Organic Chemistry PDF
Document Details
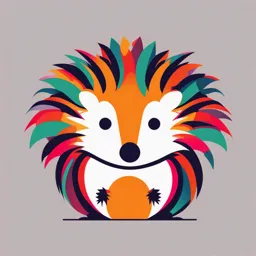
Uploaded by DependablePromethium
Ġ.F. Abela Junior College
Tags
Summary
This document provides an overview of organic chemistry, specifically focusing on the concept of hybridization (sp3, sp2, and sp1) and its implications for molecular structure and bonding.
Full Transcript
Organic Chemistry Organic chemistry can be simply defined as the study of the compounds of carbon. Organic compounds include sugars, carbohyrates and fats i.e. the compounds used by living organisms. Carbon has six electrons (atomic number of 6), meaning that it has an electron configuration of:...
Organic Chemistry Organic chemistry can be simply defined as the study of the compounds of carbon. Organic compounds include sugars, carbohyrates and fats i.e. the compounds used by living organisms. Carbon has six electrons (atomic number of 6), meaning that it has an electron configuration of: a covalent bond entails sharing of electrons between By looking at the electron configuration of carbon, one may deduce that two atoms, where each atom carbon can form 2 covalent bonds. However, this is not the case, and contributes 1 electron. carbon forms 4 bonds. Why? An orbital is the region in Carbon undergoes a process known as hybridisation. In this process, the space where there is shapes of the orbitals of the outer shell (energy level) are rearranged in maximum probability of order to form more stable bonds in the product molecule. finding an electron Carbon can undergo three types of hybridisation, depending on the reaction it is undergoing. 1 1. Sp3 hybridisation. The s orbital and the three p orbitals of the second energy level of carbon Degenerate orbitals have combine together to form 4 degenerate orbitals. These orbitals are called sp3 the same energy. hydridised orbitals. Figure 1: s and p orbitals undergoing sp3 hybridisation Orbitals contain electrons, which are negatively The four sp3 orbitals take the shape of a tetrahedron in order to mimimise charged and therefore repulsion between the electrons. Note that the largest angle between 4 repeal each other. bonds in 2D is 90ᵒ, however, in 3D, the largest angle between four bonds is 109.15ᵒ, forming a tetrahedron. Figure 2: The shape taken by sp3 hybridised carbon atom Sp3 orbitals usually bond with other hybridised orbitals or s orbitals. The bonding involves 'head-on' or collinear overlapping of these orbitals, forming what is known as a sigma ( ) bond. 2 Figure 3: Bond between a sp3 hydridised carbon atom and 4 hydrogen atoms 2. Sp2 hybridisation. The s orbital and the two p orbitals of the second energy level of carbon combine together to form 3 degenerate orbitals (1 p orbital remains unchanged). The newly formed orbitals are called sp2 hydridised orbitals. Figure 4: s and p orbitals undergoing sp2 hybridisation 3 The three newly formed sp2 orbitals are planer, with the angle between the orbitals being 120ᵒ. The p-orbital is perpendicular to the sp2 hybridised orbitals. Sp2 orbitals usually bond with other hybridised orbitals or s orbitals. The bonding involves 'head-on' or collinear overlapping of these orbitals, forming a bond. The unhybridised p-orbital undergoes 'sideways' or collateral overlapping forming a Pi ( ) bond. Thus, when carbon undergoes sp2 hybridisation it forms a double bond. The double bond is composed of two types of bonds, a sigma bond and a pi bond. Note: The Pi-bond has electron density both on top and on the bottom of the sigma-bond. Furthermore, Due to the s p fact that there are two bonds, the carbons cannot rotate relative to each other. Figure 5: Bond between two sp2 hydridised carbon atoms 3. Sp1 hybridisation. The s orbital and the one p orbital of the second energy level of carbon combine together to form 2 degenerate orbitals (2 p orbital remain unchanged). The newly formed orbitals are called sp1 hydridised orbitals. 4 Figure 6: s and p orbitals undergoing sp1 hybridisation The two newly formed sp2 orbitals are planer, with the angle between the orbitals being 180ᵒ. The p-orbitals are perpendicular to the sp1 hybridised orbitals. Sp1 orbitals usually bond with other hybridised orbitals or s orbitals. The bonding involves 'head-on' or collinear overlapping of these orbitals, forming a sigma bond. The unhybridised p-orbitals form a Pi bond. Thus, when carbon undergoes sp1 hybridisation it forms a triple bond. The triple bond is composed of two types of bonds, a sigma bond and a two pi bonds. Figure 7: Bond between two sp1 hydridised carbon atoms 5 Homologous Series A homologous series is a group of compounds with similar structural features that can be represented by a general formula and whose members differ by one -CH2- unit. You can think of an homologous series as a 'family' or group of compounds, whose chemical behaviour is very similar and whose physical properties show trends when ascending the members of the group (in terms of number of carbon atoms). Most of the times, A homologous series has a functional group, which is the part of a molecule that is responsible for the characteristic reactions of that homologous series. Each homologous series has a class name that must be distinguished from the name of the functional group that it contains. Table 1: Different Homologous Series and their functional groups 6 Table 1 (cont): Different Homologous Series and their functional groups Naming Organic compounds There are two ways which can be used to identify organic compounds: (1) Common names i.e. a particular name which was given to a particular compound. This name does not necessarily give any information on the structure of the molecule. Furthermore, one compound can have more than one common name. (2) Systematic names also referred to as IUPAC (International Union of Pure and Applied Chemistry) names. The systematic names depend on the structure of the organic molecule and are constructed using a set of simple rules. Since the systematic names give the full information on the structure of the molecule, they can become quite long for complex molecule. The next section aims to present the rules which may be used to construct the systematic names of organic molecules. 7 Naming of Organic Compounds The first two things you must identify when naming organic molecules are: (1) the number of carbons present (denoted by a prefix, as indicated in Table 2) and (2) the homologous series they pertain to (denote by a suffix, as indicated in Table 3). Table 2: Prefixes depending on the number of carbons Table 3: Suffix names of a number of functional groups Functional Group Name of Homologous Series Name as Suffix N/A Alkanes ane -C=C- Alkenes ene -C≡C- Alkynes yne -OH Alcohols ol -CHO Aldehydes al -CO Ketones one -CN Nitriles nitrile -NH2 Amines amine -COOH Carboxylic Acids oic acid -COO- Carboxylate ions oate -COCl Acid chloride Oyl chloride -CONH2 Amides amide 8 Some examples, Methane: Propane: Octane: In order to name more complex organic compounds, one must follow a set of rules, as follows: 1. Find and name the longest continuous carbon chain, that contains the functional group. 2. Identify and name groups attached to this chain. 3. Number the chain consecutively, starting at the end nearest to the functional group (or the nearest to a side branch in the case of alkanes). 4. Designate the location of each substituent group by an appropriate number and name. 5. Assemble the name, listing groups in alphabetical order using the full name. The prefixes di, tri, tetra etc., has to be used to designate several groups of the same kind, are not considered when placing groups in alphabetical order. 9 Example 1: Step 1: select longest contentious chain The longest chain has 4 carbons and the molecule has only carbon to carbon single bonds. Therefore the name of the longest contentious chain is butane Step 2: Identify and name groups attached to this chain Note on naming an alkyl a group: An alkyl group is a branch on the main chain that is composed of carbon and hydrogen only. As a prefix, we use the number of In this case, there is carbons while as a suffix we use yl. only one group, an Thus in this case, the alky group alkyl group named has 1 carbon, therefore it is a methyl methyl group. 10 Step 3: Number the chain consecutively NOT 1 2 3 4 4 3 2 1 The longest chain is numbered so that the substituent group has the lowest possible number Step 4: Designate the location of each substituent group In this case, the subsistent group is on carbon number 2, therefore we have a 2-methyl 1 2 3 4 Step 5: Assemble the name In this case we have 2-methylbutane 11 Example 2: Step 1: select longest contentious chain The longest chain has 5 carbons and the molecule has only carbon to carbon single bonds. Therefore the name of the longest contentious chain is pentane Step 2: Identify and name groups attached to this chain In this case, there are two groups. Both of these groups are alkyl group with one carbon each. Therefore these are methyl groups 12 Step 3: Number the chain consecutively 1 2 3 4 5 NOT 5 4 3 2 1 In this case, since there are two groups (which are the same), we number so that both groups have the smallest possible number. In this case 2,3 (left hand side) is smaller than 3,4 (right hand side Step 4: Designate the location of each substituent group Compounds having the same substituent group occurring more than once When the same substituent group In this case, the subsistent occurs more than once , we groups have the same introduce a prefix before the name (methyl) and are group name, as follows: on carbon number 2 and 2 groups: di 1 2 3 4 5 3, therefore we have a 2,3- 3 groups: tri dimethyl 4 groups: tetra 5 groups: penta Step 5: Assemble the name In this case we have 2,3-dimethylpentane 13 Example 3: Note on the Drawing of Organic structures: Instead of drawing all bonds in an organic molecule, we can group some of the components. For example, instead of drawing a methyl group: we can write CH3- Step 1: select longest contentious chain NOT The longest chain has 6 carbons and Note that even though both chains the molecule has only carbon to have 6 carbons, we choose the one on carbon single bonds. the left because the groups are not Therefore the name of the longest branched. contentious chain is hexane Step 2: Identify and name groups attached to this chain In this case, there are three groups. Two of these groups are alkyl group with one carbon each. Therefore these are methyl groups while the other is an alkyl group with two carbons therefore it is an ethyl group 14 Step 3: Number the chain consecutively 6 5 4 3 2 1 1 2 3 4 5 6 NOT In this case, if we start to number the carbon atoms from the right hand side or left hand side, the first substituent group will be on carbon 2. Thus in this case we number the longest chain so that the third substituent group has the smallest number. Step 4: Designate the location of each substituent group In this case, two subsistent groups have the same name (methyl) and 6 5 4 3 2 1 are on carbon number 2 and 5, while the third subsituent group has two carbons (ethyl) and is on carbon 3. Therefore we have 3-ethyl-2,5-dimethyl Step 5: Assemble the name In this case we have 3-ethyl-2,5-dimethylhexane 15 Note on the Drawing of Organic structures: Example 4: In a skeletal formula, all the hydrogen atoms are removed from carbon chains, leaving just a carbon skeleton with functional groups attached to it. If nothing is written at the junction between bonds (or at the end of a bond) it means that there is a carbon atom attached to the bond. There are enough hydrogen atoms attached to each carbon to make the total number of bonds on that carbon up to 4 (remember that carbon always have 4 bonds). In this case, the structural formula is: Step 1: select longest contentious chain The longest chain has 6 carbons and the molecule has only carbon to carbon single bonds. Therefore the name of the longest contentious chain is hexane 16 Step 2: Identify and name groups attached to this chain In this case, there are two groups. One of these groups is an alkyl group with one carbon, (methyl group) while the other is an alkyl group with two carbons (ethyl group) Step 3: Number the chain consecutively 5 1 2 6 6 4 3 NOT 1 3 4 2 5 In this case, if we start to number the carbon atoms from the right hand side or left hand side, the first substituent group will be on carbon 3. Furthermore, in this case there is no third substituent group. Thus we number by alphabetical priority. In this case e of ethyl comes before m of methyl; therefore ethyl must have the smallest number. 17 Step 4: Designate the location of each substituent group In this case, we have an ethyl group 5 1 on carbon number 3 while we have 6 4 3 a methyl group on carbon 4Therefore we have 3-ethyl-4-methyl 2 Step 5: Assemble the name In this case we have 3-ethyl-4-methylhexane Example 5: Step 1: select longest contentious chain NOT The longest chain must incorporate both carbons of the double bond. Thus in this case, we have 4 carbons (but) and the molecule has a carbon to carbon double bond (ene). We have to give the position of the double bond, in order to name the parent chain. 18 Step 2: Identify and name groups attached to this chain In this case, there is an alkyl group with two carbons (ethyl group) Step 3: Number the chain consecutively 3 2 4 2 1 3 NOT 1 4 The functional group (in this case the double bond) must have the smallest possible number Step 4: Designate the location of each substituent group In this case, we have an ethyl group 3 on carbon number 2. Therefore we 2 have 2-ethyl. 4 Note that the double bond starts on carbon 1 therefore the name of the 1 parent chain is but-1-ene Step 5: Assemble the name In this case we have 2-ethylbut-1-ene 19 Example 6: Step 1: select longest contentious chain In this case the parent chain is a cyclic molecule. In order to name it, we have to add the term cyclo before the name. Thus in this case the parent chain has six carbon atoms and carbon to carbon single bonds. Therefore it is named as cyclohexane Step 2: Identify and name groups attached to this chain In this case, there is an alkyl group with one carbon (methyl group) Step 3: Number the chain consecutively 6 Carbon 1 is the carbon to which the 5 1 functional group is attached. We continue numbering so that the nearest group has 4 2 the lowest number 3 20 Step 4: Designate the location of each substituent group In this case, we have a methyl group 6 on carbon number 2. Therefore we 5 1 have 2-methyl. 4 2 3 Step 5: Assemble the name In this case we have 2-methylcyclohexanol Drawing organic molecules in 3D. There are occasions when it is important to be able to show the precise 3-D arrangement in parts of some molecules. To do this, the bonds are shown using conventional symbols: For example, you might want to show the 3-D arrangement of the groups around the carbon which has the -OH group in butan-2-ol. Butan-2-ol has the structural formula: Using conventional bond notation, you could draw it as, for example: The only difference between these is a slight rotation of the bond between the centre two carbon atoms. 21 Isomerism. Isomers are molecules that have the same molecular formula, but have a different arrangement of the atoms in space that excludes any different arrangements which are simply due to the molecule rotating as a whole, or rotating about particular single bonds. Isomers may be subdivided into two main Groups 1. Structural Isomers, where the atoms are arranged in a completely different order; and 2. Stereo Isomers, where the atoms making up the isomers are joined up in the same order, but still manage to have a different spatial arrangement. Each of these two main groups is further subdivided into sub-classes which describe the specific type of isomerism molecules show. 22 Structural Isomerism Chain Isomers: Chain isomers have same molecular formula but differ in the order in which C-atoms are bonded to each other. These isomers arise because of the possibility of branching in carbon chains. Example: Chain Isomers of C5H12 Positional Isomers: Isomerism caused by the difference in the position of functional group in the same chain. Example: Positional Isomers of C3H7OH and 23 Functional group Isomers: Functional isomers have same molecular formula but different functional group. Example: Functional group Isomers of C2H6O Stereo Isomerism Geometric Isomers: Geometric isomerism occurs when there is restricted rotation somewhere in a molecule. Examples involve the carbon-carbon double bond. Why does Geometric Isomerism Occur? Let’s take 1,2-dichloroethane, as an example. In this molecule, there are only carbon to carbon single bonds, therefore there is unrestricted rotation. Considering the graphical representations of 1,2-dichloroethane below, one notes that these two models represent exactly the same molecule. You can get from one to the other just by twisting around the carbon-carbon single bond. These two structures represent the same molecule and therefore are not isomers. 24 But what happens if you have a carbon-carbon double bond - as in 1,2-dichloroethene? The carbon-carbon double bond won't rotate and so you would have to take the molecule to pieces in order to convert one structure into the other one. That is a simple test for isomers. If you have to take a molecule to pieces to convert it into another one, then you've got isomers. If you merely have to twist it a bit (rotation about a single bond), then you haven't! Drawing structural formulae for the last pair of models gives two possible isomers: 1. In one, the two chlorine atoms are locked on opposite sides of the double bond. This is known as the trans isomer. (trans: from latin meaning "across" - as in transatlantic). 2. In the other, the two chlorine atoms are locked on the same side of the double bond. This is know as the cis isomer. (cis : from latin meaning "on this side") 25 What needs to be attached to the carbon-carbon double bond? Think about this case: Although we've swapped the right-hand groups around, these are still the same molecule. To get from one to the other, all you would have to do is to turn the whole model over. You won't have geometric isomers if there are two groups the same on one end of the bond - in this case, the two pink groups on the left-hand end. So there must be two different groups on the left-hand carbon and two different groups on the right-hand one. 26 Stereo Isomers: Optical isomers are molecules that differ three-dimensionally by the placement of substituents around one or more atoms in a molecule Origin of Optical Isomers The examples of organic optical isomers contain a carbon atom joined to four different groups. These molecules are said to be chiral. Referring to the figure below, these two models each have the same groups joined to the central carbon atom, but still manage to be different: Obviously as they are drawn, the orange and blue groups aren't aligned the same way. Could you get them to align by rotating one of the molecules? The next diagram shows what happens if you rotate molecule B. 27 They still are not the same - and there is no way that you can rotate them so that they look exactly the same. These are isomers of each other (they are called enantiomers). They are described as being non-superimposable What happens if two of the groups attached to the central carbon atom are the same? The next diagram shows this possibility. The two models are aligned exactly as before, but the orange group has been replaced by another pink one. Rotating molecule B this time shows that it is exactly the same as molecule A. You only get optical isomers if all four groups attached to the central carbon are different. The relationship between the enantiomers One of the enantiomers is simply a non-superimposable mirror image of the other one. In other words, if one isomer looked in a mirror, what it would see is the other one. The two isomers (the original one and its mirror image) have a different spatial arrangement, and so cannot be superimposed on each other. 28 The asymmetric carbon atom in a compound (the one with four different groups attached) is often shown by a star. Optical isomers are named like this because of their effect on plane polarized light. A solution of one enantiomer rotates the plane of polarisation in a clockwise direction. This enantiomer is known as the (+) form or (d) form. For example, one of the optical isomers (enantiomers) of the amino acid alanine is known as (+)alanine. A solution of the other enantiomer rotates the plane of polarisation in an anti- clockwise direction. This enantiomer is known as the (-) form or (l) form. So the other enantiomer of alanine is known as or (-)alanine. 29 Alkanes Alkanes are hydrocarbons (composed of carbon and hydrogen only) having carbon to carbon single bonds (i.e. the carbons of alkanes are sp3 hybridised). Alkanes have the general formula CnH2n+2 Intermolecular attractive forces are Van der Waals. Thus Alkanes are non polar (alkanes do not dissolve in water) Physical Properties of Alkanes For un-branced molecules, the larger the number of carbons, the higher the boiling point. This is due to the fact that the larger the number or carbons the stronger the Van der Waals interactions. The boiling temperature of an unbranched molecule is higher than that of its branched isomer. Unbranched molecules approach one another more closely as a result, Van der Waals forces are stronger 30 Chemical Properties of Alkanes Alkanes are highly unreactive due to the fact that they lack a functional group. They are composed of carbon to carbon and carbon to hydrogen covalent bonds which are very strong bonds. In order for Alkanes to react, high energy environments must be present, such as high heat or U.V. light 31 Alkenes Alkanes are hydrocarbons having at least one carbon to carbon double bond. Alkenes have the general formula CnH2n Intermolecular attractive forces are Van der Waals since molecules are non polar (alkenes do not dissolve in water) Physical Properties of Alkanes The boiling point of each alkene is very similar to that of the alkanes with the same number of carbon atoms. Chemical Properties of Alkanes Alkenes undergo electrophilic addition reactions An electrophile is a species which is attracted to electron-rich regions. Since it is attracted to a negative region, an electrophile must be something which carries either a full positive charge, or has a slight positive charge on it. An example of an addition reaction is as follows: 32 How does it work.... In the case that Y is more electronegative than X, the electrons in the X-Y bond will be Electronegativity may be defined as attracted towards Y. This means that Y will the ability of an atom to attract have a partial negative charge (δ-) while X electrons towards it when present in a covalent bond. will have a partial positive charge (δ+). Thus in this case, the electron rich п-bond will be attracted towards δ+ X. This will result in the breaking of the double bond and the formation of a new bond between one carbon of the double and X. The other carbon of the double bond would have effectively lost an electron and therefore will have a positive charge. Furthermore, the bond between X and Y will break, with both electrons moving to Y. Therefore Y will have a negative charge. 33 The Negative charge on Y will be then attracted to the Positive change on the carbon and a new bond will be formed. This process may be summerised through the reaction mechanism, which is shown below. In this case, the arrows indicate the movement of two electrons from the tail of the arrow to the head of the arrow. 34 Alkynes Alkynes are hydrocarbons having at least one carbon to carbon triple bond. Alkynes have the general formula CnH2n-2 Intermolecular attractive forces are Van der Waals since molecules are non polar (alkenes do not dissolve in water) Chemical Properties of Alkynes As in the case of alkenes, alkynes undergo electrophilic addition reactions. Examples of the addition reactions alkynes undergo is given below. 35 Alcohols The functional group of alcohols is –OH. Alcohols have a general formula of CnH2n+1OH The first few alcohols (C1 to C4) are soluble in water because they can hydrogen bond with water molecules. Larger alcohols become less soluble due to the increase of the alkyl group which is hydrophobic. Alcohols are grouped into three categories. These are: 1. Primary (1ᵒ) alcohols: In this class of alcohols, the hydroxyl carbon is bounded to one carbon atom, for example, butan-1-ol. 2. Secondary (2ᵒ) alcohols: In this class of alcohols, the hydroxyl carbon is bound to two other carbons, for example, butan-2-ol. 3. Tertiary (3ᵒ) alcohols: In this class of alcohols, the hydroxyl carbon is bound to three other carbons, for example, 2-methyl-propan-2-ol. 36 Note that the boiling temperature of a 1ᵒ alcohol is higher than its isomeric 2ᵒ alcohol which in turn is still higher than that of a 3ᵒ alcohol. The reason is that Van der Waals attractive forces are most prominent between unbranched alkyl groups. Chemical Properties of Alcohols Note that some reactions of alcohols will proceed differently for 1ᵒ,2ᵒ and 3ᵒ alcohols, for example, oxidation of alcohols. Primary alcohols undergo oxidation reactions to form aldehydes (which in turn can be oxydised to a carboxylic acid). Secondary alcohols undergo oxidation reactions to form Ketones whilst tertiary alcohols do not get oxidised. Alcohols can also react with carboxylic acids to form esters. For example: 37 Notes on esterification reactions: Esterification reactions are equilibrium reactions. Hydrogen ions catalyse both the forward process catalyse as well the reverse one. One of the oxygens in the ester was initially present in the alcohol molecule 38