Org-Chem-Unit-6 PDF
Document Details
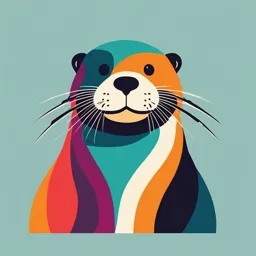
Uploaded by PreciousAestheticism
Cagayan State University
Tags
Summary
This document provides an overview of organic chemistry reactions, specifically focusing on the various types of reactions involving alkanes, alkyl halides, alkynes, and alkenes. The content includes topics such as oxidation, reduction, combustion reactions, and different types of reactions. It also includes problems and examples for classification and identification related to these reactions.
Full Transcript
Reaction of Alkanes, Alkyl Halides, Alkynes, and Alkenes Reactions of Alkanes Oxidation and Reduction of Alkanes Oxidation - Oxidation results in an increase in the number of C– Z bonds - Oxidation results in a decrease in the number of C– H bonds. Reduction - Reduction results in a decrease...
Reaction of Alkanes, Alkyl Halides, Alkynes, and Alkenes Reactions of Alkanes Oxidation and Reduction of Alkanes Oxidation - Oxidation results in an increase in the number of C– Z bonds - Oxidation results in a decrease in the number of C– H bonds. Reduction - Reduction results in a decrease in the number of C– Z bonds - Reduction results in an increase in the number of C– H bonds. Problems Determine whether the organic compound is oxidized or reduced in each transformation. Problems Classify each transformation as an oxidation, reduction, or neither. Combustion of Alkanes Combustion - Alkanes undergo combustion when they burn in the presence of oxygen to form CO2 and H2O. - Every C–H and C–C bond in the starting material is converted to a C–O bond in the product. Problems What are the products of each combustion reaction Reactions of Alkyl Halides Substitution Reaction General Features of Nucleophilic Substitution Three components in nucleophilic substitution reaction: 𝑹—An alkyl group 𝑅 containing an sp3 hybridized carbon bonded to X. 𝑿—An atom X (or a group of atoms) called a leaving group, which is able to accept the electron density in the 𝐶 − 𝑋 bond. The most common leaving groups are halide anions (𝑋 − ), but 𝐻2 𝑂(from 𝑅𝑂𝐻2+ ) and 𝑁2 (from 𝑅𝑁2+ ) are also encountered. ∶ 𝑵𝒖− —A nucleophile that contain a lone pair or a 𝜋 bond but not necessarily a negative charge. Nucleophilic Substitution Reactions Nucleophilic substitutions are Lewis acid–base reactions. The nucleophile donates its electron pair, the alkyl halide (Lewis acid) accepts it, and the C – X bond is heterolytically cleaved. Curved arrow notation can be used to show the movement of electron pairs. Examples Nucleophilic Substitution Reactions Negatively charged nucleophiles like – 𝑂𝐻 and – 𝑆𝐻 are used as salts with 𝐿𝑖 + , 𝑁𝑎+ , or 𝐾 + counterions to balance charge. When a neutral nucleophile is used, the substitution product bears a positive charge. Note that all atoms originally bonded to the nucleophile stay bonded to it after substitution occurs. When the substitution product bears a positive charge and also contains a proton bonded to O or N, the initial substitution product readily loses a proton in a Brønsted–Lowry acid–base reaction, forming a neutral product. Nucleophilic Substitution Reactions To draw any nucleophilic substitution product regardless of the identity or charge of the nucleophile: Step. Find the sp3 hybridized carbon with the leaving group. Step. Identify the nucleophile, the species with a lone pair or π bond. Step. Substitute the nucleophile for the leaving group and assign charges (if necessary) to any atom that is involved in bond breaking or bond formation. Problems: Identify the nucleophile and leaving group and draw the products of each reaction. Problems: Identify the nucleophile and leaving group and draw the products of each reaction. Problems: Draw the product of nucleophilic substitution with each neutral nucleophile. When the initial substitution product can lose a proton to form a neutral product, draw that product as well. Leaving Group In a nucleophilic substitution reaction of 𝑅 − 𝑋, the C − 𝑋 bond is heterolytically cleaved, and the leaving group departs with the electron pair in that bond, forming 𝑋:−. The more stable the leaving group 𝑋:− , the more able it is to accept an electron pair. In comparing two leaving groups, the better leaving group is the weaker base. Periodic Trends in Leaving Group Leaving Group All good leaving groups are weak bases with strong conjugate acids having low pKa values. Thus, all halide anions except 𝐹 − are good leaving groups because their conjugate acids (HCl, HBr, and HI) have low pKa values. Problems: Which is the better leaving group in each pair? Problems: Which molecules contain good leaving groups? Nucleophilic Substitution Reaction The direction of equilibrium can be predicted by comparing the basicity of the nucleophile and the leaving group. Equilibrium favors the products of nucleophilic substitution when the leaving group is a weaker base than the nucleophile. Example Will the following substitution reaction favor formation of the products? Solution The stronger the conjugate acid, the weaker the base, and the better the leaving group. Answers: The reaction favors the products. Problems: Does the equilibrium favor the reactants or products in each substitution reaction? Problems: Should it be possible to convert 𝐶𝐻3 𝐶𝐻2 𝐶𝐻2 OH to 𝐶𝐻3 𝐶𝐻2 𝐶𝐻2 Cl by a nucleophilic substitution reaction with NaCl? Explain why or why not. The Nucleophile Nucleophiles and bases are structurally similar: both have a lone pair or a 𝜋 bond. They differ in what they attack. Base is used to mean Brønsted–Lowry base that reacts with protons. Nucleophile is used to mean a Lewis base that reacts with electrophiles (electron-deficient) other than protons, usually carbons. Nucleophilicity Versus Basicity Nucleophilicity parallels basicity in three instances: For two nucleophiles with the same nucleophilic atom, the stronger base is the stronger nucleophile. A negatively charged nucleophile is always stronger than its conjugate acid. Right-to-left across a row of the periodic table, nucleophilicity increases as basicity increases. Stearic Effects and Nucleophilicity Nucleophilicity does not parallel basicity when steric hindrance becomes important. All steric effects arise because two atoms cannot occupy the same space. Steric strain is an increase in energy when big groups (occupying a large volume) are forced close to each other. Steric hindrance is a decrease in reactivity resulting from the presence of bulky groups at the site of a reaction. Stearic Effects and Nucleophilicity Nucleophilicity does not parallel basicity when steric hindrance becomes important. All steric effects arise because two atoms cannot occupy the same space. Steric hindrance decreases nucleophilicity but not basicity. Because bases pull off small, easily accessible protons, they are unaffected by steric hindrance. Nucleophiles, on the other hand, must attack a crowded tetrahedral carbon, so bulky groups decrease reactivity. Sterically hindered bases that are poor nucleophiles are called − nonnucleophilic bases. Potassium tert-butoxide 𝐾 + 𝑂𝐶 𝐶𝐻3 3 is a strong, nonnucleophilic base. Comparing Nucleophiles of Different Size – Solvent Effects Atoms vary greatly in size down a column of the periodic table, and in this case, nucleophilicity depends on the solvent used in a substitution reaction. Because substitution reactions involve polar starting materials, polar solvents are used to dissolve them. A. Polar Protic Solvents They are capable of intermolecular hydrogen bonding, because they contain an O – H or N – H bond. Polar protic solvents solvate both cations and anions well. ▪ Cations are solvated by ion–dipole interactions. ▪ Anions are solvated by hydrogen bonding. B. Polar Aprotic Solvents Polar aprotic solvents also exhibit dipole–dipole interactions, but they have no 𝑂 − 𝐻 or 𝑁 − 𝐻 bond so they are incapable of hydrogen bonding. Polar aprotic solvents solvate only cations well. ▪ Cations are solvated by ion–dipole interactions. ▪ Anions are not well solvated because the solvent cannot hydrogen bond to them. Possible Mechanisms for Nucleophilic Substitution What nucleophile is needed to convert 𝐶𝐻3 2 𝐶𝐻𝐶𝐻2 𝐶𝐻2 − 𝐵𝑟 to each product? Possible Mechanisms for Nucleophilic Substitution Bond breaking and bond making occur at the same time. [2.1] Bond breaking occurs before bond making. [2.2] Bond making occurs before bond breaking. Mechanisms for Nucleophilic Substitution SN2 Mechanism (substitution nucleophilic bimolecular) Bimolecular reaction with a one- step mechanism in which the 𝐶 − 𝑋 bond is broken as the 𝐶 − 𝑁𝑢 bond is formed. SN1 Mechanism (substitution nucleophilic unimolecular) Two-step mechanism in which the rate-determining step involves the alkyl halide only. The SN2 Mechanism Kinetics Energy Diagram 𝑟𝑎𝑡𝑒 = 𝑘 𝐶𝐻3 𝐵𝑟 [𝐶𝐻3 𝐶𝑂𝑂− ] One-step Mechanism The 𝐶 − 𝐵𝑟 bond breaks as the 𝐶 − 𝑂 bond forms. Stereochemistry of the SN2 Reaction Frontside attack The nucleophile approaches from the same side as the leaving group. Backside attack The nucleophile approaches from the side opposite the leaving group. Stereochemistry of the SN2 Reaction The products of frontside and backside attack are different compounds. A and B are stereoisomers that are nonsuperimposable – they are enantiomers. All 𝑆𝑁 2 reactions process with backside attack of the nucleophile, resulting in inversion of configuration at a stereogenic center. Stereochemistry of the SN2 Reaction All 𝑆𝑁 2 reactions process with backside attack of the nucleophile, resulting in inversion of configuration at a stereogenic center. Stereochemistry of the SN2 Reaction Example Draw the product (including stereochemistry) of the following 𝑆𝑁 2 reaction. Solution Problems Draw the product of each 𝑆𝑁 2 reaction and indicate stereochemistry. The Identity of R Group As the number of 𝑅 groups on the carbon with the leaving group increases, the rate of an 𝑆𝑁 2 reaction decreases. Methyl and 1° alkyl halides undergo 𝑆𝑁 2 reactions with ease. 2° Alkyl halides react more slowly. 3° Alkyl halides do not undergo 𝑆𝑁 2 reactions. The Identity of R Group The order of reactivity can be explained by steric effects. As small 𝐻 atoms are replaced by larger alkyl groups, steric hindrance caused by bulky R groups makes nucleophilic attack from the back side more difficult, slowing the reaction rate. Crowding around the central carbon atom increases as H atoms are successively replaced by R groups. Steric effects in the 𝑺𝑵 𝟐 reaction Two energy diagrams depicting the effect of steric hindrance in 𝑆𝑁 2 reactions: Increasing the number of R groups on the carbon with the leaving group increases crowding in the transition state, decreasing the rate of an 𝑆𝑁 2 reaction. The 𝑆𝑁 2 reaction is fastest with unhindered halides. Problems Which compound in each pair undergoes a faster 𝑆𝑁 2 reaction? SN2 Mechanism (substitution nucleophilic bimolecular) The SN1 Mechanism Kinetics 𝑟𝑎𝑡𝑒 = 𝑘 𝐶𝐻3 3 𝐶𝐵𝑟 Energy Diagram Two-step Mechanism The 𝐶 − 𝐵𝑟 bond is broken. The 𝐶 − 𝑂 bond is formed. Stereochemistry of the SN1 Reaction A carbocation (with three groups around 𝐶) is 𝑠𝑝2 hybridized and trigonal planar, and contains a vacant p orbital extending above and below the plane. B and C are stereoisomers that are not superimposable—they are enantiomers. Because there is no preference for nucleophilic attack from either direction, an equal amount of the two enantiomers is formed—a racemic mixture. ▪ Racemization is the formation of equal amounts of two enantiomeric products from a single starting material. ▪ 𝑆𝑁 1 reactions proceed with racemization at a single stereogenic center. Two example of racemization in the 𝑆𝑁 1 reaction ▪ Nucleophilic substitution of each starting material by an 𝑆𝑁 1 mechanism forms a racemic mixture of two products. ▪ With 𝐻2 𝑂 , a neutral nucleophile, the initial product of nucleophilic substitution (𝑅𝑂𝐻2+ ) loses a proton to form the final neutral product 𝑅𝑂𝐻. Problems Draw the products (including stereochemistry) of the following 𝑆𝑁 1 reaction. Solution Problems Draw the products (including stereochemistry) of the following 𝑆𝑁 1 reaction. Solution Problems Draw the products of each 𝑆𝑁 1 reaction and indicate the stereochemistry of any stereogenic centers The Identity of the R Group As the number of 𝑅 groups on the carbon with the leaving group increases, the rate of an 𝑆𝑁 1 reaction increases. 3° Alkyl halides undergo 𝑆𝑁 1 reaction rapidly. 2° Alkyl halides react more slowly. Methyl and 1° alkyl halides do not undergo 𝑆𝑁 1 reactions. Carbocation Stability Carbocations are classified as primary (1°), secondary (2°), or tertiary (3°) by the number of 𝑅 groups bonded to the charged carbon atom. As the number of R groups on the positively charged carbon atom increases, the stability of the carbocation increases. Problems Classify each carbocation as 1°, 2°, or 3°. Problems Rank the following carbocations in order of increasing stability. SN1 vs SN2 Mechanism : Alkyl Halide Four factors are examined to know whether a reaction occurs by the 𝑆𝑁 1 or 𝑆𝑁 2 mechanism: ▪ The alkyl halide – 𝐶𝐻3 𝑋, 𝑅𝐶𝐻2 𝑋, 𝑅2 𝐶𝐻𝑋, or 𝑅3 𝐶𝑋 ▪ The nucleophile – strong or weak ▪ The leaving group – good or poor ▪ The solvent – protic or aprotic ▪ Methyl and 1° halides (𝐶𝐻3 𝑋 and 𝑅𝐶𝐻2 𝑋) undergo 𝑆𝑁 2 reactions only. ▪ 3° Alkyl halides (𝑅3 𝐶𝑋) undergo 𝑆𝑁 1 reactions only. ▪ 2° Alkyl halides (𝑅2 𝐶𝐻𝑋) undergo both 𝑆𝑁 1 and 𝑆𝑁 2 reactions. Others factors determine the mechanism. Problems What is the likely mechanism of nucleophilic substitution for each alkyl halide? SN1 vs SN2 Mechanism : Nucleophile For 𝑆𝑁 1 mechanism: The rate of reaction is unaffected by the identity of the nucleophile because the nucleophile does not appear in the rate equation. 𝑟𝑎𝑡𝑒 = 𝑘 𝑅𝑋 For 𝑆𝑁 2 mechanism: The rate of reaction is affected by the identity of the nucleophile because the nucleophile appear in the rate equation. 𝑟𝑎𝑡𝑒 = 𝑘 𝑅𝑋 : 𝑁𝑢− Strong nucleophiles present in high concentration favor 𝑆𝑁 2 reactions. Weak nucleophiles favor 𝑆𝑁 1 reactions by decreasing the rate of any competing 𝑆𝑁 2 reaction. The identity of 𝑅𝑋 and the mechanism of nucleophilic substitution: The most common nucleophiles in 𝑆𝑁 2 reactions bear a net negative charge. The most common nucleophiles in 𝑆𝑁 1 reactions are weak nucleophiles such as 𝐻2 𝑂 and 𝑅𝑂𝐻. Problems For each alkyl halide and nucleophile: Draw the product of nucleophilic substitution; determine the likely mechanism (𝑆𝑁 1 or 𝑆𝑁 2) for each reaction. Problems Draw the products (including stereochemistry) for each reaction. SN1 vs SN2 Mechanism : Leaving Group A better leaving group increases the rate of both 𝑆𝑁 1 and 𝑆𝑁 2 reactions. The better the leaving group, the more willing it is to accept the electron pair in the 𝐶 − 𝑋 bond, and the faster the reaction. Trends of reactivity of alkyl halides for the 𝑆𝑁 1 and 𝑆𝑁 2 mechanisms: SN1 vs SN2 Mechanism : Solvent Polar protic solvents and polar aprotic solvents affect the rates of 𝑆𝑁 1 and 𝑆𝑁 2 reactions differently. ▪ Polar protic solvents are especially good for 𝑆𝑁 1 reactions. ▪ Polar aprotic solvents are especially good for 𝑆𝑁 2 reactions. Problems Which solvents favor 𝑆𝑁 1 reactions and which favor 𝑆𝑁 2 reactions? Problems For each reaction, use the identity of the alkyl halide and nucleophile to determine which substitution mechanism occurs. Then determine which solvent affords the faster reaction. Example Determine the mechanism of nucleophilic substitution for each reaction. Include the stereochemistry at all stereogenic centers. Vinyl Halides and Aryl Halides 𝑺𝑵 𝟏 and 𝑺𝑵 𝟐 reactions occur only at 𝒔𝒑𝟑 hybridized carbon atoms. The vinyl halides and aryl halides, have a halogen atom bonded to an 𝑠𝑝2 hybridized 𝐶, do not undergo nucleophilic substitution by either the 𝑆𝑁 1 or 𝑆𝑁 2 mechanism. Vinyl halides do not undergo 𝑆𝑁 1 reactions because heterolysis of the 𝐶 − 𝑋 bond would form a highly unstable vinyl carbocation. Because this carbocation has only two groups around the positively charged carbon, it is sp hybridized. Elimination Reaction General Features of Elimination Reaction Elimination reactions involve loss of elements from the starting material to form a new 𝜋 bond in the product. Removal of the elements of 𝐻𝑋 , called dehydrohalogenation, is one of the most common methods to introduce a 𝜋 bond and prepare an alkene. General Features of Elimination Reaction Dehydrohalogenation is an example of 𝜷 elimination, because it involves loss of elements from two adjacent atoms: the 𝜶 carbon bonded to the leaving group X, and the 𝜷 carbon adjacent to it. ▪ The base (𝐵:) removes a proton on the 𝛽 carbon, thus forming 𝐻 − 𝐵 +. ▪ The electron pair in the 𝛽 𝐶 − 𝐻 bond forms the new 𝜋 bond between the 𝛼 and 𝛽 carbons. ▪ The electron pair in the 𝐶 − 𝑋 bond ends up on halogen, forming the leaving group ∶ 𝑋 −. General Features of Elimination Reaction The most common bases used in elimination reactions are negatively charged oxygen compounds such as −𝑂𝐻 and its alkyl derivatives, −𝑂𝑅, called alkoxides. To draw any product of dehydrohalogenation: ▪ Find the 𝛼 carbon – the 𝑠𝑝3 hybridized carbon bonded to the leaving group. ▪ Identify all 𝛽 carbons with 𝐻 atoms. ▪ Remove the elements of 𝐻 and 𝑋 from the 𝛼 and 𝛽 carbons and form a 𝜋 bond General Features of Elimination Reaction Example Problems Label the α and β carbons in each alkyl halide. Draw all possible elimination products formed when each alkyl halide is treated with + − 𝐾 𝑂𝐶 𝐶𝐻3 3. E2 Mechanism of Elimination (Biomolecular elimination) Kinetics Energy Diagram 𝑅𝑎𝑡𝑒 = 𝑘 𝐶𝐻3 3 𝐶𝐵𝑟 𝑂𝐻 − One-step Mechanism Problems Use curved arrows to show the movement of electrons in the following E2 mechanism. Draw the structure of the transition state. The Base The base appears in the rate equation, so the rate of the 𝐸2 reaction increases as the strength of the base increases. E2 reactions are generally run with strong, negatively charged bases like −𝑂𝐻 and – 𝑂𝑅. The Leaving Group Because the bond to the leaving group is partially broken in the transition state, the better the leaving group the faster the 𝐸2 reaction. The Solvent Polar aprotic solvents increase the rate of 𝐸2 reactions. The Identity of the Alkyl Halide ▪ The 𝑆𝑁 2 and 𝐸2 mechanisms differ in how the R group affects the reaction rate. ▪ As the number of 𝑅 groups on the carbon with the leaving group increases, the rate of the E2 reaction increases. In the transition state, the double bond is partially formed, so increasing the stability of the double bond with alkyl substituents stabilizes the transition state (i.e., it lowers 𝐸𝑎 ), which increases the rate of the reaction. Increasing the number of R groups on the carbon with the leaving group forms more highly substituted, more stable alkenes in E2 reactions. The Identity of the Alkyl Halide Example Problems Rank the alkyl halides in each group in order of increasing reactivity in an E2 reaction. E2 Mechanism of Elimination (Biomolecular elimination) E1 Mechanism of Elimination (Biomolecular elimination) Kinetics 𝑅𝑎𝑡𝑒 = 𝑘 𝐶𝐻3 3 𝐶𝐼 Energy Diagram Two-step Mechanism The 𝐶 − 𝐵𝑟 bond is broken. The 𝐶 − 𝐻 bond is cleaved and the 𝜋 bond is formed. E1 Mechanism of Elimination (Biomolecular elimination) The E1 and E2 mechanisms both involve the same number of bonds broken and formed. The only difference is the timing. ▪ In an E1 reaction, the leaving group comes off before the a proton is removed, and the reaction occurs in two steps. ▪ In an E2 reaction, the leaving group comes off as the a proton is removed, and the reaction occurs in one step. Problem Draw an E1 mechanism for the following reaction. Draw the structure of the transition state for each step. Other Characteristics of E1 Reactions The rate of an 𝐸1reaction increases as the number of R groups on the carbon with the leaving group increases. Because the base does not appear in the rate equation, weak bases favor E1 reactions. ▪ Strong bases like –OH and –OR favor E2 reactions, whereas weaker bases like H2O and ROH favor E1 reactions. Other Characteristics of E1 Reactions E1 reactions are regioselective, favoring formation of the more substituted, more stable alkene. Example E1 Mechanism of Elimination (Unimolecular elimination) 𝑺𝑵 𝟏 and 𝑬𝟏 Reactions ▪ In an 𝑆𝑁 1 reaction, a nucleophile attacks the carbocation, forming a substitution product. ▪ In an 𝐸1 reaction, a base removes a proton, forming a new o bond. 𝑺𝑵 𝟏 and 𝑬𝟏 Reactions Example Draw the 𝑆𝑁 1 and 𝐸1 products formed in the reaction of 𝐶𝐻3 3 𝐶𝐵𝑟 with 𝐻2 𝑂. Solution Problems Draw the 𝑆𝑁 1 and 𝐸1 products of each reaction. E1 vs E2 Mechanism : Problem Which mechanism, 𝐸1 or 𝐸2, will occur in each reaction. READING ASSIGNMENT: When Is the Reaction SN1, SN2, E1, or E2? (Determining whether an alkyl halides reacts by an SN1, SN2, E1, or E2 mechanism. Reference: Smith, JG. (2010) Organic Chemistry 3rd Ed (Chapter 8 p. 300-303) E2 Reactions and Alkyne Synthesis A single elimination reaction produces the π bond of an alkene. Two consecutive elimination reactions produce the two π bonds of an alkyne. Alkynes are prepared by two successive dehydrohalogenation reactions. Two elimination reactions are needed to remove two moles of HX from a dihalide as substrate. Two different starting materials can be used. ▪ A vicinal dihalide has two X atoms on adjacent carbon atoms. ▪ A geminal dihalide has two X atoms on the same carbon atom. E2 Reactions and Alkyne Synthesis Illustration E2 Reactions and Alkyne Synthesis Example Problem Draw the alkynes formed in each reaction. Two equivalents of each base are used. Reactions of Alkenes Elimination Reaction Alkenes – Products of Elimination Reactions Alkenes are form from the elimination reaction of alkyl halide. Bonding in a Carbon-Carbon Double Bond The 𝜎 bond, formed by end-on overlap of the two 𝑠𝑝2 hybrid orbitals, lies in the plane of the molecule. The 𝜋 bond, formed by side-by-side overlap of two 2𝑝 orbitals, lies perpendicular to the plane of the molecule. The o bond is formed during elimination. Alkenes – Products of Elimination Reactions Alkenes are classified according to the number of carbon atoms bonded to the carbons of the double bond. Examples Restricted Rotation in Alkenes In both cis and trans isomers, a ring or a double bond restricts motion, preventing the rotation of a group from one side of the ring or double bond to the other. Restricted Rotation in Alkenes Whenever the two groups on each end of a carbon–carbon double bond are different from each other, two diastereomers are possible. Problems For which alkenes are stereoisomers possible? Stability of Alkenes The stability of an alkene increases, moreover, as the number of 𝑅 groups bonded to the double bond carbons increases. 𝑅 groups increase the stability of an alkene because 𝑅 groups are 𝑠𝑝3 hybridized, while 𝐶 atoms of the double bond are 𝑠𝑝2 hybridized. The higher the percent s-character, the more readily an atom accepts electron density. Thus, 𝑠𝑝2 hybridized carbon atoms are more able to accept electron density and 𝑠𝑝3 hybridized carbon atoms are more able to donate electron density. As a result, increasing the number of electron-donating R groups on a carbon atom able to accept electron density makes the alkene more stable. Stability of Alkenes Trans alkenes are generally more stable than cis alkenes because the groups bonded to the double bond carbons are farther apart, reducing steric interactions. ▪ Trans alkenes are more stable than cis alkenes because they have fewer steric interactions. ▪ Increasing alkyl substitution stabilizes an alkene by an electron-donating inductive effect. Problems Which alkene in each pair is more stable? The Zaitsev Rule The major product in a elimination has the more substituted double bond. A reaction is regioselective when it yields predominantly or exclusively one constitutional isomer when more than one is possible. The 𝐸2 reaction is regioselective because the more substituted alkene predominates. The Zaitsev Rule Examples The Zaitsev Rule Examples When a mixture of stereoisomers is possible from dehydrohalogenation, the major product is the more stable stereoisomer The Zaitsev Rule Example A reaction is stereoselective when it forms predominantly or exclusively one stereoisomer when two or more are possible. The 𝐸2 reaction is stereoselective because one stereoisomer is formed preferentially. The Zaitsev Rule Example Predict the major product in the following 𝐸2 reaction. Solution Problems What alkenes are formed from each alkyl halide by an E2 reaction? Use the Zaitsev rule to predict the major product. Problem What alkenes are formed from each alkyl halide by an E1 reaction? Use the Zaitsev rule to predict the major product. Dehydration ▪ The acid-catalyzed dehydration of alcohols with 𝐻2 𝑆𝑂4 or 𝑇𝑠𝑂𝐻 yields alkenes via an 𝐸1 mechanism for 2° and 3° alcohols and 𝐸2 mechanism for 1° alcohols. Illustration ▪ Dehydration can also be carried out with 𝑃𝑂𝐶𝑙3 and pyridine by an 𝐸2 mechanism. Illustration These elimination reactions are stereoselective and regioselective, so most stable alkene is usually formed as the major product. Problem Draw the products of each elimination reaction. Addition Reaction Introduction to Addition Reactions In addition reaction, the 𝜋 bond is broken and two new 𝜎 bonds are formed. Alkenes are electron rich. The electron density of the π bond is concentrated above and below the plane of the molecule, making the 𝜋 bond more exposed than the 𝜎 bond. ▪ Every reaction of alkenes involves addition: the o bond is always broken. ▪ Because alkenes are electron rich, simple alkenes do not react with nucleophiles or bases, reagents that are themselves electron rich. Alkenes react with electrophiles. Introduction to Addition Reactions Because the carbon atoms of a double bond are both trigonal planar, the elements of X and Y can be added to them from the same side or from opposite sides. ▪ Syn addition takes place when both X and Y are added from the same side. ▪ Anti addition takes place when X and Y are added from opposite sides. Reactions of Alkenes Hydrohalogenation – Electrophilic Addition of 𝑯𝑿 ▪ It is the addition of hydrogen halides 𝐻𝑋 (𝑋 = 𝐶𝑙, 𝐵𝑟, 𝐼) to alkenes to form alkyl halides. ▪ It is the reverse of the dehydrohalogenation of an alkyl halide to form an alkene. Two bonds are broken, the weak 𝜋 bond of the alkene and the 𝐻𝑋 bond, and two new 𝜎 bonds are formed. To draw the products of an addition reaction: Illustration ▪ Locate the 𝐶 − 𝐶 double bond. ▪ Identify the 𝜎 bond of the reagent that breaks—namely, the 𝐻 − 𝑋 bond in hydro halogenation. ▪ Break the 𝜋 bond of the alkene and the 𝜎 bond of the reagent, and form two new 𝜎 bonds to the C atoms of the double bond. Hydrohalogenation – Electrophilic Addition of 𝑯𝑿 The mechanism of electrophilic addition of 𝐻𝑋 consists of two successive Lewis acid- base reactions: Step Addition of 𝐻 + to form a carbocation. Step Nucleophilic attack of 𝑋 –. Hydrohalogenation – Electrophilic Addition of 𝑯𝑿 Energy diagram for the reaction of 𝐶𝐻3 𝐶𝐻 = 𝐶𝐻𝐶𝐻3 with 𝐻𝐵𝑟 is given below. Markonikov’s Rule In the addition of 𝐻𝑋 to an unsymmetrical alkene, the 𝐻 atom bonds to the less substituted carbon atom, the carbon that has more 𝐻 atoms to begin with. Example The basis of Markonikov’s rule is the formation of a carbocation in the rate- determining step of the mechanism. There are two possible paths fort the first step, depending on which carbon atoms of the double bond forms the new bond to hydrogen. Example Draw a stepwise mechanism for the following reaction. Problem What product is formed when each alkene is treated with 𝐻𝐶𝑙? Problem Draw the products formed when each alkene is treated with 𝐻𝐶𝑙. Problem Addition of 𝐻𝐵𝑟 to which of the following alkenes will lead to a rearrangement? Stereochemistry of Electrophilic Addition of 𝑯𝑿 ▪ Trigonal planar atoms react with reagents from two directions with equal probability. ▪ Achiral starting materials yield achiral or racemic products. An achiral alkene forms an achiral alkyl halide. Addition converts 𝑠𝑝2 hybridized carbons to 𝑠𝑝3 hybridized carbons, however, sometimes new stereogenic centers are formed from hydrohalogenation. Stereogenic center at one of the newly formed 𝑠𝑝3 hybridized carbons, an equal amount of two enantiomers—a racemic mixture—must form. Stereochemistry of Electrophilic Addition of 𝑯𝑿 Initial addition of the electrophile 𝐻 + (from 𝐻𝐶𝑙) occurs from either side of the planar double bond to form a carbocation. Both modes of addition (from above and below) generate the same achiral carbocation. Nucleophilic attack of Cl– on the trigonal planar carbocation also occurs from two different directions, forming two products, A and B, having a new stereogenic center. A and B are not superimposable, so they are enantiomers. Because attack from either direction occurs with equal probability, a racemic mixture of A and B is formed. Stereochemistry of Electrophilic Addition of 𝑯𝑿 Because hydrohalogenation begins with a planar double bond and forms a planar carbocation, addition of 𝐻 and 𝐶𝑙 occurs in two different ways. The elements of 𝐻 and 𝐶𝑙 can both be added from the same side of the double bond—that is, syn addition— or they can be added from opposite sides—that is, anti addition. Both modes of addition occur in this two-step reaction mechanism. Stereochemistry of Electrophilic Addition of 𝑯𝑿 Initial addition of 𝐻 + (from 𝐻𝐶𝑙) forms two enantiomeric carbocations that react with the 𝐶𝑙 – nucleophile from two different directions, forming four stereoisomers, A– D—two pairs of enantiomers. Reaction of 1,2 − 𝑑𝑖𝑚𝑒𝑡ℎ𝑦𝑙𝑐𝑦𝑐𝑙𝑜ℎ𝑒𝑥𝑒𝑛𝑒 with 𝐻𝐶𝑙 forms four stereoisomers. ▪ Compounds A and D are enantiomers formed in equal amounts. ▪ Compounds B and C are enantiomers formed in equal amounts. Electrophilic Addition of 𝑯𝑿 Problem Draw the products, including stereochemistry, of each reaction. Hydration – Electrophilic Addition of Water ▪ It is the addition of water to an alkene to form an alcohol. ▪ It is the reverse of the dehydration of an alcohol to form an alkene. The addition of 𝐻 + (from 𝐻3 𝑂+ ) to generate a carbocation, followed by nucleophilic attack of 𝐻2 𝑂. Hydration – Electrophilic Addition of Water Hydration – Electrophilic Addition of Water There are three consequences to the formation of carbocation intermediates: ▪ In unsymmetrical alkenes, 𝐻 adds to the less substituted carbon to form the more stable carbocation (Markovnikov’s rule) ▪ Addition of 𝐻 and 𝑂𝐻 occurs in both a syn and anti process ▪ Carbocation rearrangements can occur Alcohols add to alkenes, forming ethers, using the same mechanism. Problem What two alkenes give rise to each alcohol as the major product of acid- catalyzed hydration? Halogenation – Addition of Halogen ▪ It is the addition of halogen 𝑋2 (𝑋 = 𝐶𝑙 𝑜𝑟 𝐵𝑟) to an alkene, forming a vicinal dihalide. Halogens add to 𝜋 bonds because halogens are polarizable. The electron-rich double bond induces a dipole in an approaching halogen molecule, making one halogen atom electron deficient and the other electron rich (𝑋 𝛿+ − 𝑋 𝛿− ). The electrophilic halogen atom is then attracted to the nucleophilic double bond, making addition possible. Halogenation – Addition of Halogen Difference of halogenation from hydrohalogenation or hydration. ▪ No rearrangements occur ▪ Only anti addition of 𝑋2 is observed ▪ Carbocations are not intermediates in halogenation Bridged halonium ions resemble carbocations in that they are short-lived intermediates that react readily with nucleophiles. Carbocations are inherently unstable because only six electrons surround carbon, whereas halonium ions are unstable because they contain a strained three-membered ring with a positively charged halogen atom. Halogenation – Addition of Halogen Problem Draw the products of each reaction, including stereochemistry. Stereochemistry of Halogenation Example Stereochemistry Step Initial addition of the electrophile 𝐶𝑙 + (from 𝐶𝑙2 ) occurs from either side of the planar double bond to form the bridged chloronium ion. Stereochemistry of Halogenation Example Step Nucleophilic attack of 𝑪𝒍– must occur from the back side—that is, from the side of the five-membered ring opposite Stereochemistry to the side having the bridged chloronium ion. Because the nucleophile attacks from below in this example and the leaving group departs from above, the two Cl atoms in the product are oriented trans to each other. Backside attack occurs with equal probability at either carbon of the three- membered ring to yield an equal amount of two enantiomers—a racemic mixture. Stereochemistry of Halogenation The mechanism for halogenation of alkene occurs in two steps: ▪ Addition of 𝑋 + forms an unstable bridged halonium ion in the rate- determining step. ▪ Nucleophilic attack of 𝑋 − occurs from the back side to form trans products. The overall result is anti addition of 𝑋2 across the double bond. A reaction is stereospecific when each of two specific stereoisomers of a starting material yields a particular stereoisomer of a product. Stereochemistry of Halogenation To draw the products of halogenation: ▪ Add 𝐵𝑟2 in an anti fashion across the double bond, leaving all other groups in their original orientations. Draw the products such that a given Br atom is above the plane in one product and below the plane in the other product. ▪ Sometimes this reaction produces two stereoisomers, as in the case of cis-2-butene, which forms an equal amount of two enantiomers. Sometimes it produces a single compound, as in the case of trans-2-butene, where a meso compound is formed. Problem Draw all stereoisomers formed in each reaction. Halohydrin Formation Treatment of an alkene with a halogen 𝑿𝟐 and 𝑯𝟐 𝑶 forms a halohydrin by addition of the elements of 𝑿 and 𝑶𝑯 to the double bond. Mechanism of halohydrin formation (similar to halogenation): Step. Addition of electrophile 𝑋 + (from 𝑋2 ) to form a bridged halonium ion Step. Nucleophilic attack by 𝐻2 𝑂 from the back side on the three-membered ring Halohydrin Formation Stereochemistry and Regioselectivity of Halohydrin Formation Because the bridged halonium ion ring is opened by backside attack of 𝐻2 𝑂, addition of X and OH occurs in an anti fashion and trans products are formed. Example Draw the products of the following reaction, including stereochemistry. Product Stereochemistry and Regioselectivity of Halohydrin Formation With unsymmetrical alkenes, two constitutional isomers are possible from addition of 𝑋 and 𝑂𝐻, but only one is formed. The preferred product has the electrophile 𝑿+ bonded to the less substituted carbon atom—that is, the carbon that has more 𝐻 atoms to begin with in the reacting alkene. Thus, the nucleophile (𝑯𝟐 𝑶) bonds to the more substituted carbon. Illustration Stereochemistry and Regioselectivity of Halohydrin Formation As in the opening of an epoxide ring, nucleophilic attack occurs at the more substituted carbon end of the bridged halonium ion because that carbon is better able to accommodate a partial positive charge in the transition state. Illustration Halohydrin Formation Problem Draw the products of each reaction and indicate their stereochemistry. Hydroboration – Oxidation ▪ It is a two-step reaction sequence that converts an alkene to an alcohol. ▪ Hydroboration is the addition of borane (𝐵𝐻3 ) to an alkene, forming an alkylborane. ▪ Oxidation converts the 𝐶 − 𝐵 bond of the alkylborane to a 𝐶 − 𝑂 bond. Hydroboration – Oxidation Borane (𝑩𝑯𝟑 ) is a reactive gas that exists mostly as the dimer, diborane (𝐵2 𝐻6 ). Borane is a strong Lewis acid that reacts readily with Lewis bases. For ease in handling in the laboratory, it is commonly used as a complex with tetrahydrofuran (THF). Hydroboration It is the first in hydroboration-oxidation where the elements of 𝐻 and 𝐵𝐻2 are added to the 𝜋 bond of the alkene, forming an intermediate alkylborane. The proposed mechanism involves a concerted addition of 𝑯 and 𝑩𝑯𝟐 from the same side of the planar double bond: the 𝜋 bond and 𝐻 − 𝐵𝐻2 bond are broken as two new 𝜎 bonds are formed. Because four atoms are involved, the transition state is said to be four-centered. Hydroboration All three 𝐵 − 𝐻 bonds actually react with three equivalents of an alkene to form a trialkylborane. Because only one 𝐵 − 𝐻 bond is needed for hydroboration, commercially available dialkylboranes having the general structure 𝑹𝟐 𝑩𝑯 are sometimes used instead of 𝐵𝐻3. Hydroboration Hydroboration is regioselective. With unsymmetrical alkenes, the boron atom bonds to the less substituted carbon atom. The larger boron atom bonds to the less sterically hindered, more accessible carbon atom. Hydroboration Because 𝐻 is more electronegative than 𝐵, the 𝐵 − 𝐻 bond is polarized to give boron a partial positive charge ( 𝐻 𝛿– − 𝐵𝛿+ ), making 𝐵𝐻2 the electrophile in hydroboration. If bond breaking and bond making are not completely symmetrical, boron bears a partial negative charge in the transition state and carbon bears a partial positive charge. Because alkyl groups stabilize a positive charge, the more stable transition state has the partial positive charge on the more substituted carbon Problem What alkylborane is formed from hydroboration of each alkene? Oxidation of the Alkylborane Oxidation replaces the 𝑪 − 𝑩 bond with a 𝑪 − 𝑶 bond, forming a new 𝑶𝑯 group with retention of configuration; the 𝑶𝑯 group replaces the 𝑩𝑯𝟐 group in the same position relative to the other three groups on carbon. To draw the product of a hydroboration-oxidation reaction, below are facts on its stereochemistry: ▪ Hydroboration occurs with sync addition. ▪ Oxidation occurs with retention of configuration. The overall result of this two-step sequence is syn addition of the elements of 𝑯 and 𝑶𝑯 to a double bond. The 𝑂𝐻 group bonds to the less substituted carbon. Oxidation of the Alkylborane Example Draw the product of the following reaction sequence, including stereochemistry. Solution Hydroboration–oxidation results in the addition of H and OH in a syn fashion across the double bond. The achiral alkene is converted to an equal mixture of two enantiomers—that is, a racemic mixture of alcohols. Problem Draw the products formed when each alkene is treated with 𝐵𝐻3 followed by 𝐻2 𝑂2 , 𝐻𝑂–. Include the stereochemistry at all stereogenic centers. Problem What alkene can be used to prepare each alcohol as the exclusive product of a two-step hydroboration–oxidation sequence? Hydroboration-Oxidation of Alkenes Reactions of Alkynes Preparation of Alkynes Alkynes are prepared by elimination reactions. A strong base removes two equivalents of 𝑯𝑿 from a vicinal or geminal dihalide to yield an alkyne by two successive 𝐸2 eliminations. Because vicinal dihalides are synthesized by adding halogens to alkenes, an alkene can be converted to an alkyne by the two-step process. 16 Chem211E Organic Chemistry Chem 211E Unit IX. Alkynes 6 Preparation of Alkynes Example Convert alkene A into alkyne B by a stepwise method. Solution Step Addition of 𝑋2 forms a vicinal dihalide. Step Elimination of two equivalents of 𝐻𝑋 forms two 𝜋 bonds. 16 Chem211E Organic Chemistry Chem 211E Unit IX. Alkynes 7 Introduction to Alkyne Reactions All reactions of alkynes occur because they contain easily broken 𝝅 bonds or, in the case of terminal alkynes, an acidic, 𝒔𝒑 hybridized 𝑪 − 𝑯 bond. A. Addition Reaction Alkynes undergo addition reactions because they contain weak 𝝅bonds. Two sequential reactions take place: addition of one equivalent of reagent forms an alkene, which then adds a second equivalent of reagent to yield a product having four new bonds. 16 Chem211E Organic Chemistry Chem 211E Unit IX. Alkynes 8 A. Addition Reaction Alkynes are electron rich. The two 𝜋 bonds form a cylinder of electron density between the two 𝑠𝑝 hybridized carbon atoms, and this exposed electron density makes a triple bond nucleophilic. As a result, alkynes react with electrophiles. 16 Chem211E Organic Chemistry Chem 211E Unit IX. Alkynes 9 Introduction to Alkyne Reactions B. Terminal Alkynes – Reaction as an Acid Because 𝒔𝒑 hybridized 𝑪 − 𝑯 bonds are more acidic than 𝒔𝒑𝟐 and 𝒔𝒑𝟑 hybridized 𝑪 − 𝑯 bonds, terminal alkynes are readily deprotonated with strong base in a Brønsted–Lowry acid–base reaction. The resulting anion is called an acetylide anion. Because an acid–base equilibrium favors the weaker acid and base, only bases having conjugate acids with 𝒑𝑲𝒂 values higher than the terminal alkynes, 𝒑𝑲𝒂 values > 𝟐𝟓 —are strong enough to form a significant concentration of acetylide anion. 17 Chem211E Organic Chemistry Chem 211E Unit IX. Alkynes 0 Introduction to Alkyne Reactions B. Terminal Alkynes – Reaction as an Acid The acetylide anions formed by deprotonating terminal alkynes are strong nucleophiles that can react with a variety of electrophiles. 17 Chem211E Organic Chemistry Chem 211E Unit IX. Alkynes 1 Hydrohalogenation – Addition of Hydrogen Halides Two equivalents of 𝐻𝑋 are usually used: addition of one mole forms a vinyl halide, which then reacts with a second mole of HX to form a geminal dihalide. ▪ With two equivalents of 𝑯𝑿, both 𝑯 atoms bond to the same carbon. ▪ With a terminal alkyne, both 𝑯 atoms bond to the terminal carbon; the hydrohalogenation of alkynes follows Markovnikov’s rule. 17 Chem211E Organic Chemistry Chem 211E Unit IX. Alkynes 2 Hydrohalogenation – Addition of Hydrogen Halides ▪ With only one equivalent of HX, the reaction stops with formation of the vinyl halide. 17 Chem211E Organic Chemistry Chem 211E Unit IX. Alkynes 3 Hydrohalogenation – Addition of Hydrogen Halides Addition of two equivalents of HX to an alkyne involves two steps for each addition of 𝑯𝑿: addition of 𝐻+ (from 𝐻𝑋) to form a carbocation, followed by nucleophilic attack of 𝑋 –. 17 Chem211E Organic Chemistry Chem 211E Unit IX. Alkynes 4 Hydrohalogenation – Addition of Hydrogen Halides The vinyl carbocation formed in Step is 𝑠𝑝 hybridized and therefore less stable than a 2° 𝑠𝑝2 hybridized carbocation In Step two carbocations are possible but only one is formed. Markovnikov addition in Step places the H on the terminal carbon (C1) to form the more substituted carbocation A. Because the more stable carbocation is formed faster, carbocation A must be more stable than carbocation B. 17 Chem211E Organic Chemistry Chem 211E Unit IX. Alkynes 5 Hydrohalogenation – Addition of Hydrogen Halides A is stabilized by resonance but B is not. Two resonance structures can be drawn for carbocation A, but only one Lewis structure can be drawn for carbocation B. ▪ Resonance stabilizes a molecule by delocalizing charge and electron density. ▪ Thus, halogens stabilize an adjacent positive charge by resonance. Markovnikov’s rule applies to the addition of 𝐻𝑋 to vinyl halides because addition of 𝑯+ forms a resonance-stabilized carbocation. 17 Chem211E Organic Chemistry Chem 211E Unit IX. Alkynes 6 Problem Draw the organic products formed when each alkyne is treated with two equivalents of 𝐻𝐵𝑟. 17 Chem211E Organic Chemistry Chem 211E Unit IX. Alkynes 7 Problem Draw an additional resonance structure for each cation. 17 Chem211E Organic Chemistry Chem 211E Unit IX. Alkynes 8 Halogenation – Addition of Halogen Addition of one mole of 𝑋2 forms a trans dihalide, which can then react with a second mole of 𝑋2 to yield a tetrahalide. Each addition of 𝑋2 involves a two-step process with a bridged halonium ion intermediate, reminiscent of the addition of 𝑋2 to alkenes. A trans dihalide is formed after addition of one equivalent of 𝑋2 because the intermediate halonium ion ring is opened upon backside attack of the nucleophile. 17 Chem211E Organic Chemistry Chem 211E Unit IX. Alkynes 9 Halogenation – Addition of Halogen 18 Chem 211E Unit IX. Alkynes 0 Problem Draw the products formed when 𝐶𝐻3 𝐶𝐻2 𝐶 ≡ 𝐶𝐶𝐻2 𝐶𝐻3 is treated with each reagent: (a) 𝐵𝑟2 (2 𝑒𝑞𝑢𝑖𝑣); (b) 𝐶𝑙2 (1 𝑒𝑞𝑢𝑖𝑣). Addition of Water In the presence of strong acid or 𝐻𝑔2+ , catalyst, the elements of 𝑯𝟐 𝑶 add to the triple bond, but the initial addition product, an enol, is unstable and rearranges to a product containing a carbonyl group (𝐶 = 𝑂). Internal alkynes undergo hydration with concentrated acid, whereas terminal alkynes require the presence of an additional 𝐻𝑔2+ catalyst—usually 𝐻𝑔𝑆𝑂4 —to yield methyl ketones by Markovnikov addition of 𝑯𝟐 𝑶. 18 Chem211E Organic Chemistry Chem 211E Unit IX. Alkynes 2 Addition of Water 18 Chem211E Organic Chemistry Chem 211E Unit IX. Alkynes 3 Addition of Water A and B are called tautomers: A is the enol form and B is the keto form of the tautomer. ▪ Tautomers are constitutional isomers that differ in the location of double bond and a hydrogen atom. Two tautomers are in equilibrium with each other. ▪ An enol tautomer has an 𝑂 − 𝐻 group bonded to a 𝐶 = 𝐶. ▪ A keto tautomer has a 𝐶 = 𝑂 and an additional 𝐶 − 𝐻bond. Equilibrium favors the keto form largely because a C––O is much stronger than a C––C. Tautomerization, the process of converting one tautomer into another, is catalyzed by both acid and base. Under the strongly acidic conditions of hydration, tautomerization of the enol to the keto form occurs Chem211E Organicrapidly Chemistry by a two-step process: protonation, followed by deprotonation Chem 211E Unit IX. Alkynes 18 4 Addition of Water Mechanism of Tautomerization in Acid 18 Chem211E Organic Chemistry Chem 211E Unit IX. Alkynes 5 Mechanism of Hydration of an Alkyne 18 Chem211E Organic Chemistry Chem 211E Unit IX. Alkynes 6 Addition of Water Examples Draw the enol intermediate and the ketone product formed in the reaction. Solution Mechanism 18 Chem211E Organic Chemistry Chem 211E Unit IX. Alkynes 7 Problem Draw the keto tautomer of each enol. 18 Chem211E Organic Chemistry Chem 211E Unit IX. Alkynes 8 Hydroboration-Oxidation Hydroboration–oxidation is a two-step reaction sequence that converts an alkyne to a carbonyl compound. ▪ Addition of borane forms an organoborane. ▪ Oxidation with basic 𝑯𝟐 𝑶𝟐 forms an enol. ▪ Tautomerization of the enol forms a carbonyl compound. ▪ The overall result is addition of 𝑯𝟐 𝑶 to a triple bond. 18 Chem211E Organic Chemistry Chem 211E Unit IX. Alkynes 9 Hydroboration-Oxidation Hydroboration–oxidation of an internal alkyne forms a ketone. Hydroboration of a terminal alkyne adds BH2 to the less substituted, terminal carbon. After oxidation to the enol, tautomerization yields an aldehyde, a carbonyl compound having a hydrogen atom bonded to the carbonyl carbon. Hydration (𝐻2 𝑂, 𝐻2 𝑆𝑂4 , and 𝐻𝑔𝑆𝑂4 ) and hydroboration–oxidation (𝐵𝐻3 followed by 𝐻_2𝑂2 , 𝐻𝑂– ) both add the elements of 𝑯𝟐 𝑶 across a triple bond. 19 Chem211E Organic Chemistry Chem 211E Unit IX. Alkynes 0 Hydroboration-Oxidation Problem Draw the product formed when 𝐶𝐻3 𝐶𝐻2 𝐶 ≡ 𝐶𝐻 is treated with each of the following sets of reagents: (a) 𝐻2 𝑂, 𝐻2 𝑆𝑂4 ; and (b) 𝐵𝐻3 followed by 𝐻2 𝑂2 , 𝐻𝑂−. Solution ▪ Addition of 𝐻2 𝑂 using 𝐻2 𝑂, 𝐻2 𝑆𝑂4 , and 𝐻𝑔𝑆𝑂4 forms methyl ketones from terminal alkynes. ▪ Addition of 𝐻2 𝑂 using 𝐵𝐻3 , then 𝐻2 𝑂2 , 𝐻𝑂− form aldehydes from terminal alkynes. 19 Chem211E Organic Chemistry Chem 211E Unit IX. Alkynes 1 Problem Draw the products formed when the following alkynes are treated with each set of reagents: (a) 𝐻2 𝑂, 𝐻2 𝑆𝑂4 , 𝐻𝑔𝑆𝑂4 ; and (b) 𝐵𝐻3 followed by 𝐻2 𝑂2 , 𝐻𝑂−. 19 Chem211E Organic Chemistry Chem 211E Unit IX. Alkynes 2