공생 기말 총 PDF
Document Details
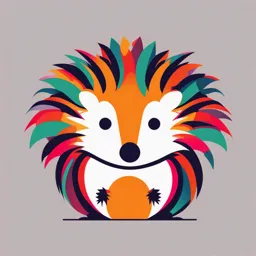
Uploaded by BestSellingDravite
Tags
Related
- Bios 138 Cell Biology and Respiration 2 PDF
- ATP: The Energy Currency of Cells PDF
- Experiment 5: Cellular Aerobic Respiration (Biology)
- 5 – Regulating Cellular Respiration and Other Metabolic Pathways - MHR Grade 12 Biology
- Cellular Respiration and Fermentation (Biology 10, PDF)
- Cellular Respiration and Fermentation PDF
Summary
이 문서는 생물학의 세포 신호전달, 세포 호흡, 그리고 아폽토시스를 다루는 기말고사 자료입니다. 세포 호흡 과정, 세포 신호 전달 경로, 그리고 아폽토시스에 대한 개요, 원리, 및 관련 사례를 다룹니다.
Full Transcript
작년 기출 문제 표시 Chapter 9.3: Transduction Usually involves multiple steps. Can greatly amplify signals. 1. Signal transduction pathways Binding of signaling molecule to the receptor triggers the first step. Receptor activates another protein. At each step, the signal is transduce...
작년 기출 문제 표시 Chapter 9.3: Transduction Usually involves multiple steps. Can greatly amplify signals. 1. Signal transduction pathways Binding of signaling molecule to the receptor triggers the first step. Receptor activates another protein. At each step, the signal is transduced into different forms: usually the shape of the protein changes. 2. Protein Phosphorylation and Dephosphorylation Protein Kinases transfer phosphates from ATP to protein ← Phosphorylation Many relay molecules in signal transduction pathways are protein kinases → create phosphorylation cascade Protein Phosphatases remove phosphate from proteins ← Dephosphorylation These two systems act as a molecular switch. 3. Small molecules and ions as second messengers Second messengers: small, nonprotein, water-soluble molecules or ions that spread throughout the cell by diffusion. Participate in pathways initiated by GPCRs and RTKs. e.g. Cyclic AMP and calcium ions. - Cyclic AMP (cAMP) Adenylyl cyclase: enzyme in plasma membrane. Converts ATP to cAMP in response to extracellular signals. Other components of cAMP pathways are G proteins, GPCRs, and protein kinases. cAMP usually activates protein kinase A, which phosphorylates other proteins. Further regulation of cell metabolism is provided by G-protein systems that inhibit the adenylyl cyclase. Importance of understanding signaling transduction pathways and cAMP - Vibrio cholerae produces a toxin that modifies a G protein → stuck in active form. Continuously makes cAMP. Intestinal cells secrete large amounts of salt into the intestines. Due to osmosis, an untreated person dies from loss of water and salt. - Cyclic GMP (cGMP), in one pathway, acts as a signaling molecule whose effects include relaxation of smooth muscles in the artery walls. Compound inhibiting hydrolysis of cGMP to GMP was prescribed for chest pains → Viagra, now used as treatment for erectile dysfunction. - Calcium ions (Ca2+) and Inositol Triphosphate (IP3) Calcium ions can function as a second messenger because its concentration in the cytosol is much lower than its concentration outside of the cell. Increasing cytosolic concentration of Ca2+ causes muscle cell contraction, secretion, cell division. Pathways leading to release of calcium involve inositol triphosphate (IP3) and diacylglycerol (DAG), which is produced by cleavage of certain phospholipid in the plasma membrane Chapter 9.4 Response 1. Nuclear and cytoplasmic responses Responses may occur in cytoplasm or in the nucleus. Final activated molecule may act as a transcription factor. Other pathways regulate activity of enzymes rather than synthesis e.g.opening ion channel Signaling pathways con affect overall cell behavior e.g.signal could lead to cell division 2. Regulation of response a. Amplification Enzyme cascades amplify response. Number of activated products is much greater at each step. b. Specificity of response Different proteins allow cells to detect and respond to different signals. Same signals can have different effects in different cells. c. Efficiency of response Scaffolding proteins: large relay proteins to which other relay proteins are attached. Can increase efficiency by grouping different proteins involved in the same pathway. d. Termination of signal If ligand concentration falls, fewer receptors will be bound. Unbound receptors revert to inactive state. G proteins function as GTPase: hydrolyzes bound GTP to GDP. Chapter 9.5 Apoptosis “Programmed cell death” Components of infected, damaged cells or cells that reached the end of functional lives are chopped and packaged into vesicles and digested by scavenger cells. Prevents enzymes from leaking out of a dying cell and damaging neighboring cells. Apoptosis in Caenorhabditis elegans (soil worm) Signals activate a cascade of suicide proteins in cells programmed to die. When a death signal is received, Ced-9, an apoptosis inhibiting protein, is inactivated. Chief caspase in nematodes is called Ced-3. In humans and other mammals, several pathways, including 15 caspases, can carry out apoptosis. One pathway involves mitochondrial proteins that form molecular pores in the mitochondrial outer membrane, causing it to release apoptosis promoting proteins, including cytochrome C, which functions in mitochondrial electron transport but acts as cell death factor when released. Apoptosis can be triggered by signals outside or inside the cell. Internal signals can result from irreparable DNA damage or excessive protein misfolding. Apoptosis is involved in hands and feet formation. External signals: death signaling ligands. Chapter 10. Cell respiration Living cells require energy from outside sources. a. Energy flows into the ecosystem as sunlight and leaves as heat. b. Photosynthesis generates O2 and organic molecules. c. Cells use chemical energy stored in organic molecules to generate ATP. Chapter 10.1 Catabolic pathways Catabolic pathways release stored energy by breaking down complex molecules. Electron transfer is important in these pathways. 1. Catabolic pathways and production of ATP Breakdown of organic molecules is exergonic. Fermentation: partial degradation of sugars that occurs without O2. Aerobic respiration: consumes organic molecules and O2, yields ATP. Anaerobic respiration is similar to aerobic respiration but consumes compounds other than O2. Cellular respiration: includes both aerobic and anaerobic; usually refers to aerobic. 2. Redox reactions: Oxidation and Reduction Transfer of electrons releases energy stored in organic molecules. This energy is used to synthesize ATP. a. Redox Chemical reactions that transfer electrons between reactants are called redox reactions. Oxidation: a substance loses electrons, oxidized. Reduction: a substance gains electrons, reduced. Reducing agent: electron donor. Oxidizing agent: electron receptor. Some redox reactions do not transfer electrons but change electron sharing in covalent bonds. b. Oxidation of organic fuel molecules during cellular respiration. Fuel(e.g. glucose) is oxidized, O2 is reduced. c. Stepwise energy harvest via NAD+ and Electrons transport chain Electrons from organic compounds are usually first transferred to a coenzyme NAD+. NAD+ functions as an oxidizing agent during cellular respiration. Each NADH(reduced form of NAD+) represents stored energy tapped to synthesize ATP. NADH passes electrons to the electron transport chain. O2 pulls electrons down the chain in an energy-yielding tumble. Energy yielded is used to regenerate ATP. 3. Stages of Cellular Respiration Glycolysis; breaks down glucose in 2 pyruvate molecules. Citric acid cycle: completes glucose breakdown Oxidative phosphorylation: most (90%) of the ATP synthesis as it is powered by redox reactions. Smaller amount of ATP formed in glycolysis and citric acid cycle by substrate-level phosphorylation. For each glucose molecule, a cell makes up to 32 ATP molecules. Chapter 10.2 Glycolysis Harvests chemical energy by oxidizing glucose to pyruvate. Has 2 major phases: energy investment phase, energy payoff phase. Chapter 10.3 Citric acid cycle In presence of O2, pyruvate enters the mitochondrion, where oxidation of glucose is completed. 1. Oxidation of pyruvate to Acetyl CoA Pyruvate must be converted to acetyl Coenzyme A, which links glycolysis to citric acid cycle. This step is carried out by a multienzyme complex that catalyzes 3 reactions. 2. Citric acid cycle (Krebs cycle) The citric acid cycle completed the breakdown of pyruvate to CO2. The cycle oxidizes organic fuel derived from pyruvate, generating 1 ATP, 2 NADH, and 1 FADH2 per turn. The cycle has 8 steps, each catalyzed by a specific enzyme. The acetyl group of acetyl CoA joins the cycle by combining with oxaloacetate, forming citrate. Next 7 steps decompose citrate back to oxaloacetate, making the process a cycle. NADH and FADH2 produced by the cycle relay electrons extracted from food to the electron transport chain. Chapter 10.4 Oxidative phosphorylation NADH and FADH2 donate electrons to the electron transport chain, which powers ATP synthesis via oxidative phosphorylation. 1. Pathway of Electron transport Electron transport chain is in the inner membrane(cristae) of the mitochondrion. Most of the components are proteins. Carriers alternate reduced and oxidized states as they accept/donate electrons. Electrons are passed through a number of proteins including cytochromes to O2. The electron transport chain generates no ATP directly. 2. Chemiosmosis: Energy coupling Electron transfer in the chain causes proteins to pump H+ from the mitochondrial matrix to the intermembrane space. H+ moves back across the membrane, passing through the protein complex, ATP synthase. ATP synthase uses exergonic flow of H+ to drive phosphorylation of ATP. Energy stored in a H+ gradient across the membrane couples the redox reactions of the electron transport chain to ATP synthesis. H+ gradient is called proton-motive force 3. ATP production by cellular respiration Glucose → NADH → electron transport chain → proton-motive force → ATP 34% of energy in glucose molecules is transferred to ATP, yielding about 32 ATP. Number of ATP is not known exactly because phosphorylation and redox reactions are not directly coupled, so the ratio of NADH to ATP is not a whole number. Chapter 10.5 Fermentation and anaerobic respiration Without O2, the electron transport chain does not operate. Glycolysis couples with anaerobic respiration or fermentation to produce ATP. Anaerobic respiration uses an electron transport chain with a final receptor other than O2. e.g. sulfate. Fermentation uses substrate level phosphorylation instead of electron transport chain. 1. Types of fermentation Fermentation consists of glycolysis plus reactions that regenerate NAD+, which can be reused by glycolysis. Two common types: alcohol fermentation and lactic acid fermentation. Lactic acid fermentation Pyruvate is reduced by NADH, forming lactate with no release of CO2. Human muscle cells use lactic acid fermentation to generate ATP when O2 is scarce. 2. Fermentation vs Aerobic/Anaerobic respiration All use glycolysis (net ATP = 2). In all three, NAD+ is the oxidizing agent. In fermentation, an organic molecule acts as the final receptor. In cellular respiration, electrons are transferred to the electron transport chain. Cellular respiration produces about 32 ATP; fermentation produces 2 ATP. Obligate anaerobes carry out fermentation or anaerobic respiration and cannot survive in presence of O2. Yeast and many bacteria are facultative anaerobes: they can survive using either fermentation or cellular respiration. In a facultative anaerobe, pyruvate is a fork in the metabolic road that leads to 2 alternative catabolic routes. 3. Evolutionary significance of glycolysis Ancient prokaryotes may have used glycolysis long before there was oxygen. They likely used only glycolysis to generate ATP. Chapter 10.6 Glycolysis and citric acid cycle connect to other Metabolic pathways 1. Versatility of Catabolism Glycolysis accepts a wide range of carbohydrates. Proteins must be digested to amino acids; amino groups can feed glycolysis or citric acid cycle. Fats are digested to glycerol and fatty acids. Fatty acids are broken down by beta oxidation and yield acetyl CoA. Oxidized gram of fat produces more than double the ATP as an oxidized gram of carbohydrate. 2. Biosynthesis (Anabolic pathways) Body uses small molecules to build substances. Feedback inhibition or metabolic control. If ATP concentration begins to drop, respiration speeds up and vice versa. Regulate activity of enzymes. Phosphofructokinase, which catalyzes an early step in glycolysis, is stimulated by AMP and inhibited by ATP and citrate. Chapter 11. Photosynthesis Converts solar energy into chemical energy. Autotrophs are producers of the biosphere. Heterotrophs are consumers of the biosphere. Fossil fuels contain high percentages of carbon. e.g. coal, petroleum, natural gas. Chapter 11.1 Photosynthesis converts light energy to chemical energy 1. Chloroplasts ↑ Chloroplasts are found mainly in cells of mesophyll, interior tissue of the leaf. Each mesophyll cell has 30-40 chloroplasts. CO2 enters and O2 exits the leaf through stomata. Chloroplast has an envelope of 2 membranes surrounding a dense fluid called stroma. Chloroplasts are structurally similar to and likely evolved from photosynthetic bacteria. Thylakoids: connected sacs in chloroplast composing a third membrane system. May be stacked in columns called grana. Chlorophyll: pigment which gives leaves their green color, resides in thylakoid membranes. 2. Tracking atoms through photosynthesis Reverse of cellular respiration. Chloroplasts splits H2O into H and O, incorporating electrons of H into sugar and releasing oxygen. Redox process where H2O is oxidized and CO2 is reduced. Photosynthesis is an endergonic process (energy is absorbed). 3. 2 stages of photosynthesis Light reactions and Calvin cycle Light reactions in thylakoids splits H2O, release O2, reduce electron acceptor NADP to NADPH, and generate ATP from ADP by photophosphorylation. The Calvin cycle in stroma forms sugar from CO2 using ATP and NADPH. Begins with carbon fixation, incorporating CO2 into organic molecules. Chapter 11.2 Light reactions 1. Nature of sunlight Light is electromagnetic energy/radiation. Wavelength is the distance between crests of waves and determines the type of electromagnetic energy. Electromagnetic spectrum is the entire range of radiation. Visible light: wavelengths that produce colors we can see. Light behaves as though it consists of discrete particles: photons 2. Photosynthetic pigments: Pigments: substances that absorb visible light. Wavelengths that are not absorbed are reflected or transmitted. Chlorophyll reflects green light. A spectrophotometer measures a pigment’s ability to absorb various wavelengths. Absorption spectrum is a graph plotting a pigment’s light absorption vs wavelength. Action spectrum plots the relative effectiveness of different wavelengths of radiation in driving a process. Violet-blue and red most effect Chlorophyll a is the main photosynthetic pigment. Accessory pigments such as chlorophyll b broaden the spectrum used for photosynthesis. Difference in absorption spectrum between pigments is due to slight structural difference. Accessory pigments called carotenoids function in photoprotection: absorb excessive light that can damage the chlorophyll. 3. Excitation of chlorophyll by light When pigment absorbs light, it goes from ground state to excited state, which is unstable. When excited electrons fall back to ground state, photons are emitted → fluorescence. 4. Photosystem A photosystem consists of a reaction-center complex surrounded by light-harvesting complexes. Light-harvesting complexes (pigments bound to proteins) transfer energy of photons to the reaction center. A primary electron acceptor in the reaction center accepts excited electrons and is reduced. 2 types of photosystems in thylakoid membrane: 1. Photosystem II (PS II) functions first. Best at absorbing wavelengths of 680 nm. Reaction center is called P680. 2. Photosystem I (PS I)is best at absorbing wavelengths of 700 nm. Reaction center is called P700. 5. Linear Electron Flow 2 possible routes for electron flow during light reactions: cyclic and linear. Linear electron flow involves both photosystems and produces ATP and NADPH using light energy. 8 steps: 1) A photon hits a pigment and its energy is passed among pigment molecules until it excites P680 2) An excited electron from P680 is transferred to the primary electron acceptor 3) H2O is split by enzymes, and the electrons are transferred from the hydrogen atoms to P680+, thus reducing it to P680 (P680+ is the strongest known biological oxidizing agent, O2 is released as a by- product) 4) Electron “falls” down from the PS II to PS I 5) Create proton gradient -> ATP generated 6) Transferred light excites P700 7) Electron falls down from primary electron acceptor of PS I to the protein ferredoxin (Fd) 8) Electrons transferred to NADP+ 6. Cyclic Electron Flow In cyclic electron flow, electrons cycle back from Fd to the PS I reaction center. It uses only photosystem I and produces ATP, but not NADPH. No oxygen released. e.g. purple sulfur bacteria: only have PS I. It is thought to have evolved before linear electron flow. Cyclic electron flow may protect cells from light-induced damage. 7. Chemiosmosis in Chloroplasts vs Mitochondria Mitochondria transfer chemical energy from food to ATP. Chloroplasts transform light energy into the chemical energy of ATP. In mitochondria, protons are pumped into intermembrane space and drive ATP synthesis as they diffuse back into the mitochondrial matrix. In chloroplasts, protons are pumped into the thylakoid space and drive ATP synthesis as they diffuse back into the stroma. ATP and NADPH are produced on the side facing stroma, where the Calvin cycle occurs. Summary: Light reactions generate ATP and increase the potential energy of electrons by moving them from H2O to NADPH. Calvin Cycle uses NADPH and ATP to produce carbohydrate. Chapter 11.3 Calvin cycle Calvin cycle use ATP and NADPH to reduce CO2 to sugar The Calvin cycle builds sugar from smaller molecules by using ADP and reducing power of electrons carried by NADPH. Carbon enters the cycle as CO2 and leaves as a sugar, glyceraldehyde 3- phosphate (G3P). For net synthesis of 1 G3P, the cycle must take place 3 times, fixing 3 CO2 molecules. It has 3 phases: 1. Carbon fixation (catalyzed by rubisco) 2. Reduction 3. Regeneration of CO2 acceptor (RuBP) Chapter 11.4 Alternative mechanisms On hot, dry days, plants close stomata, which conserves H2O but also limits photosynthesis. Closing of stomata reduces access to CO2 and causes O2 to build up. This favors an apparently wasteful process, photorespiration. In photorespiration, rubisco adds O2 instead of CO2 in the Calvin cycle, producing a 2-carbon compound. Consumes O2 and organic fuel and releases CO2 without producing ATP or sugar. It is a problem in many plants as on hot, dry days it can drain up to 50% of the carbon fixed by the Calvin cycle. C4 plants minimize the cost of photorespiration by incorporating CO2 into 4-carbon compounds. 2 distinct types of cells in C4 plants’ leaves: 1) Bundle-sheath cells: arranged in tightly packed sheaths around the veins of the leaf. 2) Mesophyll cells are loosely packed between bundle sheath and leaf surface. Sugar production in C4 plants: 1. The enzyme PEP carboxylase catalyzes production of 4-carbon precursors in mesophyll cells. PEP carboxylase has higher affinity to CO2 than rubisco; it can fix CO2 even when CO2 concentrations are low. 2. 4-carbon compounds are exported to bundle-sheath cells. 3. Within the bundle-sheath cells, they release CO2 that is then used in the Calvin cycle. Some plants use crassulacean acid metabolism (CAM) to fix carbon. Cam plants open their stomata at night, incorporating CO2 into organic acids. Stomata close during the day, and CO2 is released from organic acids and then used in the Calvin cycle. Chapter 12. Mitosis Unicellular reproduces entire organism Multicellular eukaryotes depend on cell division for developments from a fertilized cell, growth, and repair. Cell division is part of the cell cycle, life of a cell from formation to its own division. Review from chapter 7: DNA organized into discrete unit of chromosomes Each chromosome is composed of a single DNA DNA + protein = chromatin Chromosomes Chromatin Tightly packaged DNA Unwound DNA Found only during cell division Found throughout interphase DNA not being used for DNA is being used for macromolecules synthesis macromolecules synthesis Chapter 12.1 Most cell division results in genetically identical daughter cells Exception is meiosis: produce sperm & egg cell 1. Cellular organization of genetic material All DNA constitutes the cell’s genome. A genome can consist of 1 or more DNA molecules. DNA molecules in a cell are packaged into chromosomes. Eukaryotic chromosomes consist of chromatin, a complex of DNA and protein that condenses during cell division. Somatic cells(nonreproductive) have 2 sets of chromosomes. Gametes(reproductive cells: sperm and eggs) have half the chromosomes as somatic cells. 2. Distribution of chromosomes during cell division DNA is replicated and the chromosomes condense. Each duplicated chromosome has 2 sister chromatids, attached along their lengths by cohesins. The centromere is the “waist” of the duplicated chromosome, where the 2 chromatids are most closely attached. During cell division, 2 sister chromatids of each duplicated chromosome separate and move into 2 nuclei. Once separate, the chromatids are called chromosomes. Eukaryotic cell division consists of: Mitosis: division of genetic material in the nucleus Cytokinesis: division of the cytoplasm Gametes(egg&sperm) are produced by a variation of cell division called meiosis. Meiosis yields nonidentical daughter cells with half as many chromosomes as the parent cell. Chapter 12.2 Mitotic phase alternates with interphase 1. Phases of cell cycle Mitotic(M) phase (mitosis and cytokinesis) Interphase (cell growth and copying of chromosomes) Interphase (90% of the cell cycle) G1 phase (first gap) S phase (synthesis) G2 phase (second gap) Cells grow during all 3 phases, but chromosomes are duplicated only in the S phase. Mitosis: Prophase Metaphase Anaphase Telophase 2. Mitotic spindle Mitotic spindle is a structure made of microtubules that controls chromosome movement during mitosis. In animal cells, assembly of spindle microtubules begins in the centrosome. Centrosome replicates during interphase, forming 2 centrosomes that migrate to opposite ends of the cell during prophase and prometaphase. An aster (radial array of short microtubules) extends from each chromosome. The spindle includes: the centrosomes, spindle microtubules, and the asters. Centromere is part of the chromosomes that link sister chromatids. During mitosis, spindle fibers attach to the centromere via the kinetochore. During prometaphase, some spindle microtubules attach to the kinetochores of chromosomes and begin to move to the chromosomes. Kinetochores are protein complexes associated with centromeres. At metaphase, the chromosomes are all lined up at the metaphase plate, plane midway between the spindle’s two poles. In anaphase the cohesins are cleaved by an enzyme called separase. Cohesins hold together the sister chromatids of each chromosome. Sister chromatids separate and move along the kinetochore microtubules toward opposite ends of the cell. Experiment proved: Microtubules shorten by depolymerizing at their kinetochore ends. Nonkinetochore microtubules from opposite poles overlap and push against each other, elongating the cell. In telophase, genetically identical daughter nuclei form at opposite ends of the cell. Cytokinesis begins during anaphase or telophase and the spindle disassembles. 3. Cytokinesis In animal cells, cytokinesis occurs by a process known as cleavage, forming a cleavage furrow. In plant cells, a cell plate forms during cytokinesis. 4. Binary fission (in prokaryotes) In binary fission, the chromosome replicates (beginning at the origin of replication), and the two daughter chromosomes actively move apart. The plasma membrane pinches inward, dividing the cell into two. 5. Evolution of mitosis Mitosis probably evolved from binary fission. Certain protists exhibit cell division intermediate between mitosis and binary fission. Chapter 12.3 Cell cycle regulated by molecular control system Frequency of cell division varies due to regulation at molecular level. Cancer cells manage to escape usual controls. Experiment conclusion: Molecules present in the cytoplasm control the progression to S and M phases 1. Cell cycle control system Similar to a clock. Regulated by internal and external controls. Specific checkpoints where cycle stops until signal is received: G1 checkpoint, G2 checkpoint, M checkpoint. a. Cell cycle clock 2 types of regulatory proteins in cell cycle: cyclins and cyclin- dependent kinases (Cdks) Activity of Cdk depends on concentration of cyclin MPF (maturation-promoting factor) is a cyclin-Cdk complex that triggers a cell’s passage past the G2 checkpoint into the M phase. b. Internal and External checkpoints The G1 checkpoint is most important. If it receives a go-ahead signal at this checkpoint, it usually completes all phases and divides. If not, it will exit the cycle, entering a nondividing state, G0 phase. External factors include growth factors, which are released by certain cells and stimulate other cells to divide. PDGF (Platelet-derived growth factor) is made by blood cell fragments called platelets. In density-dependent inhibition, crowded cells stop dividing. Most cells exhibit anchorage dependence: to divide, they must be attached to a substratum. These two check the growth of cells at optimal density. Cancer cells exhibit neither type of regulation.(does not require solid surface for division, loss of contact inhibition) 2. Loss of cell cycle controls in cancer cells Cancer cells do not respond normally to control mechanisms. May not need growth factors to grow and divide. A normal cell is converted to a cancerous cell by transformation. Cancer cells not eliminated by the immune system form tumors. If abnormal cells remain at the original site, it is a benign tumor. Malignant tumors invade surrounding tissues and can metastasize: export cancer cells to other body parts. Localized tumors may be treated with high-energy radiation, damaging the cancer cells’ DNA. To treat metastatic cancers, chemotherapies that target the cell cycle may be used. Ex) The drug taxol freezes the mitotic spindle by preventing microtubule depolymerization, which stops actively dividing cells from proceeding past metaphase. Side effects of chemotherapy are due to drugs’ effects Ex) nausea results from chemotherapy’s effects on intestinal cells Hair loss from effects on hair follicle cells Coupled with the ability to sequene the DNA of cells in a particular tumor, treatments are becoming more “personalized”: Ex) Cells of roughly 20-25% breast cancer tumors show abnormally high amounts of cell-surface receptor tyrosine kinase(RTKs) called HER2. and many show increase in the number of estrogen receptor(ER) molecules. Herceptin can be prescribed for HER2 and tamoxifen for ERs. RTKs: membrane receptors attach phosphates to tyrosines trigger multiple signal transduction pathways abnormal functioning of RTKs is associated with many types of cancer ER: intracellular receptor Chapter 13. Sexual life cycles and meiosis Chapter 13.1 Offspring acquire genes from parents by inheriting chromosomes 1. Inheritance of genes Genes are passed to the next generation via gametes. Humans have 46 chromosomes in somatic cells. Gene’s specific position along a chromosome is called the locus. 2. Asexual vs Sexual reproduction In asexual reproduction, a single individual passes all of its genes to its offspring without the fusion of gametes. A clone is a group of genetically identical individuals from the same parent. In sexual reproduction, two parents give rise to offspring that have unique combinations of genes inherited from the two parents. Chapter 13.2 Fertilization and meiosis alternate in sexual life cycles. Life cycle is the generation-to-generation sequence of stages in the reproductive history of an organism. 1. Sets of chromosomes in human cells Human somatic cells have 23 pairs of chromosomes. Karyotype: ordered display of the pairs of chromosomes from a cell. The two chromosomes in each pair are called homologous chromosomes, or homologs. Chromosomes in a homologous pair are the same length and shape and carry genes controlling the same inherited characteristics. Sex chromosomes, which determine the sex are called X or Y. Human females: homologous pair of X chromosomes (XX) Human males, one X and one Y. Remaining 22 chromosomes are autosomes. Each pair of homologous chromosomes includes one chromosome from each parent. 46 chromosomes in human somatic cell: 2 sets of 23. 1 set from mother 1 set from father A diploid cell (2n) has two sets of chromosomes. For humans, the diploid number is 46 (2n = 46). In a cell where DNA synthesis has occurred, each chromosome is replicated. Each replicated chromosome consists of two identical sister chromatids. A gamete contains a single set of chromosomes and is haploid (n). For humans, the haploid number is 23. Each set of 23 consists of 22 autosomes and a single sex chromosome. In an unfertilized egg (ovum), the sex chromosome is X. In a sperm, sex chromosome may be X or Y. 2. Behavior of Chromosome sets in the human life cycle Fertilization: union of gametes. Fertilized egg: zygote. Has one set of chromosomes from each parent. Zygote produces somatic cells by mitosis and develops into an adult. At sexual maturity. ovaries and testes produce haploid gametes. Gametes are the only types of human cells produced by meiosis. Meiosis results in one set of chromosomes in each gamete. Fertilization and meiosis alternate in sexual life cycles to maintain chromosome number. 3. Variety of sexual life cycles Alternation of meiosis and fertilization is common to all organisms that reproduce sexually. The three main types of sexual life cycles differ in timing of meiosis and fertilization. Depending on type of life cycle. Only diploid cells can undergo meiosis. In all life cycles, halving and doubling of chromosomes contribute to genetic variation in offspring. Chapter 13.3 Meiosis reduces the number of chromosome sets from diploid to haploid. Meiosis takes place in 2 consecutive cell divisions, meiosis I and meiosis II. These cell divisions result in 4 daughter cells. Each daughter cell has only half as many chromosomes as the parent cell. 1. Stages of meiosis Chromosomes duplicate during interphase. Sister chromatid cohesion: Resulting sister chromatids are closely associated along their lengths. Chromatids are sorted into 4 haploid daughter cells. Meiosis I Prophase I In early prophase I, each chromosome pairs with its homolog and crossing over occurs. X-shaped regions called chiasmata are sites of crossover. The crossing over occurs between nonsister chromatids. Metaphase I Pairs of homologs line up at the metaphase plate. Microtubules from one pole are attached to the kinetochore of one chromosome of each tetrad. Microtubules form the other pole are attached to the kinetochore of the other chromosome. Anaphase I Pair of homologous chromosomes separate. One chromosome of each pair moves towards opposite poles, guided by spindle apparatus. Sister chromatids remain attached at the centromere and move as a unit toward the pole. Telophase I and cytokinesis In the beginning of telophase I, each half of the cell has a haploid set of chromosomes; each chromosome consists of 2 sister chromatids. Cytokinesis occurs simultaneously, forming 2 haploid daughter cells. No chromosome replication occurs between end of meiosis I and beginning of meiosis II as the chromosomes are already replicated. Meiosis II Prophase II A spindle apparatus forms. Chromosomes move toward the metaphase plate. Metaphase II Sister chromatids are arranged at the metaphase plate. Due to crossing over in meiosis I, the 2 sister chromatids of each chromosome are not genetically identical. The kinetochores of sister chromatids attach to microtubules extending from opposite poles. Anaphase II Sister chromatids separate. Sister chromatids of each chromosome move as two newly individual chromosomes towards opposite poles. Telophase II and cytokinesis In telophase II, chromosomes arrive at opposite poles. Nuclei form. Chromosomes begin decondensing. Cytokinesis separates cytoplasm. At the end of meiosis, there are 4 daughter cells, each with haploid cells of unreplicated chromosomes. Each daughter is genetically distinct from the others and from the parent cell. 2. Crossing over and synapsis during prophase I After interphase, sister chromatids are held together by proteins called cohesins. Nonsister chromatids are broken at precisely corresponding positions. Synapsis (syndesis): pairing of two homologous chromosomes during meiosis. Allows matching up of homologous pairs before their segregation, and possible chromosomal crossover. Synapsis occurs during prophase I. Crossing over: exchange of genetic material between homologous chromosomes that results in recombinant chromosomes during sexual reproduction. A zipper-like structure called synaptonemal complex holds the homologs together tightly. DNA breaks are repaired, joining DNA from one nonsister chromatid to the corresponding segment of another. 3. Mitosis vs Meiosis Mitosis conserves the number of chromosome sets, producing cells genetically identical to the parent cell. Meiosis reduces the number of chromosome sets from diploid to haploid, producing cells that differ genetically from each other and from the parent cell. Events unique to meiosis. All three occur in meiosis I: Synapsis and crossing over Homologous pairs at the metaphase plate Separation of homologs during anaphase I Sister chromatid cohesion allows sister chromatids to stay together through meiosis I. In mitosis, cohesins are cleaved at the end of metaphase. In meiosis, cohesins are cleaved along the chromosome arms in anaphase I and at the centromeres in anaphase II Meiosis I is called reductional division as it reduces the number of chromosomes per cell. Chapter 13.4 Genetic variation produced in sexual life cycles contributes to evolution Mutations: changes in an organism’s DNA. They are the original source of genetic diversity. Mutations create different versions of genes called alleles· Reshuffling of alleles during sexual reproduction produces genetic variation. 1. Origins of genetic variation among offspring Three mechanisms contribute to genetic variation: - Independent assortment of chromosomes - Crossing over - Random fertilization a. Independent assortment Homologous pairs of chromosomes orient randomly at metaphase I. In the independent assortment, each pair of chromosomes sort maternal and paternal homologs into daughter cells independently of the other pairs. The number of combinations possible when chromosomes assort independently into gametes is 2n, where n is the haploid number. For humans there are more than 8 million possible combinations of chromosomes. b. Crossing over Crossing over produces recombinant chromosomes, which combine DNA inherited from each parent. Crossing over contributes to genetic variation by combining DNA from parents into a single chromosome. In humans an average of 1 to 3 crossover events occur per chromosome. c. Random fertilization Any sperm can fuse with any ovum. Fusion of 2 gametes (each with 8.4 million possible combinations) produces a zygote with any of about 70 trillion diploid combinations. 2. Evolutionary significance of genetic variation within populations Natural selection results in accumulation of genetic variations favored by the environment. Sexual reproduction contributes to the genetic variation in a population, which originates from mutations. Animals that always reproduce asexually are rare. Organisms like bdelloid rotifer increase their genetic diversity through horizontal gene transfer. Chapter 14. Mendelian genetics Chapter 14.1 Mendel discovered the basic principles of heredity by breeding garden peas in planned experiments. Character: a heritable feature that varies among individuals (flower color) Trait: Each variant for a character (purple/white color) Advantages of using peas: - Many different varieties - Short generation time - Large numbers of offspring - Mating could be controlled. Self-pollinate or cross-pollinate. Law of segregation When Mendel crossed contrasting, true-breeding white and purple flowered pea plants, all F1 hybrids were purple. When Mendel crossed F1 hybrids, many F2 plants were purple, but some had white. The ratio was 3:1, purple:white in F2 generation. Only the purple flower factor was affecting the flower color in F1 hybrids. He called the purple color a dominant trait and the white color a recessive trait. Factor for white was not diluted because it reappeared in F2 generation. Mendel observed the same pattern in 6 other characters. The “heritable factor” is the gene. Mendel’s model It has 4 concepts to explain the 3:1 inheritance pattern. 1. Alternative versions of genes account for variations in inherited characters. Alleles: alternative versions of genes. 2. For each character, an organism inherits 2 alleles, one from each parent. 3. If 2 alleles at a locus differ, then the dominant allele determines the organism’s appearance. The recessive allele has no noticeable effect. 4. Law of segregation: the 2 alleles for a heritable character segregate during gamete formation and end up in different gametes. An egg or sperm gets only one of the 2 alleles. Punnett square Capital letter: dominant allele. Lowercase letter: recessive allele. An organism with two identical alleles for a character is homozygous for the gene controlling that character. An organism with two different alleles for a character is heterozygous for the gene controlling that character. Heterozygotes are not true-breeding. An organism’s traits do not always reveal genetic composition. We distinguish between phenotype(physical appearance) and genotype(genetic makeup). Example from above, RR and Rr have the same phenotype but different genotypes. Testcross: breeding mystery individual with a homozygous recessive individual. If any offspring display the recessive phenotype, the mystery parent is heterozygous. Law of independent assortment Monohybrid: heterozygous for one character. Monohybrid cross: cross between monohybrid heterozygotes. Crossing between 2 true-breeding parents differing in 2 characters produces dihybrids in the F1 generation, heterozygous for both characters. Dihybrid cross: cross between F1 dihybrids. It can determine whether 2 characters are transmitted to offspring as a package or independently. The law of independent assortment states that each pair of alleles segregates independently of each other pair of alleles during gamete formation. It applies only to genes on different, nonhomologous chromosomes or those far apart on the same chromosome. Genes located near each other on the same chromosome tend to be inherited together. Chapter 14.3 Inheritance patterns are often more complex than predicted by simple Mendelian genetics The relationship between genotype and phenotype is rarely as simple as in the pea plant characters Mendel studied. Many heritable characters are not determined by one one gene with two alleles. Nevertheless, basic principles of segregation and independent assortment apply to even more complex patterns of inheritance. 1. Extending Mendelian genetics for a single gene Inheritance of characters by a single gene may deviate from simple Mendelian patterns: - When alleles are not completely dominant or recessive - When a gene has more than 2 alleles - When a gene produces multiple phenotypes a. Degrees of dominance Complete dominance: phenotypes of the heterozygote and dominant homozygote are identical Incomplete dominance. The phenotype of F1 hybrids is somewhere between the phenotypes of two parental varieties. Codominance. Two dominant alleles affect the phenotype in separate, distinguishable ways. Tay-Sachs disease. A dysfunctional enzyme causes an accumulation of lipids in the brain. - At organismal level, allele is recessive - At biochemical level, the phenotype (enzyme activity level) is incompletely dominant - At molecular level, the alleles are codominant Dominant alleles are not necessarily more common than recessive alleles. b. Multiple alleles Most genes exist in more than 2 allelic forms. For instance, 4 phenotypes of ABO blood group are determined by 3 alleles for the enzyme (I) that attaches A or B carbohydrates to red blood cells. IA, IB, and i. c. Pleiotropy Pleiotropy: One gene influences two or more seemingly unrelated phenotypic traits. 2. Extending Mendelian Genetics for 2 or more genes a. Epistasis In epistasis, a gene at a locus alters the phenotypic expression of a gene at a second locus. b. Polygenic inheritance Quantitative characters: those that vary in population along a continuum. Quantitative variation usually indicates polygenic inheritance, an additive effect of 2 or more genes on a single phenotype. e.g. skin color in humans. 3. Environment impact on phenotype Traits that depend on multiple genes combined with environment influences are called multifactorial. 4. Mendelian view of heredity and variation Organism’s phenotype includes its physical appearance, internal anatomy, physiology, and behavior. An organism’s phenotype reflects its overall genotype and unique environmental history. Chapter 14.4 Many human traits follow Mendelian patterns of inheritance Humans are not good subjects for research: - Generation time is too long - Parents produce relatively few offspring. - Breeding experiments are unacceptable. Basic Mendelian genetics endures as the foundation of human genetics. 1. Pedigree analysis Pedigree: family tree that describes interrelationships of parents and children across generations. Can also be used to make predictions about future offspring 2. Recessively inherited disorders Many genetic disorders are inherited in a recessive manner. These range from relatively mild to life-threatening. a. Behavior of recessive alleles Recessively inherited disorders show up only in individuals homozygous for the allele. Carriers: heterozygous individuals who carry the recessive allele but are phenotypically normal. Most individuals with recessive disorders are born to carrier parents. Albinism is a recessive condition characterized by lack of pigmentation in skin and hair. Consanguineous marriage increases the chance of mating between 2 carriers of the same rare allele. b. Cystic fibrosis Cystic fibrosis is the most common lethal genetic disease in the US. 1 out of every 2500 people of European descent. Cystic fibrosis allele results in defective or absent chloride transport channels in plasma membranes leading to a buildup of chloride ions outside the cell. Symptoms include mucus buildup in internal organs and abnormal absorption of nutrients in the small intestine. c. Sickle-cell disease It affects 1 out of 400 African-Americans. It is caused by substitution of a single amino acid in the hemoglobin protein. In homozygous individuals, all hemoglobin is abnormal. Symptoms include physical weakness, pain, organ damage, or even paralysis. Heterozygotes are usually healthy but may suffer some symptoms. 1 out of 10 African Americans has sickle-cell trait. Heterozygotes are less susceptible to malaria parasites, so there is an advantage to being heterozygous in regions where malaria is common. 3. Dominantly inherited disorders Some human disorders are caused by dominant alleles. Achondroplasia is a form of dwarfism caused by a rare dominant allele. Huntington’s disease is a degenerative disease of the nervous system. It has no obvious phenotypic effects until the individual is 35-40 years old. Once deterioration of the nervous system begins the condition is irreversible and fatal. 4. Multifactorial disorders Many diseases have both genetic and environmental components. Ex) heart disease, diabetes, alcoholism, mental illness and cancer 5. Genetic testing and counseling Genetic counselors can provide information to prospective parents concerned about a family history for a specific disease. a. Counseling based on Mendelian genetics and Probability rules b. Tests for identifying carriers c. Fetal testing Amniocentesis. The liquid that bathes the fetus is removed and tested. Chorionic villus sampling (CVS). A sample of the placenta is removed and tested. Other techniques, such as ultrasound and fetoscopy, allow fetal health to be assessed visually in utero. d. Newborn screening Some genetic disorders can be detected at birth by simple tests. One common test is for phenylketonuria (PKU), a recessively inherited disorder. Treatment is with a diet low in foods that contain phenylalanine and special supplements Chapter 15. Linkage and chromosomes Boveri-Sutton chromosome theory (chromosome theory of inheritance) states that chromosomes are the basis for all genetic inheritance. Chapter 15.1 Morgan showed that Mendelian inheritance has its physical basis in the behavior of chromosomes. 1. Morgan’s choice of experimental organism Advantages of using Drosophila melanogaster, fruit fly: - Many offspring - Generation bred every 2 weeks - Only 4 pairs of chromosomes Morgan noted wild type (normal) phenotypes common in fly populations. Traits alternative to wild type are mutant phenotypes. 2. Correlating behavior of a gene’s alleles with behavior of a chromosome pair Morgan mated male flies with white (mutant eyes) and female flies with red (wild type) eyes. The F1 generation all had red eyes. F2 generation showed 3:1, red: white, but only males had white eyes. White-eyed allele must be located on X chromosomes → support chromosomes theory of inheritance Chapter 15.2 Sex-linked genes exhibit unique patterns of inheritance 1. Chromosomal basis of sex In humans and mammals: larger X chromosome and smaller Y chromosome. Person with 2 X chromosomes is female, while male develops from zygote with one X and one Y. Short segments at the ends of Y chromosomes are homologous with the X, allowing the two to behave like homologues during meiosis in males. A gene on the Y chromosome called SRY(sec-determining region) is responsible for development of testes in an embryo. Sex-linked gene: Gene located on either sex chromosome. Genes on Y chromosomes are Y-linked genes, only few of these. Genes on the X chromosome are X-linked genes. 2. Inheritance of X-linked genes X chromosomes have genes for many characters unrelated to sex. Most Y- linked genes are related to sex determination. For a recessive X-linked trait to be expressed: - A female needs 2 copies of the allele (homozygous) - A male needs only 1 copy of the allele (hemizygous) → X-linked recessive disorders are much more common in males. Heterozygous: two alleles of a diploid organism are different at a locus Hemizygous: one allele is missing at a locus Nullizygous: both alleles are missing at a locus. Disorders caused by recessive alleles on X chromosome: a. Color blindness X-linked recessive traits Female: needs two copies of recessive alleles Male: only one copy b. Duchenne muscular dystrophy Progressive weakening of muscles and loss of coordination Caused by absence of a key muscle protein: dystrophin. (x chromosome) Dystrophin is located between the sarcolemma and the outermost of myofilaments in the myofiber. Cohesive protein that links actin filaments to another support protein. c. Hemophilia Absence of one or more proteins required for blood clotting. Today treated as needed with intravenous injections of missing protein. Hemophilia A: insufficient clotting factor VIII Hemophilia B: insufficient clotting factor IX 3. X inactivation in female mammals In mammalian females, one of the two X chromosomes is randomly inactivated. Inactive X condenses into a Barr body. If a female is heterozygous for a particular gene on the X chromosome, she will be a mosaic for that character. Tortoiseshell gene: on x chromosome. If a female cat is heterozygous for the tortoiseshell gene, she is tortoiseshell Chapter 15.3 Linked genes: genes located on the same chromosome that tend to be inherited together. 1. How linkage affects inheritance Morgan crossed flies that differed in body color and wing size. He found that body color and wing size are usually inherited together in specific combinations (parental phenotype). These genes do not assort independently → they were on the same chromosome. Nonparental phenotypes were also produced. Genetic recombination: production of offspring with combinations of traits differing from either parent. 2. Genetic recombination and linkage a. Unlinked genes: Independent assortment of chromosomes Parental types: Offspring with phenotype matching one of the parental phenotypes. Recombinant types (recombinants): Offspring with nonparental phenotypes (new combinations of traits). 50% of frequency of recombination observed for any 2 genes on different chromosomes. b. Linked genes: Crossing over Morgan discovered that genes can be linked, but the linkage was incomplete because some recombinant phenotypes were observed. Some process must break the physical connection between genes on the same chromosome → crossing over of homologous chromosomes. c. New combinations of alleles: natural selection Recombinant chromosomes bring alleles together in new combinations in gametes. Random fertilization increases further the number of variant combinations. If traits conferred by particular combinations are better suited for an environment, organisms possessing those genotypes will leave more offspring, continuing their genetic complement. 3. Mapping distance between genes using recombination data Genetic map: ordered list of the genetic loci song a particular chromosome. Sturtevant predicted that the farther apart two genes are, the higher the probability that a crossover will occur between them and therefore the higher the recombination frequency. Linkage map: genetic map of a chromosome based on recombination frequencies. Distances between genes can be expressed as map units. One map unit, or centimorgan, represents 1% recombination frequency. Map units represent relative distance order. Genes far apart on the same chromosome can have recombination frequency near 50%. Such genes are physically linked, but genetically unlinked, and behave as if found on different chromosomes. Sturtevant made linkage maps of fruit fly genes. Found that genes clustered into 4 groups of linkage groups. Chapter 15.4 Alterations of chromosome number or structure cause some genetic disorders. 1. Abnormal chromosome number Nondisjunction: pairs of homologous chromosomes do not separate normally during meiosis. One gamete receives 2 of the same type of chromosome, and another gamete receives no copy. Aneuploidy: presence of abnormal number of chromosomes in a cell. Results from fertilization of gametes in which nondisjunction occurred. Monosomic zygote has only one copy of a particular chromosome. Trisomic zygote has 3 copies of a particular chromosome. Polyploidy: condition in which an organism has more than 2 complete sets of chromosomes. Triploidy (3n) Tetraploidy (4n) Polyploidy is common in plants. Polyploids are more normal in appearance than aneuploids. 2. Alterations of chromosome structure Breakage of a chromosome can lead to 4 types of changes in chromosome structure: Deletion removes a chromosomal segment. Duplication repeats a segment. Inversion reverses orientation of a segment within a chromosome. Translocation moves a segment from one chromosome to another. 3. Human disorders due to chromosomal alterations a. Down syndrome (Trisomy 21) Aneuploid condition that results from 3 copies of chromosome 21. Affects about one out of every 700 children born in the US. Frequency of Down Syndrome increases with the age of the mother. b. Aneuploidy of sex chromosomes Nondisjunction of sex chromosomes produces a variety of aneuploidy conditions. XXX females are healthy. Klinefelter syndrome: result of an extra chromosome in a male, producing XXY individuals (sterile). Monosomy X, called Turner syndrome, produces X0 females, who are sterile. It is the only known viable monosomy in humans. c. Disorders caused by structurally altered chromosomes The syndrome cri du chat (“cry of the cat”), results from a specific deletion in chromosome 5. A child born with this syndrome is severely intellectually disabled and has a catlike cry. Usually die early. Certain cancers, including chronic myelogenous leukemia (CML), are caused by translocations of chromosomes. Cancerous cells in nearly all CML patients contain an abnormally short chromosome 22, the Philadelphia chromosome. Chapter 15.5 Some inheritance patterns are exceptions to standard Mendelian inheritance. 2 normal exceptions to Mendelian genetics: genes located in the nucleus, genes located outside the nucleus In both cases, the sex of the parent contributing an allele is a factor in the pattern of inheritance. 1. Genomic imprinting For a few mammalian traits, the phenotype depends on which parent passed along the alleles for those traits. Such variation in phenotype is genomic imprinting. It involves silencing of certain genes depending on which parent passes them on. Genomic imprinting is the epigenetic process that involves DNA methylation and histone methylation without altering genetic sequence. Ex) a. Homozygote: a mouse homozygous for the wild-type lgf2 allele is normal-sized. Only the paternal allele of this gene is expressed b. Heterozygote: mating between wild-type mice and those homozygous for the recessive mutant lgf2 allele produce heterozygous offspring. The dwarf(mutant) phenotype is seen only when the father contributed the mutant allele because the maternal allele is not expressed. 2. Inheritance of organelle genes Extranuclear genes are found in organelles in cytoplasm. Extranuclear genes are inherited maternally because the zygote’s cytoplasm comes from the egg. Plastid is a major double-membrane organelle found in cells of plants and algae. It is the site of manufacture and storage of important chemical compounds. A pattern of leaf coloration exhibited by a plant depends on the ratio of wild-type to mutant plastids in its various tissues. Some defects in mitochondrial genes prevent cells from making enough ATP → diseases affect the muscular and nervous system Ex) mitochondrial myopathy and Leber’s hereditary optic neuropathy. In both cases, sex of the parent contributing an allele is a factor in the pattern of inheritance. 2019 William G. Kaelin Jr., Sir Peter J. Ratcliffe and Gregg L. Semenza for their discoveries of how cells sense and adapt to oxygen availability SUMMARY Animals need oxygen for the conversion of food into useful energy. The fundamental importance of oxygen has been understood for centuries, but how cells adapt to changes in levels of oxygen has long been unknown. William G. Kaelin Jr., Sir Peter J. Ratcliffe and Gregg L. Semenza discovered how cells can sense and adapt to changing oxygen availability. They identified molecular machinery that regulates the activity of genes in response to varying levels of oxygen. The seminal discoveries by this year’s Nobel Laureates revealed the mechanism for one of life’s most essential adaptive processes. They established the basis for our understanding of how oxygen levels affect cellular metabolism and physiological function. Their discoveries have also paved the way for promising new strategies to fight anemia, cancer and many other diseases. 동물은 음식을 유용한 에너지로 전환하기 위해 산소가 필요합니다. 산소의 근본적인 중요성은 수세기 동안 이해되어 왔지만, 세포가 산소 수준의 변화에 어떻게 적응하는지는 오랫동안 알려지지 않았습니다. 윌리엄 G. 케일린 주니어, 피터 J. 래틀리프 경, 그레그 L. 세멘자는 세포가 어떻게 산소 가용성의 변화를 감지하고 적응할 수 있는지를 발견했습니다. 그들은 산소 수준의 변화에 반응하여 유전자의 활동을 조절하는 분자 기계를 식별했습니다. 올해 노벨상 수상자들의 획기적인 발견은 생명체의 가장 중요한 적응 과정 중 하나에 대한 메커니즘을 밝혀냈습니다. 그들은 산소 수준이 세포 대사와 생리적 기능에 어떻게 영향을 미치는지에 대한 우리의 이해의 기초를 확립했습니다. 그들의 발견은 빈혈, 암 및 많은 다른 질병을 치료하기 위한 유망한 새로운 전략의 길을 열었습니다. Oxygen at center stage Oxygen, with the formula O2, makes up about one fifth of Earth’s atmosphere. Oxygen is essential for animal life: it is used by the mitochondria present in virtually all animal cells in order to convert food into useful energy. Otto Warburg, the recipient of the 1931 Nobel Prize in Physiology or Medicine, revealed that this conversion is an enzymatic process. During evolution, mechanisms developed to ensure a sufficient supply of oxygen to tissues and cells. The carotid body, adjacent to large blood vessels on both sides of the neck, contains specialized cells that sense the blood’s oxygen levels. The 1938 Nobel Prize in Physiology or Medicine to Corneille Heymansawarded discoveries showing how blood oxygen sensing via the carotid body controls our respiratory rate by communicating directly with the brain. 산소(O₂)는 지구 대기의 약 5 분의 1 을 차지합니다. 산소는 동물의 생명에 필수적입니다. 산소는 거의 모든 동물 세포에 존재하는 미토콘드리아에 의해 음식물을 유용한 에너지로 전환하는 데 사용됩니다. 1931 년 노벨 생리의학상 수상자인 오토 바르부르크는 이 전환이 효소 과정이라는 것을 밝혀냈습니다. 진화 과정에서 조직과 세포에 충분한 산소 공급을 보장하기 위한 메커니즘이 발달했습니다. 목의 양쪽 큰 혈관 옆에 있는 경동맥체에는 혈액의 산소 수준을 감지하는 특수 세포가 있습니다. 1938 년 노벨 생리의학상 수상자인 코르네일 헤이만스는 경동맥체를 통한 혈액 산소 감지가 뇌와 직접 소통하여 우리의 호흡률을 조절하는 방법을 발견했습니다. HIF enters the scene In addition to the carotid body-controlled rapid adaptation to low oxygen levels (hypoxia), there are other fundamental physiological adaptations. A key physiological response to hypoxia is the rise in levels of the hormone erythropoietin (EPO), which leads to increased production of red blood cells (erythropoiesis). The importance of hormonal control of erythropoiesis was already known at the beginning of the 20th century, but how this process was itself controlled by O2 remained a mystery. 경동맥체가 조절하는 저산소증에 대한 빠른 적응 외에도 다른 근본적인 생리학적 적응이 있습니다. 저산소증에 대한 주요 생리적 반응 중 하나는 적혈구 생성 호르몬인 에리트로포이에틴(EPO) 수치의 상승입니다. 이는 적혈구 생성(적혈구형성)의 증가로 이어집니다. 적혈구형성의 호르몬 조절의 중요성은 20 세기 초부터 이미 알려져 있었지만, 이 과정이 어떻게 O₂에 의해 조절되는지는 여전히 미스터리였습니다. Gregg Semenza studied the EPO gene and how it is regulated by varying oxygen levels. By using gene-modified mice, specific DNA segments located next to the EPO gene were shown to mediate the response to hypoxia. Sir Peter Ratcliffe also studied O2-dependent regulation of the EPO gene, and both research groups found that the oxygen sensing mechanism was present in virtually all tissues, not only in the kidney cells where EPO is normally produced. These were important findings showing that the mechanism was general and functional in many different cell types. 그레그 세멘자는 EPO 유전자와 그것이 다양한 산소 수준에 의해 어떻게 조절되는지를 연구했습니다. 유전자 변형 쥐를 사용하여, EPO 유전자 옆에 위치한 특정 DNA 조각들이 저산소증에 대한 반응을 중재하는 것으로 나타났습니다. 피터 래틀리프 경도 EPO 유전자의 산소 의존적 조절을 연구했고, 두 연구 그룹은 산소 감지 메커니즘이 EPO 가 일반적으로 생성되는 신장 세포뿐만 아니라 거의 모든 조직에서 존재한다는 것을 발견했습니다. 이러한 발견은 메커니즘이 여러 다른 세포 유형에서 일반적이고 기능적임을 보여주는 중요한 발견이었습니다. Semenza wished to identify the cellular components mediating this response. In cultured liver cells he discovered a protein complex that binds to the identified DNA segment in an oxygen-dependent manner. He called this complex the hypoxia- inducible factor (HIF). Extensive efforts to purify the HIF complex began, and in 1995, Semenza was able to publish some of his key findings, including identification of the genes encoding HIF. HIF was found to consist of two different DNA-binding proteins, so called transcription factors, now named HIF-1α and ARNT. Now the researchers could begin solving the puzzle, allowing them to understand which additional components were involved and how the machinery works. 세멘자는 이 반응을 매개하는 세포 구성 요소를 확인하고자 했습니다. 배양된 간 세포에서 그는 식별된 DNA 조각에 산소 의존적으로 결합하는 단백질 복합체를 발견했습니다. 그는 이 복합체를 저산소 유도 인자(HIF)라고 명명했습니다. HIF 복합체를 정제하려는 광범위한 노력이 시작되었고, 1995 년 세멘자는 HIF 를 암호화하는 유전자의 식별을 포함한 몇 가지 주요 발견을 발표할 수 있었습니다. HIF 는 두 개의 다른 DNA 결합 단백질로 구성된 것으로 밝혀졌으며, 이들은 현재 HIF-1α 와 ARNT 로 명명되었습니다. 이제 연구자들은 퍼즐을 풀기 시작할 수 있었고, 추가적인 구성 요소들이 어떤 역할을 하며 이 기계가 어떻게 작동하는지 이해할 수 있게 되었습니다. VHL: an unexpected partner When oxygen levels are high, cells contain very little HIF-1α. However, when oxygen levels are low, the amount of HIF-1α increases so that it can bind to and thus regulate the EPO gene as well as other genes with HIF-binding DNA segments (Figure 1). Several research groups showed that HIF-1α, which is normally rapidly degraded, is protected from degradation in hypoxia. At normal oxygen levels, a cellular machine called the proteasome, recognized by the 2004 Nobel Prize in Chemistry to Aaron Ciechanover, Avram Hershko and Irwin Rose, degrades HIF-1α. Under such conditions a small peptide, ubiquitin, is added to the HIF-1α protein. Ubiquitin functions as a tag for proteins destined for degradation in the proteasome. How ubiquitin binds to HIF-1α in an oxygen-dependent manner remained a central question. 산소 수치가 높을 때, 세포에는 HIF-1α 가 거의 없습니다. 그러나 산소 수치가 낮을 때 HIF- 1α 의 양이 증가하여 EPO 유전자와 HIF-결합 DNA 조각을 가진 다른 유전자를 결합하고 조절할 수 있게 됩니다(Figure 1). 여러 연구 그룹은 정상적으로 빠르게 분해되는 HIF-1α 가 저산소증에서는 분해로부터 보호된다는 것을 보여주었습니다. 정상적인 산소 수치에서는 2004 년 노벨 화학상 수상자 아론 치하노버, 아브람 허시코, 어윈 로즈에 의해 인식된 프로테아좀이라는 세포 기계가 HIF-1α 를 분해합니다. 이러한 조건에서 소형 펩타이드인 유비퀴틴이 HIF-1α 단백질에 추가됩니다. 유비퀴틴은 프로테아좀에서 분해될 단백질을 태그하는 역할을 합니다. 유비퀴틴이 산소 의존적으로 HIF-1α 에 결합하는 방법은 여전히 중요한 질문이었습니다. The answer came from an unexpected direction. At about the same time as Semenza and Ratcliffe were exploring the regulation of the EPO gene, cancer researcher William Kaelin, Jr. was researching an inherited syndrome, von Hippel-Lindau’s disease (VHL disease). This genetic disease leads to dramatically increased risk of certain cancers in families with inherited VHL mutations. Kaelin showed that the VHL gene encodes a protein that prevents the onset of cancer. Kaelin also showed that cancer cells lacking a functional VHL gene express abnormally high levels of hypoxia-regulated genes; but that when the VHL gene was reintroduced into cancer cells, normal levels were restored. This was an important clue showing that VHL was somehow involved in controlling responses to hypoxia. Additional clues came from several research groups showing that VHL is part of a complex that labels proteins with ubiquitin, marking them for degradation in the proteasome. Ratcliffe and his research group then made a key discovery: demonstrating that VHL can physically interact with HIF-1α and is required for its degradation at normal oxygen levels. This conclusively linked VHL to HIF-1α. 답은 예상치 못한 방향에서 나왔습니다. 세멘자와 래틀리프가 EPO 유전자의 조절을 연구하고 있을 때, 암 연구자인 윌리엄 케일린 주니어는 유전적 증후군인 폰 히펠-린다우 질병(VHL 질병)을 연구하고 있었습니다. 이 유전 질병은 VHL 돌연변이를 유전하는 가족에서 특정 암의 위험을 극적으로 증가시킵니다. 케일린은 VHL 유전자가 암의 발병을 방지하는 단백질을 암호화한다는 것을 보여주었습니다. 케일린은 또한 기능성 VHL 유전자가 없는 암세포가 비정상적으로 높은 수준의 저산소증 조절 유전자를 발현하지만, VHL 유전자가 암세포에 다시 도입되었을 때 정상 수준으로 회복된다는 것을 보여주었습니다. 이는 VHL 이 저산소증에 대한 반응을 조절하는 데 어떤 방식으로든 관련되어 있음을 보여주는 중요한 단서였습니다. 추가 단서는 여러 연구 그룹에서 VHL 이 단백질을 유비퀴틴으로 표시하여 프로테아좀에서 분해되도록 하는 복합체의 일부라는 것을 보여주면서 나왔습니다. 래틀리프와 그의 연구 그룹은 VHL 이 HIF-1α 와 물리적으로 상호작용할 수 있으며 정상적인 산소 수치에서 HIF-1α 의 분해에 필요하다는 중요한 발견을 했습니다. 이는 VHL 을 HIF-1α 와 결정적으로 연결했습니다. Oxygen sHIFts the balance Many pieces had fallen into place, but what was still lacking was an understanding of how O2 levels regulate the interaction between VHL and HIF-1α. The search focused on a specific portion of the HIF-1α protein known to be important for VHL-dependent degradation, and both Kaelin and Ratcliffe suspected that the key to O2-sensing resided somewhere in this protein domain. In 2001, in two simultaneously published articles they showed that under normal oxygen levels, hydroxyl groups are added at two specific positions in HIF-1α (Figure 1). This protein modification, called prolyl hydroxylation, allows VHL to recognize and bind to HIF-1α and thus explained how normal oxygen levels control rapid HIF-1α degradation with the help of oxygen- sensitive enzymes (so-called prolyl hydroxylases). Further research by Ratcliffe and others identified the responsible prolyl hydroxylases. It was also shown that the gene activating function of HIF-1α was regulated by oxygen-dependent hydroxylation. The Nobel Laureates had now elucidated the oxygen sensing mechanism and had shown how it works. 많은 부분이 자리를 잡았지만, 여전히 산소 수준이 VHL 과 HIF-1α 의 상호작용을 어떻게 조절하는지에 대한 이해가 부족했습니다. 연구는 VHL 의존적 분해에 중요한 것으로 알려진 HIF-1α 단백질의 특정 부분에 집중되었고, 케일린과 래틀리프는 산소 감지의 핵심이 이 단백질 도메인 어딘가에 있다고 의심했습니다. 2001 년, 두 개의 동시에 발표된 논문에서 그들은 정상적인 산소 수치에서는 HIF-1α 의 두 특정 위치에 하이드록실 그룹이 추가된다는 것을 보여주었습니다(Figure 1). 이 단백질 수정은 프로릴 하이드록실화라고 불리며, VHL 이 HIF-1α 를 인식하고 결합할 수 있게 하여 정상적인 산소 수치가 산소 민감성 효소(이른바 프로릴 하이드록실레이스)의 도움으로 HIF-1α 의 빠른 분해를 조절하는 방법을 설명했습니다. 래틀리프와 다른 연구자들의 추가 연구는 책임 있는 프로릴 하이드록실레이스를 식별했습니다. 또한 HIF-1α 의 유전자 활성화 기능이 산소 의존적 하이드록실화에 의해 조절된다는 것도 밝혀졌습니다. 노벨 수상자들은 이제 산소 감지 메커니즘을 밝혀내고 그것이 어떻게 작동하는지 보여주었습니다. Figure 1. When oxygen levels are low (hypoxia), HIF-1α is protected from degradation and accumulates in the nucleus, where it associates with ARNT and binds to specific DNA sequences (HRE) in hypoxia-regulated genes (1). At normal oxygen levels, HIF-1α is rapidly degraded by the proteasome (2). Oxygen regulates the degradation process by the addition of hydroxyl groups (OH) to HIF-1α (3). The VHL protein can then recognize and form a complex with HIF-1α leading to its degradation in an oxygen-dependent manner (4). Oxygen shapes physiology and pathology Thanks to the groundbreaking work of these Nobel Laureates, we know much more about how different oxygen levels regulate fundamental physiological processes. Oxygen sensing allows cells to adapt their metabolism to low oxygen levels: for example, in our muscles during intense exercise. Other examples of adaptive processes controlled by oxygen sensing include the generation of new blood vessels and the production of red blood cells. Our immune system and many other physiological functions are also fine-tuned by the O2-sensing machinery. Oxygen sensing has even been shown to be essential during fetal development for controlling normal blood vessel formation and placenta development. 이 노벨상 수상자들의 획기적인 연구 덕분에 우리는 다양한 산소 수준이 기본적인 생리적 과정을 어떻게 조절하는지에 대해 훨씬 더 많이 알게 되었습니다. 산소 감지는 세포가 낮은 산소 수준에 대사적으로 적응할 수 있게 합니다. 예를 들어, 격렬한 운동 중 우리 근육에서처럼 말입니다. 산소 감지에 의해 조절되는 적응 과정의 다른 예로는 새로운 혈관 생성과 적혈구 생성이 있습니다. 우리의 면역 체계와 많은 다른 생리적 기능들도 산소 감지 기계에 의해 세밀하게 조절됩니다. 산소 감지는 태아 발달 중에도 정상적인 혈관 형성과 태반 발달을 조절하는 데 필수적임이 밝혀졌습니다. Oxygen sensing is central to a large number of diseases (Figure 2). For example, patients with chronic renal failure often suffer from severe anemia due to decreased EPO expression. EPO is produced by cells in the kidney and is essential for controlling the formation of red blood cells, as explained above. Moreover, the oxygen-regulated machinery has an important role in cancer. In tumors, the oxygen- regulated machinery is utilized to stimulate blood vessel formation and reshape metabolism for effective proliferation of cancer cells. Intense ongoing efforts in academic laboratories and pharmaceutical companies are now focused on developing drugs that can interfere with different disease states by either activating, or blocking, the oxygen-sensing machinery. 산소 감지는 많은 질병의 중심입니다(Figure 2). 예를 들어, 만성 신부전 환자는 종종 EPO 발현 감소로 인한 심각한 빈혈을 겪습니다. EPO 는 신장의 세포에 의해 생성되며, 위에서 설명한 바와 같이 적혈구 형성을 조절하는 데 필수적입니다. 게다가, 산소 조절 기계는 암에서 중요한 역할을 합니다. 종양에서는 산소 조절 기계가 혈관 생성을 자극하고 암세포의 효과적인 증식을 위해 대사를 재구성하는 데 이용됩니다. 현재 학계 연구실과 제약 회사에서는 산소 감지 기계를 활성화하거나 차단하여 다양한 질병 상태에 개입할 수 있는 약물을 개발하는 데 집중적인 노력을 기울이고 있습니다. Figure 2. The awarded mechanism for oxygen sensing has fundamental importance in physiology, for example for our metabolism, immune response and ability to adapt to exercise. Many pathological processes are also affected. Intensive efforts are ongoing to develop new drugs that can either inhibit or activate the oxygen-regulated machinery for treatment of anemia, cancer and other diseases. 2020 The Nobel Assembly at Karolinska Institutet has today decided to award the 2020 Nobel Prize in Physiology or Medicine jointly to Harvey J. Alter, Michael Houghton and Charles M. Rice for the discovery of Hepatitis C virus. 하비 J. 알터, 마이클 호튼, 찰스 M. 라이스는 C 형 간염 바이러스의 발견으로 2020 년 생리학 및 의학 부문 노벨상을 받게 되었다. SUMMARY This year’s Nobel Prize is awarded to three scientists who have made a decisive contribution to the fight against blood-borne hepatitis, a major global health problem that causes cirrhosis and liver cancer in people around the world. 올해의 노벨상은 혈액 매개형 간염과의 싸움에 결정적인 기여를 한 세 명의 과학자들에게 수여된다. 혈액 매개형 간염은 전 세계적으로 간경변 및 간암을 일으키는 주요 글로벌 건강 문제이다. Harvey J. Alter, Michael Houghton and Charles M. Rice made seminal discoveries that led to the identification of a novel virus, Hepatitis C virus. Prior to their work, the discovery of the Hepatitis A and B viruses had been critical steps forward, but the majority of blood- borne hepatitis cases remained unexplained. The discovery of Hepatitis C virus revealed the cause of the remaining cases of chronic hepatitis and made possible blood tests and new medicines that have saved millions of lives. 하비 J. 알터, 마이클 호튼, 찰스 M. 라이스는 C 형 간염 바이러스라는 새로운 바이러스를 식별하는 데 결정적인 발견을 했다. 이들의 연구 이전에 A 형과 B 형 간염 바이러스의 발견은 중요한 진전이었지만, 대부분의 혈액 매개형 간염 사례는 여전히 설명되지 않았다. C 형 간염 바이러스의 발견은 만성 간염의 나머지 사례의 원인을 밝혀냈고, 수백만 명의 생명을 구한 혈액 검사와 새로운 약물의 개발을 가능하게 했다. Hepatitis – a global threat to human health Liver inflammation, or hepatitis, a combination of the Greek words for liver and inflammation, is mainly caused by viral infections, although alcohol abuse, environmental toxins and autoimmune disease are also important causes. In the 1940’s, it became clear that there are two main types of infectious hepatitis. The first, named hepatitis A, is transmitted by polluted water or food and generally has little long-term impact on the patient. The second type is transmitted through blood and bodily fluids and represents a much more serious threat since it can lead to a chronic condition, with the development of cirrhosis and liver cancer (Figure 1). This form of hepatitis is insidious, as otherwise healthy individuals can be silently infected for many years before serious complications arise. Blood-borne hepatitis is associated with significant morbidity and mortality, and causes more than a million deaths per year world-wide, thus making it a global health concern on a scale comparable to HIV-infection and tuberculosis. 간염 – 인간 건강에 대한 글로벌 위협 간 염증, 즉 간염은 그리스어로 간과 염증을 결합한 단어로, 주로 바이러스 감염에 의해 발생하지만, 알코올 남용, 환경 독소 및 자가면역 질환도 중요한 원인이다. 1940 년대에 두 가지 주요 유형의 전염성 간염이 있다는 것이 분명해졌다. 첫 번째 유형은 A 형 간염으로, 오염된 물이나 음식을 통해 전염되며 일반적으로 환자에게 장기적인 영향을 미치지 않는다. 두 번째 유형은 혈액과 체액을 통해 전염되며, 만성 상태로 발전하여 간경변 및 간암을 유발할 수 있기 때문에 훨씬 더 심각한 위협을 나타낸다 (그림 1). 이 형태의 간염은 교활하게도 건강한 개인도 수년 동안 조용히 감염될 수 있어 심각한 합병증이 발생하기 전에는 감지되지 않는다. 혈액 매개형 간염은 상당한 이환율과 사망률을 동반하며 전 세계적으로 매년 백만 명 이상의 사망을 초래하여 HIV 감염 및 결핵과 비교할 만한 글로벌 건강 문제로 자리잡았다. Figure 1. There are two main forms of hepatitis. One form is an acute disease caused by Hepatitis A virus that is transmitted by contaminated water or food. The other form is caused by Hepatitis B virus or Hepatitis C virus (this year’s Nobel prize). This form of blood- borne hepatitis is often a chronic disease that may progress to cirrhosis and hepatocellular carcinoma. 그림 1. 간염에는 두 가지 주요 형태가 있다. 한 형태는 오염된 물이나 음식을 통해 전염되는 A 형 간염 바이러스에 의해 발생하는 급성 질환이다. 다른 형태는 B 형 간염 바이러스 또는 C 형 간염 바이러스(올해의 노벨상 수상)로 인해 발생한다. 이 혈액 매개형 간염은 종종 만성 질환으로 발전하여 간경변 및 간세포 암종으로 진행될 수 있다. An unknown infectious agent The key to successful intervention against infectious diseases is to identify the causative agent. In the 1960’s, Baruch Blumberg determined that one form of blood-borne hepatitis was caused by a virus that became known as Hepatitis B virus, and the discovery led to the development of diagnostic tests and an e`ective vaccine. Blumberg was awarded the Nobel Prize in Physiology or Medicine in 1976 for this discovery. 알려지지 않은 감염성 병원체 전염병에 대한 성공적인 개입의 열쇠는 원인 병원체를 식별하는 것이다. 1960 년대에 바루크 블럼버그는 혈액 매개형 간염의 한 형태가 B 형 간염 바이러스로 알려진 바이러스에 의해 발생한다는 것을 밝혀냈고, 이 발견은 진단 검사와 효과적인 백신 개발로 이어졌다. 블럼버그는 이 발견으로 1976 년 생리학 및 의학 부문 노벨상을 수상했다. At that time, Harvey J. Alter at the US National Institutes of Health was studying the occurrence of hepatitis in patients who had received blood transfusions. Although blood tests for the newly-discovered Hepatitis B virus reduced the number of cases of transfusion-related hepatitis, Alter and colleagues worryingly demonstrated that a large number of cases remained. Tests for Hepatitis A virus infection were also developed around this time, and it became clear that Hepatitis A was not the cause of these unexplained cases. 당시, 미국 국립보건원(NIH)의 하비 J. 알터는 수혈을 받은 환자들에서 간염의 발생을 연구하고 있었다. 새로 발견된 B 형 간염 바이러스에 대한 혈액 검사가 수혈 관련 간염 사례를 줄였지만, 알터와 동료들은 여전히 많은 사례가 남아 있다는 것을 걱정스럽게 증명했다. 이 무렵 A 형 간염 바이러스 감염에 대한 검사도 개발되었고, A 형 간염이 이러한 설명되지 않은 사례의 원인이 아님이 분명해졌다. It was a great source of concern that a significant number of those receiving blood transfusions developed chronic hepatitis due to an unknown infectious agent. Alter and his colleagues showed that blood from these hepatitis patients could transmit the disease to chimpanzees, the only susceptible host besides humans. Subsequent studies also demonstrated that the unknown infectious agent had the characteristics of a virus. Alter’s methodical investigations had in this way defined a new, distinct form of chronic viral hepatitis. The mysterious illness became known as “non-A, non-B” hepatitis. 수혈을 받은 상당수의 사람들이 알려지지 않은 감염성 병원체로 인해 만성 간염에 걸리는 것은 큰 우려 사항이었다. 알터와 그의 동료들은 이러한 간염 환자의 혈액이 인간 외에 유일한 감수성 숙주인 침팬지에게 질병을 전염시킬 수 있음을 보여주었다. 후속 연구에서도 이 알려지지 않은 감염성 병원체가 바이러스의 특성을 가지고 있음을 증명했다. 알터의 체계적인 조사 덕분에 새로운, 구별되는 형태의 만성 바이러스성 간염이 정의되었다. 이 신비한 질병은 '비 A 형, 비 B 형' 간염으로 알려지게 되었다. Identification of Hepatitis C virus Identification of the novel virus was now a high priority. All the traditional techniques for virus hunting were put to use but, in spite of this, the virus eluded isolation for over a decade. Michael Houghton, working for the pharmaceutical firm Chiron, undertook the arduous work needed to isolate the genetic sequence of the virus. Houghton and his co- workers created a collection of DNA fragments from nucleic acids found in the blood of an infected chimpanzee. The majority of these fragments came from the genome of the chimpanzee itself, but the researchers predicted that some would be derived from the unknown virus. On the assumption that antibodies against the virus would be present in blood taken from hepatitis patients, the investigators used patient sera to identify cloned viral DNA fragments encoding viral proteins. Following a comprehensive search, one positive clone was found. Further work showed that this clone was derived from a novel RNA virus belonging to the Flavivirus family and it was named Hepatitis C virus. The presence of antibodies in chronic hepatitis patients strongly implicated this virus as the missing agent. C 형 간염 바이러스의 식별 이제 새로운 바이러스를 식별하는 것이 높은 우선순위가 되었다. 바이러스 탐색을 위한 모든 전통적인 기법이 사용되었지만, 그럼에도 불구하고 바이러스는 10 년 넘게 분리되지 않았다. 제약 회사 Chiron 에서 일하는 마이클 호튼은 바이러스의 유전적 서열을 분리하는 데 필요한 어려운 작업을 시작했다. 호튼과 그의 동료들은 감염된 침팬지의 혈액에서 발견된 핵산으로부터 DNA 조각 모음을 만들었다. 이 조각들의 대부분은 침팬지 자체의 유전체에서 나왔지만, 연구자들은 일부가 알려지지 않은 바이러스에서 유래했을 것으로 예측했다. 간염 환자의 혈액에 바이러스에 대한 항체가 있을 것이라는 가정 하에, 연구자들은 환자 혈청을 사용하여 바이러스 단백질을 암호화하는 클론 바이러스 DNA 조각을 식별했다. 포괄적인 검색 끝에 하나의 양성 클론이 발견되었다. 추가 연구에서 이 클론이 플라비바이러스과에 속하는 새로운 RNA 바이러스에서 유래했으며, 이는 C 형 간염 바이러스로 명명되었다. 만성 간염 환자의 항체 존재는 이 바이러스가 잃어버린 병원체임을 강력히 시사했다. Figure 2. Summary of the discoveries awarded by this year’s Nobel prize. The methodical studies of transfusion-associated hepatitis by Harvey J. Alter demonstrated that an unknown virus was a common cause of chronic hepatitis. Michael Houghton used an untested strategy to isolate the genome of the new virus that was named Hepatitis C virus. Charles M. Rice provided the final evidence showing that Hepatitis C virus alone could cause hepatitis. 그림 2. 올해의 노벨상 수상자들의 발견 요약. 하비 J. 알터의 수혈 관련 간염에 대한 체계적인 연구는 알려지지 않은 바이러스가 만성 간염의 일반적인 원인임을 증명했다. 마이클 호튼은 새로운 바이러스의 유전체를 분리하기 위해 시험되지 않은 전략을 사용했으며, 이 바이러스는 C 형 간염 바이러스로 명명되었다. 찰스 M. 라이스는 C 형 간염 바이러스만으로 간염을 일으킬 수 있음을 보여주는 최종 증거를 제공했다. The discovery of Hepatitis C virus was decisive; but one essential piece of the puzzle was missing: could the virus alone cause hepatitis? To answer this question the scientists had to investigate if the cloned virus was able to replicate and cause disease. Charles M. Rice, a researcher at Washington University in St. Louis, along with other groups working with RNA viruses, noted a previously uncharacterized region in the end of the Hepatitis C virus genome that they suspected could be important for virus replication. Rice also observed genetic variations in isolated virus samples and hypothesized that some of them might hinder virus replication. Through genetic engineering, Rice generated an RNA variant of Hepatitis C virus that included the newly defined region of the viral genome and was devoid of the inactivating genetic variations. When this RNA was injected into the liver of chimpanzees, virus was detected in the blood and pathological changes resembling those seen in humans with the chronic disease were observed. This was the final proof that Hepatitis C virus alone could cause the unexplained cases of transfusion-mediated hepatitis. C 형 간염 바이러스의 발견은 결정적이었지만, 한 가지 중요한 퍼즐 조각이 빠져 있었다: 바이러스가 단독으로 간염을 일으킬 수 있는가? 이 질문에 답하기 위해 과학자들은 클론 바이러스가 복제되고 질병을 일으킬 수 있는지 조사해야 했다. 세인트루이스의 워싱턴 대학교 연구원 찰스 M. 라이스와 RNA 바이러스를 연구하는 다른 그룹은 C 형 간염 바이러스 유전체의 끝에 있는 이전에 특성화되지 않은 영역이 바이러스 복제에 중요할 수 있다고 의심했다. 라이스는 또한 분리된 바이러스 샘플에서 유전적 변이를 관찰하고, 일부 변이가 바이러스 복제를 방해할 수 있다고 가설을 세웠다. 유전 공학을 통해 라이스는 새로 정의된 바이러스 유전체 영역을 포함하고 비활성화 유전적 변이가 없는 C 형 간염 바이러스 RNA 변이를 생성했다. 이 RNA 를 침팬지의 간에 주입했을 때, 혈액에서 바이러스가 검출되었고 인간의 만성 질병에서 보이는 병리학적 변화와 유사한 변화가 관찰되었다. 이것은 C 형 간염 바이러스가 수혈 매개 간염의 설명되지 않은 사례를 일으킬 수 있다는 최종 증거였다. Significance of this Nobel Prize-awarded discovery The Nobel Laureates’ discovery of Hepatitis C virus is a landmark achievement in the ongoing battle against viral diseases (Figure 2). Thanks to their discovery, highly sensitive blood tests for the virus are now available and these have essentially eliminated post- transfusion hepatitis in many parts of the world, greatly improving global health. Their discovery also allowed the rapid development of antiviral drugs directed at hepatitis C. For the first time in history, the disease can now be cured, raising hopes of eradicating Hepatitis C virus from the world population. To achieve this goal, international e`orts facilitating blood testing and making antiviral drugs available across the globe will be required (Figure 3). 노벨상 수상 발견의 의의 노벨 수상자들의 C 형 간염 바이러스 발견은 바이러스 질병과의 지속적인 싸움에서 획기적인 성과이다 (그림 2). 이들의 발견 덕분에 이제 바이러스에 대한 고감도 혈액 검사가 가능해졌고, 이는 세계 많은 지역에서 수혈 후 간염을 본질적으로 제거하여 글로벌 건강을 크게 향상시켰다. 이들의 발견은 또한 C 형 간염을 대상으로 하는 항바이러스 약물의 신속한 개발을 가능하게 했다. 역사상 처음으로, 이 질병은 이제 치료될 수 있으며, 세계 인구에서 C 형 간염 바이러스 제거에 대한 희망을 높이고 있다. 이 목표를 달성하기 위해서는 혈액 검사 촉진과 항바이러스 약물의 전 세계적 제공을 위한 국제적인 노력이 필요할 것이다 (그림 3). Figure 3. The discoveries by the three Nobel laureates allowed the design of sensitive blood tests that have eliminated the risk of transfusion-transmitted hepatitis in a large part of the world. This breakthrough also enabled the development of antiviral drugs that can cure the disease. Hepatitis C remains a major global health concern, but the opportunity now exists to eliminate the disease. 그림 3. 세 노벨 수상자의 발견은 민감한 혈액 검사의 설계를 가능하게 했으며, 이는 세계 많은 지역에서 수혈로 인한 간염 위험을 제거했다. 이 획기적인 발견은 또한 질병을 치료할 수 있는 항바이러스 약물의 개발을 가능하게 했다. C 형 간염은 여전히 주요 글로벌 건강 문제로 남아 있지만, 이제 이 질병을 제거할 기회가 존재한다. 2021 Nobel Prize in Physiology or Medicine David Julius and Ardem Patapoutian for their discoveries of receptors for temperature and touch Summary Our ability to sense heat, cold, and touch is essential for survival. Recently, how nerve impulses are initiated to perceive temperature and pressure was discovered. David Julius used capsaicin, a pungent compound from chili peppers that induces a burning sensation, to identify a sensor in the nerve endings of skin that responds to heat. Ardem Patapoutian used pressure-sensitive cells to discover a new class of sensors that respond to mechanical stimuli in the skin and internal organs. How do we perceive the world? In the 17th century, René Descartes envisioned threads connecting different parts of the skin with the brain. In this way, a foot touching an open flame would send a mechanical signal to the brain. Later, specialized sensory neurons that register changes in our environment were discovered. Joseph Erlanger and Herbert Gasser received the Nobel Prize in 1944 for discovering different types of sensory nerve fibers that react to different stimuli. Nerve cells are highly specialized for detecting and transducing differing types of stimuli. 우리의 열, 냉기, 그리고 촉각을 감지하는 능력은 생존에 필수적입니다. 최근, 온도와 압력을 인식하기 위해 신경 자극이 어 떻게 시작되는지가 발견되었습니다. 데이비드 줄리어스는 고추에서 불타는 느낌을 유발하는 매운 화합물인 캡사이신을 사용 하여, 열에 반응하는 피부의 신경 말단에 있는 센서를 확인했습니다. 아르뎀 파타푸티안은 압력에 민감한 세포를 사용하여 피부와 내부 장기에서 기계적 자극에 반응하는 새로운 종류의 센서를 발견했습니다. 우리는 세상을 어떻게 인식할까요? 17 세기에 르네 데카르트는 피부의 여러 부분과 뇌를 연결하는 실을 상상했습니다. 이와 같은 방식으로, 발이 열린 불꽃을 만지 면 기계적인 신호가 뇌로 전달될 것이라고 생각했습니다. 이후, 환경 변화에 반응하는 특수 감각 뉴런이 발견되었습니다. 조 지프 얼랑거와 허버트 개서는 다른 자극에 반응하는 다양한 유형의 감각 신경 섬유를 발견한 공로로 1944년 노벨상을 수상 했습니다. 신경 세포는 다양한 유형의 자극을 감지하고 변환하는 데 매우 특화되어 있습니다. The science heats up David Julius at the University of California, San Francisco, saw the possibility for major advances by analyzing how capsaicin causes the burning sensation. Julius and his coworkers created a library of millions of DNA fragments of genes expressed in sensory neurons that react to pain, heat, and touch. They hypothesized that the library would include a DNA fragment encoding the protein capable of reacting to capsaicin. They expressed individual genes in cells that normally do not react to capsaicin. They found a gene able to make cells capsaicin sensitive. Further experiments found that the gene encoded a new ion channel protein that was named TRPV1. TRPV1 is a heat-sensing receptor activated at temperatures perceived as painful. TRPV1 led to discoveries of additional temperature-sensing receptors. Both Julius and Patapoutian used menthol to identify TRPM8, a receptor activated by cold. Additional related ion channels were identifie