ultimately they must take provide good comfort or long service life. the time and effort to design the systems, prepare speci- 5. Select and locate the registers and diffusers to sup- fications, and verify that construction and operation ply and return air to each zone. A large number of practices follow design intent so that the building pro- low-velocity diffusers are quieter and generally pro- vides long-term occupant safety and health with the vide better comfort, but they cost more. Having lowest possible maintenance requirements, operating fewer registers requires high outlet velocities, costs, and environmental impact. which create more noise with less even air distribu- tion. The fan energy requirement is also increased. REFERENCES Large return air registers are more expensive, but they are quieter and have low friction losses. 1. NSPE. Engineers’ Creed. National Society of Profes- 6. Route and size ductwork to connect equipment to sional Engineers, Alexandria, VA. diffusers and registers. Large ducts cost more and 2. ASHRAE. 2004a. Code of Ethics. American Society are more difficult to route and install, but they gen- of Heating, Refrigerating and Air-Conditioning erate less noise (lower velocity) and require less fan Engineers, Atlanta. http://www.ashrae.org/tem- energy with resulting heat generation. plate/AssetDetail/assetid/25318. 7. Select and size fans. 3. ASHRAE. 2004b. ASHRAE Bylaws. American Soci- 8. Route and size piping for water-based systems. ety of Heating, Refrigerating and Air-Conditioning Large pipes cost more and are more difficult to Engineers, Atlanta. http://www.ashrae.org/tem- route and install, but they generate less noise (lower plate/AssetDetail/assetid/25318. velocity) and require less pump energy. 4. Means. 2004. Mechanical Cost Data. Kingston, MA: 9. Select and size pumps. R.S. Means, Reed Construction Data. 4 2 HVAC Fundamentals: Refrigeration REFRIGERATION CYCLES the surrounding fluid so it begins to flash in the evapora- tor. The cooling effect is accomplished in the evaporator The purpose of a refrigeration cycle is to transfer as the fluid (typically air or water) is passed through the heat from a region of low temperature to a region of higher temperature. There is a variety of thermodynamic opposite side of this heat exchanger and its temperature cycles for this purpose, but discussion in this section is is reduced by the evaporating refrigerant. The refriger- limited to the single-stage vapor compression cycle, ant exits the evaporator as low-temperature and low- which is the dominant method used in air-conditioning pressure gas to complete the cycle at point 1. (cooling-only) units and heat pumps (heating and cool- This process is referred to as the Carnot refrigera- ing). Introductory discussions of additional cycles can tion cycle. It is an attractive thermodynamic process be found in the ASHRAE Handbook—Fundamentals because heat is being “pumped” from one level (temper- (ASHRAE 2005, chapter 1). The heart of the cycle is the ature) to another. Therefore, it is possible to deliver heat compressor, which elevates the pressure and tempera- at a rate greater than the required input power. This pro- ture of a refrigerant gas. At the elevated temperature, vides an advantage over heating processes that convert heat is rejected to the environment (cooling mode) or to heat from chemical or electrical energy that cannot the indoor space (heating mode). After the refrigerant exceed conversion efficiencies of 100%. has released heat, it is a liquid at high pressure and flows to an expansion device. This device reduces the pressure The efficiency, or dimensionless coefficient of per- and temperature of the refrigerant, which initiates evap- formance (COP), of a Carnot refrigeration cycle is lim- oration of the liquid at low temperature. At this tempera- ited by the temperature of the heat source (evaporating ture, heat is removed from the indoor space (cooling temperature, te) and the heat sink (condensing tempera- mode) or the environment (heating mode). The refriger- ant gas then enters the compressor to repeat the cycle. The Carnot Cycle Figure 2.1 is a component diagram of a simple vapor compression refrigeration cycle operating in the cooling mode. Refrigerant enters the compressor as a low-temperature and low-pressure gas (point 1). Work (W) is required to drive the compressor so it can elevate the refrigerant to a higher pressure and temperature. The refrigerant exits the compressor as a superheated vapor (point 2) and enters the condenser. At the higher temper- ature, heat is rejected to the environment (air, water, or ground) through a condenser (heat exchanger), which lowers the temperature of the refrigerant vapor and then converts it to a liquid. The warm liquid refrigerant exits the condenser (point 3) and enters the expansion device. As the refrigerant leaves the expansion device (point 4), Figure 2.1 Simple vapor compression refrigeration its pressure is now lower than the saturation pressure of cycle. HVAC Simplified ture, tc). If cooling is the intended effect of the cycle, the The Ideal Vapor Compression Cycle theoretical COP is with Refrigerants t e ( in absolute temperature ) An important concept to remember in these systems -. COP c = ------------------------------------------------------------------ (2.1) is the relationship between the pressure of the refriger- tc – te ant and the saturation temperature at that point. The If the intended effect is heating (heat pump in heating high-pressure side of the system (compressor discharge mode), the theoretical COP is to expansion device inlet) operates at a relatively con- stant pressure. The corresponding saturation tempera- t c ( in absolute temperature ) -. COP h = ------------------------------------------------------------------ (2.2) ture (temperature at which a pure fluid changes state at a tc – te given pressure) is referred to as the condensing tempera- The COP values generated with Equations 2.1 and ture (tc). The refrigerant enters the condenser above this 2.2 assume the process is completely reversible. How- temperature, but once it has been desuperheated and ever, the second law of thermodynamics is used to begins to condense, the temperature will be essentially describe the irreversibilities in real processes due to constant until it is 100% liquid. In an ideal system the pressure drops, mechanical friction, heat transfer, etc., in refrigerant will exit the condenser at this state, but in a terms of entropy (Btu/lb·°F) flow. real system it is typically subcooled several degrees before entering the expansion device. On the low-pres- Thermodynamics Definitions sure side of the system (expansion device outlet to com- pressor inlet), the saturation temperature is referred to as adiabatic compression: compression of a gas during the evaporating temperature (te). The refrigerant typi- which no heat is exchanged with the surroundings. cally enters the evaporator at this temperature and adiabatic efficiency: (1) efficiency with which work is remains relatively constant until it is 100% vapor. In an done with respect to heat gains or losses. (2) (indicated ideal system the refrigerant will exit the evaporator at efficiency) ratio of the work absorbed in compressing a this condition, but in a real system it is typically super- unit mass of refrigerant in a compressor to the work heated several degrees before entering the compressor absorbed in compressing the same mass in an ideal com- inlet (suction). pressor. See isentropic process. An ideal system would include an isentropic com- adiabatic expansion: expansion of a fluid during which pression (s1 = s2) from point 1 to point 2, constant pres- no heat is exchanged with the surroundings. sure desuperheating and condensing (p2 = p3) to a saturated liquid state at point 3, adiabatic expansion adiabatic process: thermodynamic process during (h3 = h4) from a saturated liquid at p3 to a mixture of liq- which no heat is extracted from or added to the system. uid and gas at p4, and a constant pressure evaporation (p4 = p1) through the evaporator to point 1, a saturated enthalpy (heat content): thermodynamic quantity equal vapor at te. to the sum of the internal energy of a system plus the product of the pressure-volume work done on the sys- Both ideal and “real” vapor compression cycles can tem; h = u + pv, where h = enthalpy or total heat content, be analyzed with pressure-enthalpy (p-h) diagrams. In u = internal energy of the system, p = pressure, and this case, enthalpy (internal energy + pressure × vol- v = volume. ume) is used to quantify the energy of the refrigerant per unit mass at various points in the cycle. Figure 2.2 entropy (ratio): ratio of the heat absorbed by a sub- is a p-h diagram that is used to visualize the process stance to the absolute temperature at which it was thermodynamically (see note). More accurate and com- added. plete p-h diagrams are available in Appendix B, “Refrig- specific entropy: entropy per unit mass of a sub- erant Pressure-Enthalpy Charts and Sea Level stance. Psychrometric Chart,” Figures B.1 through B.3. irreversible process: a process in which available Tables 2.1 and 2.2 will also be used in this discussion to energy is lost due to the creation of entropy. The energy provide improved accuracy for the properties of R-134a. loss in a system or component is determined by multi- Table 2.1 lists the saturation temperatures, enthalpy, and plying the absolute temperature of the surroundings by entropy of saturated liquid and gaseous R-134a the entropy increase. (C2H2F4) for pressures of 25 psia through 225 psia. Sat- urated liquid values correspond to points on the left side isentrope: line of equal or constant entropy. of the dome-shaped curve in Figure 2.2. Saturated vapor isentropic process (reversible adiabatic): thermody- lines correspond to points on the right side of the dome- namic change at constant entropy. shaped line. Table 2.2 is a set of properties for super- 6 HVAC Fundamentals: Refrigeration Figure 2.2 R-134a pressure-enthalpy diagram for ideal vapor compression cycle. Table 2.1 Properties of Saturated R-134a Liquid and Vapor p (psia) 25 50 75 100 125 150 175 200 225 tsat (°F) 7.2 40.3 62.2 79.2 93.2 105.2 115.8 125.3 133.9 hliq (Btu/lb) 14.3 24.8 32.0 37.7 42.5 46.8 50.6 54.1 57.4 hvap (Btu/lb) 104.1 108.7 111.7 113.8 115.4 116.7 117.8 118.6 119.3 s-liq (Btu/lb·°F) 0.0322 0.0538 0.0678 0.0784 0.0872 0.0946 0.1013 0.1072 0.1127 s-vap (Btu/lb·°F) 0.2245 0.2217 0.2204 0.2196 0.2190 0.2184 0.2179 0.2174 0.2169 heated R-134a, which correspond to values to the right The process continues from point 1 via isentropic of the dome in Figure 2.2. compression to the condensing pressure of 200 psia along a line of constant entropy (s1 = s2 = Note: The reader is alerted to the use of h as the 0.2217 Btu/lb·°F) to point 2. Since this process moves symbol for heat transfer coefficient (in heat transfer), the refrigerant into the superheated vapor region, head (in fluid mechanics), and enthalpy (in thermody- Table 2.2 applies. To find point 2, it is necessary to namics). locate the point where p2 = 200 psia and s2 = Consider a vapor compression system operating at a 0.2217 Btu/lb·°F. Values in Table 2.2 indicate this occurs when t2 = 135°F. Thus, compressor inlet (suction) pressure of 50 lb/in.2 absolute (psia) (= 35.3 lb/in.2 gauge [psig]) and an outlet (dis- h2 = 121.4 Btu/lb, p2 = 200 psia, t2 ≈ 135°F charge) pressure of 200 psia (185.3 psig). The evaporat- (tsat@50 psia), ing temperature (te) at 50 psia pressure is 40.3°F and and point 1 is located on the saturated liquid line as shown in s2 = 0.222 Btu/lb·°F Figure 2.2. Table 2.1 is consulted for more exact values From point 2 the process continues along a line of at this point. p = 200 psia from a superheated vapor to a point on the saturated vapor line. At this point the temperature of the h1 = 108.7 Btu/lb, p1 = 50 psia, t1 = 40.3°F refrigerant is 125.3°F, the condensing temperature at (tsat@50 psia), 200 psia. The temperature and pressure remain constant and as the condensation process continues until the refriger- s1 = 0.2217 Btu/lb·°F ant is 100% liquid at point 3 on the saturated liquid line. 7 HVAC Simplified Table 2.2 Properties of Superheated R-134a Vapor P-abs. t-sat. P-gauge P-abs t-sat. P-gauge P-abs. t-sat. P-gauge 25 psia 7.2°F 10.3 psig 50 psia 40.3°F 35.3 psig 75 psia 62.2°F 60.3 psig t (°F) h (Btu/lb) s (Btu/lb·°F) t (°F) h (Btu/lb) s (Btu/lb·°F) t (°F) h (Btu/lb) s (Btu/lb·°F) 10 105.1 0.226 45 109.8 0.224 65 112.3 0.222 15 105.9 0.228 50 110.8 0.226 70 113.5 0.224 20 106.6 0.230 55 111.9 0.228 75 114.6 0.226 25 107.6 0.232 60 113.0 0.230 80 115.7 0.228 30 108.6 0.234 65 114.1 0.232 85 116.9 0.230 35 109.6 0.236 70 115.2 0.234 90 118.0 0.232 100 psia 79.2°F 85.3 psig 125 psia 93.2°F 35.3 psig 150 psia 105.2°F 135.3 psig t (°F) h (Btu/lb) s (Btu/lb·°F) t (°F) h (Btu/lb) s (Btu/lb·°F) t (°F) h (Btu/lb) s (Btu/lb·°F) 80 113.8 0.220 95 113.8 0.220 110 113.8 0.221 85 115.2 0.222 100 117.2 0.222 115 119.3 0.223 90 116.4 0.224 105 118.4 0.224 120 120.6 0.225 95 117.6 0.227 110 119.7 0.227 125 121.9 0.227 100 118.8 0.229 115 120.9 0.229 130 123.2 0.230 105 120.0 0.231 120 122.2 0.231 135 124.5 0.232 175 psia 115.8°F 160.3 psig 200 psia 125.3°F 185.3 psig 225 psia 133.9°F 210.3 psig t (°F) h (Btu/lb) s (Btu/lb·°F) t (°F) h (Btu/lb) s (Btu/lb·°F) t (°F) h (Btu/lb) s (Btu/lb·°F) 120 119.0 0.220 130 120.0 0.200 135 113.8 0.217 125 120.4 0.222 135 121.4 0.222 140 121.2 0.220 130 121.7 0.225 140 122.9 0.225 145 122.6 0.222 135 123.1 0.227 155 124.2 0.227 150 124.0 0.225 140 124.4 0.229 150 125.5 0.229 155 125.5 0.227 145 125.8 0.231 155 127.0 0.232 160 127.0 0.230 Note that the constant temperature line is horizontal Additionally, the minimum amount of power (wComp) within the dome-shaped curve created by the saturated required to compress the refrigerant and the maximum liquid and vapor lines. Table 2.1 can be consulted for the theoretical refrigerant cooling (COPc) can be found. For saturated liquid properties at 200 psia. any refrigerant flow rate (mr): h3 = 54.1 Btu/lb, p3 = 200 psia, t3 = 125.3°F qc = m r( h 1 – h4) (2.3a) (tsat@200 psia), w Comp = m r ( h 2 – h 1 ) (2.3b) and s3 = 0.1072 Btu/lb·°F qc ( h1 – h4 ) COP c = --------------- - = --------------------- (2.3c) From point 3 the process continues down a line of w Comp ( h2 – h1 ) constant enthalpy (h3 = h4) until it intersects a horizontal In cases where heating is the desired effect (i.e., line representing the evaporating pressure of 50 psia, heat pump heating mode, heat pump water heater), the which corresponds to a temperature of 40.3°F. At this desired effect is desuperheating and condensation point (4) the refrigerant is a mixture of liquid and vapor (h2h3). Equation 2.3 is modified to find qh, wComp, and (mostly liquid). COPh for heating. h4 = 54.1 Btu/lb, p4 = 50 psia, and t4 = 40.3°F qh = m r( h2 – h 3 ) (2.4a) (tsat@50 psia) w Comp = m r ( h 2 – h 1 ) (2.4b) The final leg of the process is constant pressure evaporation at p = 50 psia and t = 40.3°F from point 4 to q3 ( h2 – h3 ) point 1, which provides the desired refrigeration effect. COP h = --------------- - = --------------------- (2.4c) w Comp ( h2 – h1 ) The values generated in this process can be used to find the maximum possible cooling effect (qc) for R-134a at It should be noted that the values computed for the selected evaporating and condensing temperatures. COPc and COPh in Equations 2.3c and 2.4c are ideal- 8 HVAC Fundamentals: Refrigeration ized theoretical values for R-134a and should be less greater than ideal, and entropy will be generated than the corresponding theoretical values of since the processes are irreversible. Equations 2.1 and 2.2, which assume both an “ideal” There is pressure drop and internal heat generation refrigerant and reversible processes. Thus, the values through the condenser with a corresponding change computed using Equations 2.1 and 2.2 should be greater in saturation temperature as the pressure changes. than those found using Equation 2.3c and 2.4c for the Condensers are heat exchangers of finite size and same evaporating and condensing temperatures. will operate at temperatures elevated above the ide- alized condensing temperatures. Operating Characteristics of The refrigerant must be subcooled several degrees Real Vapor Compression Cycles below the saturation temperature to ensure the A familiarity with this process and the values gener- refrigerant is 100% liquid since the performance of ated in the preceding discussion is valuable even though many expansion devices is compromised by the the process is idealized. Limits are set and the system presence of vapor. designer can determine the relative importance of oper- Heat transfer and entropy generation are encoun- ating conditions and component selection that will have tered when refrigerant passes through real expan- the greatest impact on total system optimization. How- sion devices. ever, there are a number of constraints that limit the Evaporators are heat exchangers of finite size and capacity and efficiency of actual vapor compression will operate at temperatures below the idealized cycles. Several are listed here: evaporation temperatures. The evaporation temperature in many cases must be Refrigerant enters the compressor as a superheated suppressed to low values to meet the needs of the vapor to protect it from damage by liquid refriger- application (ice making, dehumidification, etc.). ant (it is essentially incompressible and may Many alternative refrigerants are zeotropic mixtures remove oil from bearing surfaces). Therefore, the that do not behave as a pure substance. Thus, con- inlet gas density will be slightly lower compared to densation and evaporation saturation temperatures saturated vapor, thereby reducing mass flow rate change (glide) with phase concentration. This typi- and compressor capacity. cally results in lower system efficiency and/or There are pressure drops (across ports, valves, and larger heat exchanger size. mufflers), friction in the compressor and fluid, and heat generated by the compressor drive. Thus, the Figure 2.3 is a p-h diagram that demonstrates the pressure difference from inlet to outlet will be impact of a number of these constraints. Figure 2.3 P-h process diagram of a real vapor compression cycle (ASHRAE 2005). 9 HVAC Simplified Figure 2.4 Hermetic compressors. Figure 2.5 Scroll compressor operation. Figure 2.6 Rotary vane compressor. 10 HVAC Fundamentals: Refrigeration COMPRESSORS Another type of compressor often used in a variety of applications is the rotary vane. One design is shown A variety of compressor types are available to meet in Figure 2.6. Larger compressors are typically “open” the many refrigeration and air-conditioning applications. drives in that they are connected through a shaft to a Several types are discussed in this section, but more motor (or engine). Figure 2.7 is a diagram of an open detail can be found in the ASHRAE Handbook—HVAC drive twin-screw compressor used for larger applica- Systems and Equipment (ASHRAE 2004a, chapter 34). tions. Figure 2.8 is a complete chiller package with a Figure 2.4 provides diagrams of two common hermetic centrifugal compressor that is typically used in large designs. The term hermetic indicates the motor and commercial and industrial applications. Performance compressor are encapsulated into a pressure vessel to data for these larger machines will be provided in chap- eliminate the compressor drive shaft leakage that occurs ter 5. with open compressor designs. The suction port opens The impact of operating characteristics on the actual performance of a compressor can be summarized directly into the casing, while the discharge port and in curves or tables. Table 2.3 is a typical presentation electrical leads enter through pressure-sealed passages. that includes the gross compressor refrigeration capac- Motor cooling and lubricating oil circulation are accom- ity, the required input power, and the refrigerant mass plished with strategic injection of the return gas. The flow rate for a hermetic scroll compressor with R-134a reciprocating piston design shown in Figure 2.4 places refrigerant. the motor above the compressor. The orbiting scroll Table 2.3 provides the performance information for design has become popular because of the reduction in various combinations of evaporating and condensing moving components. Advances in precision manufactur- temperatures at conditions that are typically encountered ing have resulted in scroll compressors with slightly in air-conditioning applications. The temperature of the higher efficiency compared to reciprocating models. vapor entering the compressor is 20°F (superheated) Figure 2.5 shows the scroll compression cycle. above the evaporating temperatures in the table. Com- Figure 2.7 Rotary twin-screw compressor. Figure 2.8 Chiller with centrifugal compressor. 11 HVAC Simplified Table 2.3 Performance of an R-134a Scroll Compressor (Copeland 2004a) Conditions: 20°F suction superheat, 15°F liquid subcooling, 95°F ambient air. Cond. Temp. Capacity (qr)—Power Input (kW)—Mass Flow Rate (mr)—Evaporation Temperature (°F) (°F) 10 20 30 40 45 50 55 qr - MBtu/h 16.0 20.3 25.6 31.9 35.5 39.4 43.7 80 kW 1.45 1.43 1.42 1.41 1.40 1.39 1.38 mr - lb/h 212 264 326 399 440 484 530 qr - MBtu/h 15.1 19.3 24.4 30.4 33.9 37.7 41.8 90 kW 1.63 1.62 1.60 1.58 1.57 1.57 1.56 mr - lb/h 210 263 325 398 439 483 530 qr - MBtu/h 14.3 18.3 23.1 29.0 32.3 35.9 39.8 100 kW 1.83 1.82 1.80 1.78 1.77 1.76 1.75 mr - lb/h 208 261 323 396 437 481 530 qr - MBtu/h 13.4 17.2 21.9 27.4 30.6 34.1 37.9 110 kW 2.07 2.05 2.03 2.01 2.00 1.98 1.97 mr - lb/h 206 259 322 395 436 480 525 qr - MBtu/h 12.5 16.1 20.6 25.9 28.9 32.2 35.8 120 kW 2.33 2.32 2.29 2.27 2.25 2.24 2.23 mr - lb/h 203 256 319 392 433 477 525 qr - MBtu/h --- 15.0 19.2 24.2 27.1* 30.3 33.7 130 kW --- 2.62 2.60 2.57 2.55* 2.54 2.52 mr - lb/h --- 253 316 389 430* 474 520 qr - MBtu/h --- --- 17.8 22.5 25.3 28.2 31.5 140 kW --- --- 2.94 2.91 2.89 2.88 2.86 mr - lb/h --- --- 311 385 426 470 515 * ARI-520 rating conditions: EER = 27.1 ÷ 2.55 = 10.6 COP = EER (MBtu/kWh) ÷ 3.412 MBtu/kWh. pressor manufacturers suggest this level of superheat to specific volume (νAct) to the specific volume for 20°F protect the compressor from any non-evaporated liquid superheated vapor (νSH = 20°F). entering the compressor. However, air-conditioner and ρ Ac t υ SH = 20°F heat pump manufacturers often attempt to reduce the q r ≈ q SH = 20°F ⎛ ------------------------⎞ ≈ q SH = 20°F ⎛ ------------------------⎞ (2.5a) superheat in order to increase the density (or lower the ⎝ ρ SH = 20°F⎠ ⎝ υ Ac t ⎠ specific volume) of the vapor entering the compressor. ρ Act υ SH = 20°F Higher density will result in higher mass flow rates and, m r ≈ m SH = 20°F ⎛ ------------------------⎞ ≈ m SH = 20°F ⎛ ------------------------⎞ (2.5b) therefore, greater cooling capacity with little change in ⎝ ρ SH = 20°F⎠ ⎝ υ Act ⎠ the power required. The performance rating conditions also consider that Refrigeration capacity values in Table 2.3 can be the liquid refrigerant leaving the condenser is subcooled corrected for other subcooling temperatures (tSC) by 15°F below the condensing temperature. This practice q r ≈ q SC = 15°F – m r c p ( 15°F – t SC ). (2.6) will add to the capacity of the system since the enthalpy of the liquid is reduced and will result in a lower The impact of alternative refrigerants is demon- enthalpy mixture entering the evaporator. A standard rat- strated by comparing the performance data shown in ing point (ARI 1997) for air-conditioning applications is Table 2.4, which result when the traditional air-condi- shown in bold in Table 2.3 with the figures of merit being tioning refrigerant (R-22) is substituted for R-134a in refrigeration capacity (qr) in Btu/h and energy efficiency the same compressor. Efficiency with R-22 improves by ratio (EER = qr/W Btu/Wh MBtu/kWh) at te = 45°F and approximately 5% and capacity increases by almost tc = 130°F. 50% at the standard rating point. This reduction in The refrigeration capacity (qr) and refrigerant mass capacity is almost directly proportional to the mass den- flow rate (mr) values in Table 2.3 can be corrected for sity of the refrigerant at the compressor suction. For this different suction superheat temperatures by assuming a reason, it is not likely that R-134a will be a popular R-22 direct correlation with the ratio of the rated to actual substitute in heat pump and air-conditioning applica- 12 HVAC Fundamentals: Refrigeration Table 2.4 Performance of an R-22 Scroll Compressor (Copeland 2004b) Conditions: 20°F suction superheat, 15°F liquid subcooling, 95°F ambient air. Cond. Temp. Capacity (qr)—Power Input (kW)—Mass Flow Rate (mr)—Evaporation Temperature (°F) (°F) 10 20 30 40 45 50 55 qr - MBtu/h 25.3 31.7 39.0 47.5 52.0 57.0 62.5 80 kW 1.99 1.98 1.97 1.95 1.94 1.94 1.93 mr - lb/h 315 391 475 570 625 680 740 qr - MBtu/h 24.2 30.3 37.4 45.5 50.0 55.0 60.0 90 kW 2.25 2.24 2.23 2.21 2.20 2.19 2.17 mr - lb/h 313 388 473 570 620 675 735 qr - MBtu/h 22.9 28.9 35.7 43.5 47.8 52.5 57.5 100 kW 2.55 2.54 2.53 2.50 2.49 2.48 2.46 mr - lb/h 309 384 468 565 615 670 730 qr - MBtu/h 21.6 2.73 33.8 41.3 45.5 50.0 54.5 110 kW 2.89 2.88 2.86 28.4 2.82 2.81 2.79 mr - lb/h 303 378 462 560 610 665 725 qr - MBtu/h 20.1 25.6 31.9 39.1 43.1 47.4 52.0 120 kW 3.27 3.26 3.25 3.22 3.20 3.18 3.16 mr - lb/h 295 370 455 550 605 660 720 qr - MBtu/h --- 23.8 29.2 36.8 40.6* 44.7 49.1 130 kW --- 3.69 3.68 3.65 3.63* 3.61 3.59 mr - lb/h --- 361 447 545 595* 650 710 qr - MBtu/h --- --- 27.8 34.4 38.1 42.0 46.2 140 kW --- --- 4.16 4.13 4.12 4.09 4.07 mr - lb/h --- --- 437 535 585 640 700 * ARI-520 rating conditions: EER = 40.6 ÷ 3.63 = 11.2. tions. More likely substitutes are R-407c and R-410a, during a 24-hour period. The heat of fusion for water-to- which provide improved capacities and in some cases ice is 144 Btu/lb; thus, higher efficiencies for compressors of equivalent size. However, they will operate at higher pressures. q r ⎛ -------------⎞ = 2000 Btu/h ( lb/ton ) × 144 ( Btu/lb ) ------------------------------------------------------------------ ⎝ ton ⎠ 24 h (2.8) In the US the COP of compressors traditionally has = 12, 000 Btu/ton·h not been expressed in terms of the dimensionless param- eter COP. Manufacturers have used the energy effi- To convert kW/ton to EER and COP, ciency ratio (EER) with the units of capacity in Btu/h and power input in watts. Coincidentally, the value for 12000 ( Btu/ton·h ) - = ------------------------ EER ( Btu/W·h ) = -------------------------------------------------------------- 12 - EER in Btu/Wh is identical to the value if the units of 1000 ( w/kW ) × ( kW ⁄ ton ) ( kW ⁄ ton ) capacity are expressed in the often used units of MBtu/h (2.9) (1000 Btu/h) and power in kW. (Note the English unit symbol for 1000 is M while the international unit sym- 12000 ( Btu/ton-h ) COP = -------------------------------------------------------------------------------------------------------------- 1000 ( W/kW ) × 3.412 ( Btu/W·h ) × ( kW ⁄ ton ) bol for 1000 is k.) 3.52 To convert EER to COP, = ------------------------- ( kW ⁄ ton ) (2.10) EER ( Btu/W·h ) EER ( MBtu/kWh ) COP = --------------------------------------- = ---------------------------------------------- (2.7) 3.412 ( Btu/W·h ) 3.412 ( MBtu/kWh ) REFRIGERANT CONTROLS Manufacturers of larger compressors used to pro- A variety of control devices are used to optimize the duce chilled water (chillers) have traditionally expressed performance and protect the components of refrigerant performance in terms of an inverse efficiency: kW of devices. A few simple devices are discussed here, but input power per ton of refrigeration (kW/ton), where a more detail and a list of references are presented in the ton is defined as the rate (12,000 Btu/h) required to con- ASHRAE Handbook—Refrigeration (ASHRAE 2002, vert 2000 lb (1 ton) of liquid water at 32°F to ice at 32°F chapter 45). 13 HVAC Simplified The metering of refrigerant flow is critical to ensure permits flow to go in both directions. This is necessary efficient performance without risking damage to the for heat pump operation when flow is reversed. compressor under widely varying loads. A common The capillary tube shown in Figure 2.10 is another metering device is the thermostatic expansion valve refrigerant flow metering device that is often used in (TXV), shown in Figure 2.9. The valve is located refrigerators, freezers, small air-conditioning units, and between the condenser and the evaporator. It is opened air heat pumps operating in the heating mode. The and closed by a diaphragm, which is connected by a length and diameter of the “cap tube” are sized to pro- small capillary tube to a bulb filled with an ideal gas vide a relatively constant mass flow rate at all condi- (typically some type of refrigerant). The bulb is attached tions. The tube is sized to pass primarily liquid. Higher with insulating wrap to the refrigerant line between the flow rates are regulated since the refrigerant will tend to evaporator outlet and the compressor inlet. Consider the flash as back pressure is reduced, thus creating a higher situation with the valve partially open. As the load volume flow rate and more resistance to increased flow. increases, more heat is available, so the gas leaving the Short-tube (3/8 to 1/2 in.) restrictors operate in the evaporator will have an increasing temperature (super- same fashion as capillary tubes, but they are more com- heat). The temperature in the bulb will also increase. pact and can be replaced more easily. They can also be Since the gas is ideal, the pressure will also increase, used to eliminate the check valves in heat pump systems which expands the diaphragm and opens the valve to when two different metering devices are needed because increase refrigerant flow. The temperature of the gas cooling and heating mode mass flow rates are substan- leaving the evaporator will then decrease since the mass tially different. In the forward flow direction, the body flow has increased. If the valve is correctly sized, the of the restrictor opens like a check valve. In the reverse correct valve position will be established without a great direction, flow is forced through the small restrictor port deal of oscillation (or “hunting”). that serves as the expansion device, as shown in Note the valve has a screw to set the superheat tem- Figure 2.10. A second restrictor (not shown in perature by adjusting the spring tension on the dia- Figure 2.10) is open in the reverse flow direction and is phragm. There are two options for the valve shown. One the expansion device in the forward direction. is a “bleed port” design that permits a small amount of Refrigeration control can also be accomplished refrigerant to flow at all times, which permits the high- with electric or electronically activated valves. One of pressure side to bleed to the low-pressure side of the the most common control valves is the four-way revers- system more quickly when the compressor turns off. ing valve, which is used to reverse the mode of a heat This speeds the time that the compressor is able to pump from heating to cooling operation. Most heat restart without having to work against a high differential pump designs set the U-shaped port to connect the out- pressure. The second variation is a bi-flow valve, which door coil to the compressor suction, which also allows Figure 2.9 Thermostatic expansion valve (TXV). 14 HVAC Fundamentals: Refrigeration Figure 2.10 Capillary tube and short-tube restrictor expansion devices. Figure 2.11 Heat pump four-way reversing valve. flow from the compressor discharge to the indoor (heat- including performance, application operating conditions, ing) coil, as shown in the “Heating Mode” position of environment, safety, lubricant compatibility, and materi- Figure 2.11. Thus, the hot gas from the compressor goes als of construction compatibility. In the early develop- to the indoor coil, which is the condenser in this mode. ment of refrigeration, flammable and hazardous fluids The refrigerant flows from the indoor coil through the were common. More stable and inert refrigerants were expansion device into the outdoor coil, which is the developed by substituting fluorine and chlorine for some evaporator in this mode. When the coil is activated elec- or all of the hydrogen in light hydrocarbons (methane, trically, the U-shaped port is set so that gas flows from ethane, propane). Chlorofluorocarbons (CFCs) were the indoor coil to the compressor suction. Hot compres- widely used for low-temperature refrigeration, mobile air sor gas is routed to the outdoor coil, which is the con- conditioning, and centrifugal chillers. Hydrochlorofluo- denser in the cooling mode. Refrigerant moves through rocarbons (HCFCs) were commonly used in air-condi- the expansion device through the indoor coil, which is tioning, heat pumps, and screw compressors. Azeotropic now the evaporator (cooling coil). Except for the revers- blends (mixtures that behave similarly to pure refriger- ing valve, heat pump operation is identical in this mode ants) of CFCs and HCFCs were also common. to a conventional air conditioner. The chemical stability and low cost of CFCs that made them attractive refrigerants have resulted in unin- REFRIGERANTS tended environmental consequences. For many years, Refrigerants for vapor compression systems are a CFCs from systems that needed repair were frequently rapidly evolving topic due to a multitude of constraints vented to the atmosphere. Systems with minor leaks 15 HVAC Simplified Refrigerant Charging The amount of refrigerant charge in a vapor compression system is critical to maintain efficient performance and minimize maintenance requirements. The system must also be free of noncondensable vapor (such as air) and water. Factory-charged systems and systems that have been repaired must be completely evacuated with a vacuum pump to remove all contaminants. The system is then charged with refrigerant, with great care being required to not re-inject air into the system. A highly recommended method of metering the correct charge into new, repaired, or poorly functioning systems is by “suction superheat.” Manufacturers will provide a recommended suction superheat (typically 10°F to 20°F). A refrigerant-charging manifold with two gauges is connected to the compressor suction and discharge. A small bulb thermometer is attached to the outside of the suction line, as shown in the figure below. Refrigerant is drawn into the suction operating compressor through the manifold. The low-pressure gauge records the suction pressure (in psig = psia –14.7) shown in the enlarged view on the left. Note the gauge has two scales that also pro- vide the saturation temperature of R-134a and R-22. The gauge indicates the suction pressure is 40 psig, which corresponds to an evaporation temperature (te) of 45°F. However, the thermometer indicates the temperature is actually 60°F, which means the superheat is 15°F (60°F – 45°F). Note also the discharge pressure is 250 psig (tc = 144°F), which is somewhat high for an R-134a system. Figure 2.12 Determination of acceptable refrigerant charge using suction superheat. 16 HVAC Fundamentals: Refrigeration were recharged rather than repaired. CFCs were used for provides higher capacity but greater power consump- cleaning and insulation manufacturing. These stable tion. This typically results in high compression ratios, compounds diffused into the atmosphere where condi- greater loads on the compressor, shortened compressor tions allow them to break down. The released chlorine life, and higher required rating pressure for heat then reacts with and begins to deplete the atmosphere’s exchangers and related components (Hughes 2003). protective ozone layer. HCFCs also contribute some- There are a variety of potential replacements for R-22 what to ozone depletion, but they are less stable and applications that demonstrate a variety of benefits and tend to break down before reaching the stratosphere. limitations. The Montreal Protocol, originally signed in 1987 and Azeotropic refrigerant mixtures exhibit characteris- continuously amended, is an international agreement to tics similar to pure substances in that they evaporate and phase out the production of CFCs by 1996 and essen- condense at a constant temperature when pressure is tially phase out HCFCs by 2020 (UNEP 2003). held constant. However, zeotropic mixtures do not Currently a variety of hydrofluorocarbons (HFCs) exhibit constant temperature during evaporation and and mixtures of HCFCs and hydrocarbons are being condensation. This undesirable trait (glide) results in a used as replacement refrigerants. There are advantages reduction in efficiency unless heat exchangers are and disadvantages to all options that cannot be ade- enlarged to compensate. A more practical problem quately addressed in this document. References are results from the fact that they also tend to have different available for additional information (ASHRAE 2005, component fractions in the gas phase at various temper- chapters 19, 20; ASHRAE 2002, chapter 5; McLindon atures. When leaks occur, the individual component et al. 2000). However, some general characteristics are losses are not in proportion to the original refrigerant listed in the following sections. components. Recharging is complicated because the makeup of the remaining refrigerant is not easily deter- Refrigerant Numbering System mined. For methane, ethane, and propane derivatives, the refrigerant number is related to the number of Safety and Environmental Issues atoms in the compound structure by ASHRAE Standard 34 (ASHRAE 2004b) classifies R- [carbon atoms −1][hydrogen atoms +1] refrigerants into six safety groups (A1, A2, A3, B1, B2, [fluorine atoms]. B3). Letter designations refer to toxicity, with class A being no identifiable toxicity and B being toxic. Numer- For example, ical values indicate flammability, with 1 being no flame chlorodifluoromethane (CHClF2) is propagation in standard air, 2 having a low, lower flam- R- [1−1][1+1] = R-022 or R-22. mability limit (LFL) and low heat of combustion ( 0.88]), lenge of high ventilation air loads. In some cases this such as air-cooled designs. Table 5.8 summarizes the involves the use of a dedicated outdoor air system requirements for minimum COP for chillers from (DOAS) that is decoupled from the primary cooling and ASHRAE Standard 90.1-2004. These values are con- Table 5.8 ASHRAE Standard 90.1 Minimum Chiller COP, EER, and Maximum kW/ton (ASHRAE 2004a) Conditions according to ARI Standard 550 (ARI 1998d): 44°F LWT, 85°F/95°F Condenser Water or 95°F OAT < 150 tons (530 kW) ≥ 150, < 300 tons ≥ 300 tons (1060 kW) Chiller Type COP kW/ton EER COP kW/ton EER COP kW/ton EER Air-Cooled w/Condenser 2.8 1.26 9.6 2.8 1.26 9.6 2.8 1.26 9.6 Air-Cooled w/o Condenser 3.1 1.14 10.6 3.1 1.14 10.6 3.1 1.14 10.6 Water Cooled Reciprocating 4.2 0.84 14.3 4.2 0.84 14.3 4.2 0.84 14.3 Water Cooled Scroll and Screw 4.45 0.79 15.2 4.9 0.72 16.7 5.5 0.64 18.8 Water Cooled Centrifugal 5.0 0.70 17.1 5.55 0.63 18.9 6.1 0.58 20.8 Figure 5.12 Central air handler with variable air volume and fan-powered terminals. 55 HVAC Simplified verted to EER and maximum allowable kW/ton, an three chiller leaving water temperatures (LWTs). inverse efficiency commonly used by manufacturers in Figure 5.13 provides information regarding the evapora- the US. The spreadsheet program HVACSysEff.xls is tor head loss as a function of water flow rate for the five available on the CD accompanying this book to compare chiller models shown in Table 5.9. As noted, the values the system COP, kW/ton, and EER of all HVAC types in Table 5.9 correspond to a drop in chilled water tem- when all components are included. perature of 10°F, which results from a chilled flow rate Table 5.9 provides the capacity (ton), demand of 2.4 gpm/ton. Therefore, nominal evaporator flow rate (kW), and efficiency (EERBtu/W·h) of a product line of can be approximated by multiplying 2.4 by the chiller small air-cooled chillers with scroll compressors. Data capacity in tons. A typical arrangement for an air-cooled are given for three outdoor air temperatures (OATs) and chiller is shown is Figure 5.14. Table 5.10 provides the capacity (tons), demand (kW), and efficiency (EER ≡ Btu/W·h) of a product line of small water-cooled chillers with scroll compressors. Data are given for three condenser entering water tem- peratures (EWTs) and three chiller leaving water tem- peratures (LWTs). Figure 5.13 can be used to determine the evaporator head loss for the air-cooled chiller prod- uct line. Figure 5.15 provides information regarding the condenser head loss as a function of water flow rate for the water-cooled chillers. As noted, the values in Table 5.10 correspond to a drop in chilled water temper- ature of 10°F and a rise in condenser water of 10°F. This results from a chilled flow rate of 2.4 gpm/ton and a condenser water flow rate of 3.0 gpm/ton. Therefore, nominal evaporator flow rate can be approximated by multiplying 2.4 by the chiller capacity in tons, and con- Figure 5.13 Evaporator head loss for Table 5.9 (AC) denser flow rate can be approximated by multiplying 3.0 and Table 5.10 (WC) chillers. by the chiller capacity in tons. Table 5.9 Air-Cooled Chiller Capacity,* Demand,* and Efficiency (T/ASC 2002) LWT OAT = 75°F OAT = 95°F OAT = 115°F °F tons kW EER† tons kW EER tons kW EER 40 18.0 17.5 12.4 16.2 21.3 9.2 14.2 26.1 6.5 Model 45 19.7 18.0 13.2 17.8 21.8 9.8 15.6 26.7 7.0 020 50 21.5 18.4 14.0 19.4 22.3 10.4 17.1 27.3 7.5 40 28.5 28.1 12.2 25.7 33.9 9.2 22.7 40.8 6.7 Model 45 31.2 28.6 13.1 28.2 34.3 9.8 24.9 41.6 7.2 030 50 34.0 29.2 14.0 30.8 35.0 10.5 26.3 42.1 7.5 40 35.3 34.1 12.4 31.7 41.5 9.2 27.8 51.0 6.5 Model 45 38.5 35.0 13.2 34.7 42.5 9.8 30.5 52.1 7.0 040 50 42.0 35.9 14.0 37.8 43.5 10.4 33.3 53.2 7.5 40 44.1 43.3 12.2 39.7 51.9 9.2 35.0 63.0 6.7 Model 45 48.3 44.3 13.1 43.5 53.1 9.8 38.5 64.3 7.2 050 50 52.7 45.3 14.0 47.5 54.3 10.5 42.1 65.7 7.7 40 58.5 56.6 12.4 52.6 68.5 9.2 46.2 83.4 6.7 Model 45 63.7 58.1 13.2 57.3 70.2 9.8 50.6 85.4 7.1 060 50 69.1 59.6 13.9 62.3 72.0 10.4 55.0 87.5 7.5 † EER (Btu/W·h) = 12 × TC (tons) ÷ W (kW) = 12,000 × TC (tons) ÷ W (watts) gpm (Evap.) ≈ TC (Btu/h) ÷ [500 × Δtevap (°F)] * Performance based on 100% water and 10°F chilled water temperature drop. For 30% ethylene glycol: 70% water, multiply capacity (tons) by 0.97 and kW by 0.985. For 30% propylene glycol: 70% water, multiply capacity (tons) by 0.955 and kW by 0.98. 56 HVAC Equipment, Systems, and Selection Figure 5.14 Air-cooled chiller. Table 5.10 Water-Cooled Scroll Compressor Chiller Capacity,* Demand,* and Efficiency (T/ASC 1999) Condenser Condenser Condenser LWT EWT = 75°F EWT = 85°F EWT = 95°F °F tons kW EER† tons kW EER tons kW EER 40 19.2 13.8 16.7 18.6 15.4 14.6 17.7 17.1 12.4 Model 45 21.1 13.9 17.9 20.4 15.4 14.9 19.5 17.2 13.4 020 50 23.2 14.1 19.7 22.4 15.6 17.2 21.4 17.4 14.8 40 28.3 20.7 16.4 27.0 22.9 14.1 25.6 25.4 12.1 Model 45 30.9 20.8 17.9 29.7 23.0 15.5 28.2 25.6 13.2 030 50 34.1 21.1 19.5 32.6