Obj 5 Respiratory 20241 PDF
Document Details
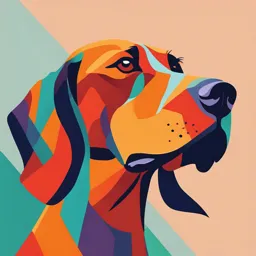
Uploaded by GloriousPanFlute
2016
Tags
Related
- Respiratory System Anatomy & Physiology PDF
- Respiratory System Anatomy & Physiology PDF
- Cardio-Respiratory Anatomy and Physiology Part 1 (Prelim) PDF
- Respiratory System Anatomy and Physiology PDF
- Human Anatomy & Physiology - Respiratory System PDF
- Essentials of Human Anatomy & Physiology (Chapter 13) PDF
Summary
This document is a detailed study guide on the respiratory system, covering its structure, function, and various processes, including ventilation, gas exchange, and transport of gases in the body.
Full Transcript
Objective 5: The Respiratory System PN 1241 Chapter 22 5. Discuss the structure and function of the respiratory system. 5.1 Define key terms related to the respiratory system. 5.2 Discuss the primary functions of the respiratory system. 5.3 Discuss the three phases of respiration. 5.5 Identify the m...
Objective 5: The Respiratory System PN 1241 Chapter 22 5. Discuss the structure and function of the respiratory system. 5.1 Define key terms related to the respiratory system. 5.2 Discuss the primary functions of the respiratory system. 5.3 Discuss the three phases of respiration. 5.5 Identify the main structures of the respiratory system. 5.6 Discuss the structure and the functions of the external nose, nasal cavity and paranasal sinuses. 5.7 Discuss the structure and the functions of the: a) pharynx b) larynx c) trachea d) bronchi e) lungs 5.8 Discuss the pressure relationships in the thoracic cavity. 5.9 Discuss the events in pulmonary ventilation. 5.10 Discuss the lung volumes and capacities during pulmonary ventilation. 5.11 Discuss the various pulmonary function tests used to evaluate respiratory function. 5.12 Discuss the process of gas exchange between the blood, lungs and tissues. 5.13 Discuss how oxygen and carbon dioxide is transported in the blood. 5.14 Discuss how respiration (breathing) is regulated and controlled. Respiratory System Major functions of respiratory system: supply body with O2 Get rid of CO2, Respiratory and circulatory system are closely coupled Also functions in olfaction and speech 5 Functions of Respiratory System | Respiratory Anatomy (visiblebody.com) © 2016 Pearson Education, Inc. Respiratory System Respiration involves four processes 1. 2. 3. 4. Pulmonary ventilation (breathing): movement of air into and out of lungs External respiration: exchange of O2 and CO2 between lungs and blood Transport of O2 and CO2 in blood Internal respiration: exchange of O2 and CO2 between blood vessels and tissues Respiratory system Circulatory system © 2016 Pearson Education, Inc. Part 1 Functional Anatomy Major organs Nose and nasal cavity Paranasal sinuses Pharynx Upper respiratory Larynx Trachea Bronchi and branches Lungs and alveoli Lower respiratory © 2016 Pearson Education, Inc. Figure 22.1 The major respiratory organs in relation to surrounding structures. Nasal cavity Nostril Oral cavity Pharynx Larynx Trachea Carina of trachea Right main (primary) bronchus Left main (primary) bronchus Left lung Diaphragm Right lung © 2016 Pearson Education, Inc. Table 22.1 The Upper Respiratory System © 2016 Pearson Education, Inc. Table 22.2 The Lower Respiratory System © 2016 Pearson Education, Inc. Upper Respiratory System 1. The Nose and Paranasal Sinuses Nose is only external portion of respiratory system Functions of nose: Provides an airway for respiration Moistens and warms entering air Filters and cleans inspired air Serves as resonating chamber for speech Houses olfactory receptors Divided into two regions: external nose and nasal cavity © 2016 Pearson Education, Inc. The Nose and Paranasal Sinuses (cont.) External nose Nostrils (nares) External nose is formed by: Nasal and frontal bones superiorly (bridge of nose) Maxillary bones laterally Plates of hyaline cartilage inferiorly © 2016 Pearson Education, Inc. The Nose and Paranasal Sinuses (cont.) Nasal cavity Divided by nasal septum Septum formed by cartilage, vomer bone and ethmoid bone Posteriorly nasal cavity turns into nasopharynx © 2016 Pearson Education, Inc. The Nose and Paranasal Sinuses (cont.) Nasal cavity (cont.) Nasal vestibule: nasal cavity superior to nostrils Contains sebaceous & sweat glands Lined with hairs that filter coarse particles from inspired air Rest of nasal cavity lined with 2 types of mucous membranes: Olfactory mucosa: contains smell receptors Respiratory mucosa: contains many seromucous nasal glands © 2016 Pearson Education, Inc. The Nose and Paranasal Sinuses (cont.) Nasal conchae Scroll-like, mucosa-covered projections that protrude from wall of nasal cavity Functions of conchae During inhalation, conchae and nasal mucosa: Filter, heat, and moisten air During exhalation these structures: Reclaim heat and moisture This minimizes the amount of moisture and heat lost from body through breathing © 2016 Pearson Education, Inc. The Nose and Paranasal Sinuses (cont.) Paranasal sinuses Paranasal sinuses form ring around nasal cavities Located in frontal, sphenoid, ethmoid, and maxillary bones Functions: Lighten skull Secrete mucus Help to warm and moisten air Nose blowing helps drain sinuses © 2016 Pearson Education, Inc. The Pharynx Pharynx (throat): funnel-shaped muscular tube that runs from base of skull to vertebra C6 Connects nasal cavity and mouth to larynx and esophagus Composed of skeletal muscle Three regions: Nasopharynx Oropharynx Laryngopharynx © 2016 Pearson Education, Inc. Figure 22.4a The pharynx, larynx, and upper trachea. Nasopharynx Oropharynx Laryngopharynx Regions of the pharynx © 2016 Pearson Education, Inc. The Pharynx (cont.) Nasopharynx Air passageway Soft palate and uvula close nasopharynx during swallowing Pharyngeal tonsils (adenoids) located here Pharyngotympanic tubes (auditory tubes) drain and equalize pressure in middle ear and open into lateral walls © 2016 Pearson Education, Inc. The Pharynx (cont.) Oropharynx Passageway for food and air Palatine tonsils located in lateral walls Lingual tonsil located on posterior surface of tongue Laryngopharynx Passageway for food and air Extends to larynx, where it is continuous with esophagus Summary: Upper Respiratory System | Respiratory Anatomy (visiblebody.com) © 2016 Pearson Education, Inc. Lower Respiratory System Consists of larynx, trachea, bronchi and lungs The Larynx voice box; contains vocal cords Opens into laryngopharynx and is continuous with trachea Three functions of larynx: 1. 2. 3. Provides patent airway Routes air and food into proper channels Voice production – houses vocal cords © 2016 Pearson Education, Inc. The Larynx (cont.) Framework of larynx consists of nine hyaline cartilages (except for epiglottis), connected by membranes and ligaments 1. 2. 3. 5. 7. 9. Thyroid cartilage: large, shield-shaped cartilage that contains laryngeal prominence (Adam’s apple) Cricoid cartilage: ring-shaped & 4. Paired arytenoid cartilages & 6. Paired cuneiform cartilages & 8. Paired corniculate cartilages Epiglottis © 2016 Pearson Education, Inc. The Larynx (cont.) Epiglottis Spoon shaped Consists of elastic cartilage (not hyaline) Covered in taste bud–containing mucosa Prevents food or liquids from entering respiratory channels during swallowing © 2016 Pearson Education, Inc. The Larynx (cont.) Vocal folds Vocal ligaments: form core of vocal folds (true vocal cords) on each side Glottis: opening between vocal folds Folds vibrate to produce sound as air rushes up from lungs Vestibular folds (false vocal cords): Superior to vocal folds No part in sound production Help to close the glottis during swallowing © 2016 Pearson Education, Inc. Figure 22.6 Movements of the vocal folds. Epiglottis Vestibular fold (false vocal cord) Vocal fold (true vocal cord) Glottis Inner lining of trachea Cuneiform cartilage Corniculate cartilage Vocal folds in closed position; closed glottis © 2016 Pearson Education, Inc. Vocal folds in open position; open glottis The Larynx (cont.) Sphincter functions of the larynx Vocal folds may act as sphincter to prevent air passage Example: Valsalva’s maneuver Glottis closes to prevent exhalation Abdominal muscles contract Intra-abdominal pressure rises Helps to empty rectum or stabilizes trunk during heavy lifting © 2016 Pearson Education, Inc. The Trachea Windpipe extends from larynx to main bronchi It is about 4 inches long, 3/4 inch in diameter, and very flexible Expands for breathing © 2016 Pearson Education, Inc. The Trachea (cont.) Wall composed of three layers Mucosa: propels mucus toward pharynx Submucosa: seromucous glands supported by 16–20 C-shaped cartilage rings that prevent collapse of trachea Adventitia: outermost layer made of connective tissue; encases C-shaped rings © 2016 Pearson Education, Inc. The Trachea (cont.) Trachealis Consists of smooth muscle fibers that connect posterior parts of cartilage rings Contracts during coughing to expel mucus Expands as swallowed food passes through Carina Last tracheal cartilage that is expanded and found at point where trachea branches into two main bronchi Mucosa of carina highly sensitive Violent coughing will be triggered if any foreign object makes contact with it © 2016 Pearson Education, Inc. The Bronchi and Subdivisions Trachea divides to form Rt and Lt main bronchi Rt and Lt main bronchi run into their respective lungs Air passages further branch out Branching referred to as bronchial tree Each main bronchus then branches in to lobar (secondary) bronchi: 3 on Rt 2 on Lt Each Lobar bronchus supplies one lobe © 2016 Pearson Education, Inc. The Bronchi and Subdivisions (cont.) Each lobar bronchus branches into segmental (tertiary) bronchi Segmental bronchi divide repeatedly Branches become smaller and smaller Bronchioles: less than 1 mm in diameter Terminal bronchioles: smallest of all branches Less than 0.5 mm in diameter © 2016 Pearson Education, Inc. Figure 22.8 Conducting zone passages. Trachea Superior lobe of left lung Left main (primary) bronchus Superior lobe of right lung Lobar (secondary) bronchus Middle lobe of right lung Segmental (tertiary) bronchus Inferior lobe of right lung Inferior lobe of left lung © 2016 Pearson Education, Inc. The Bronchi and Subdivisions (cont.) respiratory bronchioles lead into alveolar ducts and finally into alveolar sacs (saccules) Alveolar sacs contain clusters of alveoli ~300 million alveoli make up most of lung volume Sites of actual gas exchange © 2016 Pearson Education, Inc. Figure 22.9a Respiratory zone structures. Alveoli Alveolar duct Respiratory bronchioles Terminal bronchiole © 2016 Pearson Education, Inc. Alveolar duct Alveolar sac The Bronchi and Subdivisions (cont.) Respiratory membrane Blood air barrier that allows gas exchange across membrane by simple diffusion Alveolar walls consist of: Single layer of squamous epithelium Scattered cuboidal alveolar cells which secrete surfactant and antimicrobial proteins © 2016 Pearson Education, Inc. Lungs and Pleurae Lungs flank the mediastinum in thoracic cavity Root: site of vascular and bronchial attachment to mediastinum Costal surface: surfaces in close contact with ribs Apex: superior tip, deep to clavicle Base: inferior surface that rests on diaphragm © 2016 Pearson Education, Inc. Gross Anatomy of the Lungs (cont.) Hilum: is the site for entry/exit of blood vessels, bronchi, lymphatic vessels, and nerves Left lung: separated into superior and inferior lobes by oblique fissure (2 lobes) Smaller than right because of position of heart Cardiac notch: concavity for heart to fit into Right lung: separated into superior, middle, and inferior lobes (3 lobes) Superior and middle lobes separated by horizontal fissure Middle and inferior lobes separated by oblique fissure © 2016 Pearson Education, Inc. Figure 22.11a Anatomical relationships of organs in the thoracic cavity. Intercostal muscle Rib Parietal pleura Pleural cavity Visceral pleura Lung Trachea Thymus Apex of lung Right superior lobe Horizontal fissure Right middle lobe Oblique fissure Left superior lobe Oblique fissure Left inferior lobe Right inferior lobe Heart (in mediastinum) Diaphragm Base of lung Cardiac notch Anterior view. The lungs flank mediastinal structures laterally. © 2016 Pearson Education, Inc. Gross Anatomy of the Lungs (cont.) Each lobe further divided into bronchopulmonary segments 10 on right and 8–10 on left Each segment is served by its own artery, vein, and bronchus If one segment is diseased, it can be individually removed © 2016 Pearson Education, Inc. Gross Anatomy of the Lungs (cont.) Lobules: smallest subdivisions visible to naked eye; served by bronchioles and their branches Lungs are mostly composed of alveoli; the rest consists of stroma - elastic connective tissue Makes lungs very elastic and spongy © 2016 Pearson Education, Inc. The Pleurae Pleurae: thin, double-layered serosal membrane that divides thoracic cavity Parietal pleura: lines the thoracic wall and mediastinum Visceral pleura: membrane on external lung surface Pleural fluid fills pleural cavity between two pleurae Provides lubrication and surface tension that assists in expansion and recoil of lungs Summary: Lower Respiratory System | Respiratory Anatomy (visiblebody.com) © 2016 Pearson Education, Inc. Part 2 – Respiratory Physiology 22.4 Mechanics of Breathing Pulmonary ventilation consists of two phases Inspiration: gases flow into lungs Expiration: gases exit lungs © 2016 Pearson Education, Inc. Pressure Relationships in the Thoracic Cavity Atmospheric pressure * Pressure exerted by air surrounding the body Intrapulmonary pressure * Pressure in alveoli Also called intra-alveolar pressure Fluctuates with breathing Always eventually equalizes with atmospheric pressure © 2016 Pearson Education, Inc. Pressure Relationships in the Thoracic Cavity (cont.) Intrapleural pressure * Pressure in pleural cavity Fluctuates with breathing **Always a negative pressure in order to keep lungs inflated © 2016 Pearson Education, Inc. Pulmonary Ventilation Consists of inspiration and expiration Mechanical process that depends on volume changes in thoracic cavity Volume changes lead to pressure changes Pressure changes lead to the flow of gases to equalize pressure Gases always move from an area of high pressure to an area of low pressure* © 2016 Pearson Education, Inc. Pulmonary Ventilation (cont.) Inspiration* Active process involving inspiratory muscles (contraction of diaphragm and external intercostals) Action of the diaphragm: when dome-shaped diaphragm contracts, moves up and flattens out; there is an increase in thoracic volume Action of intercostal muscles: when external intercostals contract, rib cage is lifted up and out Results in increase in thoracic volume © 2016 Pearson Education, Inc. Pulmonary Ventilation (cont.) Inspiration (cont.) As thoracic cavity volume increases, lungs are stretched as they are pulled out with thoracic cage Intrapulmonary pressure drops Because of difference between atmospheric and intrapulmonary pressure, air rushes into lungs until intrapulmonary and atmospheric pressures are equalized © 2016 Pearson Education, Inc. Pulmonary Ventilation (cont.) Inspiration (cont.) Forced (deep) inspiration can occur during vigorous exercise or in people with COPD Accessory muscles are also activated Act to further increase thoracic cage size, creating a larger pressure gradient so more air is drawn in Pulmonary Ventilation (cont.) Expiration* passive process Inspiratory muscles relax, thoracic cavity volume decreases, and lungs recoil Volume decrease causes intrapulmonary pressure (Ppul) to increase so air flows out of lungs down its pressure gradient until intrapulmonary pressure is equal to atmospheric pressure © 2016 Pearson Education, Inc. Slide 11 Sequence of events Changes in anterior-posterior and superior-inferior dimensions Changes in lateral dimensions (superior view) 1 Inspiratory muscles contract (diaphragm descends; rib cage rises). Figure 22.15 Changes in thoracic volume and sequence of events during inspiration and expiration. Inspiration 2 Thoracic cavity volume increases. Ribs are elevated and sternum flares as external intercostals contract. 3 Lungs are stretched; intrapulmonary volume increases. External intercostals contract. 4 Intrapulmonary pressure drops (to −1 mm Hg). 5 Air (gases) flows into lungs down its pressure gradient until intrapulmonary pressure is 0 (equal to atmospheric pressure). Diaphragm moves inferiorly during contraction. 1 Inspiratory muscles relax (diaphragm rises; rib cage descends due to recoil of costal cartilages). Expiration 2 Thoracic cavity volume decreases. Ribs and sternum are depressed as external intercostals relax. 3 Elastic lungs recoil passively; intrapulmonary volume decreases. External intercostals relax. 4 Intrapulmonary pressure rises (to +1 mm Hg). 5 Air (gases) flows out of lungs down its pressure gradient until intrapulmonary pressure is 0. © 2016 Pearson Education, Inc. Diaphragm moves superiorly as it relaxes. Pulmonary Ventilation (cont.) Non-respiratory air movements May modify normal respiratory rhythm Most result from reflex action, although some are voluntary Examples: coughing, sneezing, crying, laughing, hiccups, and yawns © 2016 Pearson Education, Inc. Physical Factors Influencing Pulmonary Ventilation Three physical factors influence the ease of air passage and the amount of energy required for ventilation: Airway resistance Alveolar surface tension Lung compliance © 2016 Pearson Education, Inc. Physical Factors Influencing Pulmonary Ventilation Airway resistance Friction: major non-elastic source of resistance to gas flow* Decreases air passage Causes breathing movements to become more strenuous Greatest resistance to airflow occurs in midsize bronchi © 2016 Pearson Education, Inc. Physical Factors Influencing Pulmonary Ventilation (cont.) Alveolar surface tension Surface tension: the attraction of liquid molecules to one another at a gasliquid interface Tends to draw liquid molecules closer together and reduce contact with dissimilar gas molecules Resists any force that tends to increase surface area of liquid Tends to cause alveoli to shrink to smallest size—that is, collapse *surfactant, a fat-protein complex, prevents alveolar collapse Produced by alveolar cells © 2016 Pearson Education, Inc. Physical Factors Influencing Pulmonary Ventilation (cont.) Lung compliance Lung compliance: Measure of how much “stretch” the lung has Normally high because of: Distensibility of lung tissue Surfactant, which decreases alveolar surface tension Higher lung compliance means it is easier to expand lungs Depends on elasticity of lung tissue and flexibility of bony thorax If compliance issues exist, inspiration becomes harder © 2016 Pearson Education, Inc. Assessing Ventilation* Several respiratory volumes can be used to assess respiratory status Respiratory volumes can be combined to calculate respiratory capacities, which can give information on a person’s respiratory status Respiratory volumes and capacities are usually abnormal in people with pulmonary disorders © 2016 Pearson Education, Inc. Respiratory Volumes (cont.) Tidal volume (TV): amount of air moved into and out of lung with each breath Averages ~500ml Inspiratory reserve volume (IRV): amount of air that can be inspired forcibly beyond the tidal volume (2100–3200 ml) © 2016 Pearson Education, Inc. Respiratory Volumes (cont.) Expiratory reserve volume (ERV): amount of air that can be forcibly expelled from lungs (1000–1200 ml) Residual volume (RV): amount of air that always remains in lungs Needed to keep alveoli open © 2016 Pearson Education, Inc. Respiratory Capacities Combinations of two or more respiratory volumes Inspiratory capacity (IC): sum of tidal volume (TV) + inspiratory reserve volume (IRV) Functional residual capacity (FRC): sum of residual volume (RV) + expiratory reserve volume (ERV) *Vital capacity (VC): sum of tidal volume (TV) + Inspiratory reserve volume (IRV) + expiratory reserve volume (ERV) *Total lung capacity (TLC): sum of all lung volumes (TV + IRV+ ERV + RV) © 2016 Pearson Education, Inc. Gas exchange Occurs between lungs and blood as well as blood and tissues External respiration: diffusion of gases between blood and lungs Internal respiration: diffusion of gases between blood and tissues Pulmonary Function Tests (cont.) Pulmonary functions tests can measure rate of gas movement Forced vital capacity (FVC): amount of gas forcibly expelled after taking deep breath Forced expiratory volume (FEV): amount of gas expelled during specific time interval of FVC Healthy individuals can expel 80% of FVC in 1st second Patients with obstructive disease exhale less than 80% in 1st second, whereas those with restrictive disease exhale 80% or more even with reduced FVC © 2016 Pearson Education, Inc. Alveolar Ventilation Minute ventilation: total amount of gas that flows into or out of respiratory tract in 1 minute Normal at rest = ~ 6 L/min Normal with exercise = up to 200 L/min Only rough estimate of respiratory efficiency Alveolar ventilation rate (AVR): flow of gases into and out of alveoli during a particular time Better indicator of effective ventilation as it accounts for the anatomical dead space © 2016 Pearson Education, Inc. Gas Exchange Gas exchange occurs between lungs and blood as well as blood and tissues External respiration: diffusion of gases between blood and lungs Internal respiration: diffusion of gases between blood and tissues Both are subject to: basic properties of gases Composition of alveolar gas © 2016 Pearson Education, Inc. Properties of Gases Dalton’s Law of Partial Pressures the total pressure of a mixture of gases is the sum of the partial pressures of the individual components. Partial pressure: The mixture pressure exerted by an individual gas in a Dalton’s Law In lungs: Alveoli – PO2 is high PC02 is low Because inspiration occurred Pulmonary capillaries – PO2 is low PCO2 is high *Both gases flow from area of high to low concentration Blood just came from tissues Dalton’s Law Systemic (Tissues): Tissues – PO2 is low PCO2 is high Because tissues using oxygen Tissue capillaries – PO2 is high PCO2 is low * both gases flow from area of high to low concentration Because blood just came from lungs Dalton’s Law the relative concentration of gases (their partial pressures) does not change as the pressure and volume of the gas mixture changes so air inhaled into the lungs will have the same relative concentration of gases as atmospheric air. In the lungs, the relative concentration of gases determines the rate at which each gas will diffuse across the alveolar membranes Henry’s Law predicts how gasses will dissolve in the alveoli and bloodstream during gas exchange. The amount of oxygen that dissolves into the bloodstream is directly relational to the partial pressure of oxygen in alveolar air. For gas mixtures in contact with liquid: Each gas will dissolve in the liquid in proportion to its partial pressure At equilibrium, partial pressures in two phases will be equal Amount of each gas that dissolves depends on: Solubility: CO2 more soluble in water than O2 Temperature: at temp of liquid rises, solubility decreases ex: Hyperbaric chamber (100% O2) Composition of Alveolar Gas Alveoli contain more CO2 and water vapor and less O2 than atmospheric air because: Gas exchanges in lungs (O2 diffuse out of lungs and CO2 diffuse into lung) Humidification of air by conducting passages Mixing of alveolar gas with each breath Newly inspired air mixes with air that was left in passageways between breaths © 2016 Pearson Education, Inc. External Respiration External respiration involves the exchange of O2 and CO2 across respiratory membranes Exchange is influenced by: pressure gradients (Dalton’s Law) and gas solubilities (Henry’s Law) Thickness and surface area of respiratory membrane The thicker the membrane, the less diffusion; thinner the membrane, more diffusion. This affects the rate of gas exchange © 2016 Pearson Education, Inc. External Respiration (cont.) Partial pressure gradients and gas solubilities A steep partial pressure gradient for O2 exists between blood and lungs Venous blood PO2 = 40 mm Hg Alveolar PO2 = 104 mm Hg Drives oxygen flow into blood © 2016 Pearson Education, Inc. External Respiration (cont.) Partial pressure gradient for CO2 is less steep Venous blood PCO2 = 45 mm Hg Alveolar PCO2 = 40 mm Hg Though gradient is not as steep, CO2 still diffuses in equal amounts with oxygen Reason is that CO2 is 20 more soluble in plasma and alveolar fluid than oxygen © 2016 Pearson Education, Inc. External Respiration (cont.) Thickness and surface area of the respiratory membrane Gas movement occurs by diffusion Rate of diffusion depends on the concentration of the gas and the barrier; especially the thickness of the respiratory membranes Respiratory membranes are very thin 0.5 to 1 m thick Large total surface area of the alveoli is 40 the surface area of the skin © 2016 Pearson Education, Inc. Internal Respiration Internal respiration involves gas exchange between blood and tissues Partial pressure and diffusion gradients are reversed compared to external respiration Tissue PO2 is always lower than arterial blood PO2, so O2 moves from blood to tissues Tissues PCO2 is always higher than arterial blood PCO2 so CO2 moves from tissues into blood © 2016 Pearson Education, Inc. Transport of Respiratory Gases by Blood Oxygen Transport Molecular O2 is carried in blood in two ways: dissolved in plasma bound to hemoglobin (Hb) in RBCs (98.5%) © 2016 Pearson Education, Inc. Oxygen Transport (cont.) Association of oxygen and hemoglobin each Hgb can transport four oxygen molecules Oxyhemoglobin (HgbO2): hemoglobin-O2 combination Deoxyhemoglobin(HHb) hemoglobin that has released O2 © 2016 Pearson Education, Inc. Oxygen Transport (cont.) Association of oxygen and hemoglobin (cont.) Loading and unloading of O2 is facilitated by a change in shape of Hgb As O2 binds, Hgb changes shape, increasing its attraction for O2 As O2 is released, Hgb shape change causes a decrease in attraction for O2 Fully saturated (100%): all four heme groups carry O2 Partially saturated: when only one to three hemes carry O2 © 2016 Pearson Education, Inc. Oxygen Transport (cont.) Association of oxygen and hemoglobin (cont.) Rate of loading and unloading of O2 is regulated to ensure adequate oxygen delivery to cells Factors that influence hemoglobin saturation: P O2 Other factors such as: Temperature Blood pH PCO2 Concentration of BPG © 2016 Pearson Education, Inc. Oxygen Transport (cont.) Influence of PO2 on hemoglobin saturation PO2 heavily influences binding and release of O2 with hemoglobin In arterial blood: Hgb is 98% saturated Further increases in PO2 (as in deep breathing) produce minimal increases in O2 binding In venous blood: Hgb is still 75% saturated Venous reserve: oxygen remaining in venous blood that can still be used © 2016 Pearson Education, Inc. Oxygen Transport (cont.) Influence of other factors on hemoglobin saturation Increases in temperature, H+ and PCO2 can modify structure of hemoglobin Results in a decrease for Hgb’s affinity for O2 Enhances O2 unloading (Bohr’s effect) Decreases in these factors decreases oxygen unloading from blood © 2016 Pearson Education, Inc. Carbon Dioxide Transport CO2 is transported in blood in three forms: dissolved in plasma as PCO2 bound to hemoglobin Referred to as carbaminohemoglobin 70% is transported as bicarbonate ions (HCO3–) in plasma Accumulating CO2 lowers blood pH Depletion of CO2 in blood raises blood pH © 2016 Pearson Education, Inc. Carbon Dioxide Transport Occurs primarily in RBCs, where enzyme carbonic anhydrase reversibly and rapidly catalyzes this reaction In systemic capillaries, after bicarbonate is created, it quickly diffuses from RBCs to plasma This outpouring of bicarbonate from RBCs is balanced as chloride moves into RBCs from plasma (chloride shift) Carbon Dioxide Transport In pulmonary capillaries, the process occurs in reverse Bicarbonate moves into RBCs while Chloride moves out of RBCs back into plasma Bicarbonate binds with H+ to form H2CO3 (carbonic acid) Carbonic acid is split by carbonic anhydrase into CO2 and H20 CO2 diffuses into alveoli Carbon Dioxide Transport (cont.) Influence of CO2 on blood pH Carbonic acid–bicarbonate buffer system: helps blood resist changes in pH If H+ concentration in blood rises, excess H+ is removed by combining with Bicarbonate (HCO3–) to form carbonic acid (H2CO3), which dissociates into CO2 and H2O If H+ concentration begins to drop, H2CO3 separates, releasing H+ HCO3– is considered the alkaline reserve of carbonic acid-bicarbonate buffer system © 2016 Pearson Education, Inc. Carbon Dioxide Transport (cont.) Changes in respiratory rate and depth affect blood pH Slow, shallow breathing causes an increase in CO2 in blood, resulting in a drop in pH Rapid, deep breathing causes a decrease in CO2 in blood, resulting in a rise in pH Changes in ventilation can help adjust pH when disturbances are caused by metabolic factors Breathing plays a major role in acid-base balance of body © 2016 Pearson Education, Inc. Control of Respiration Respiratory rhythms are regulated by: higher brain centers chemoreceptors other reflexes © 2016 Pearson Education, Inc. Neural Mechanisms Neural controls involve neurons in reticular formation of medulla and pons Medullary respiratory centers Clustered neurons in two areas of medulla are most important: Ventral respiratory group (VRG) Sets normal respiratory rate and rhythm Dorsal respiratory group (DRG) Assists VRG © 2016 Pearson Education, Inc. Neural Mechanisms (cont.) Pontine respiratory centers Neurons in this center influence and modify activity of Ventral Respiratory Group (VRG) Act to smooth out transition between inspiration and expiration and vice versa Transmit impulses to VRG that modify and fine-tune breathing rhythms during vocalization, sleep, exercise © 2016 Pearson Education, Inc. Factors Influencing Breathing Rate and Depth Depth is determined by how actively the respiratory center stimulates respiratory muscles The greater the stimulation, the greater the number of motor units excited, increasing depth of inspiration Rate is determined by how long center is active Both are modified by changing body demands © 2016 Pearson Education, Inc. Factors Influencing Breathing Rate and Depth (cont.) Respiratory centers are affected by: Chemical factors Influence of higher brain centers Pulmonary irritant reflexes Inflation reflex © 2016 Pearson Education, Inc. Factors Influencing Breathing Rate and Depth (cont.) Chemical factors Most important of all factors affecting depth and rate of inspiration* Changing levels of PCO2, PO2, and pH are most important Levels of these chemicals are sensed by: Central chemoreceptors: located throughout brain stem Peripheral chemoreceptors: found in aortic arch and carotid arteries © 2016 Pearson Education, Inc. Factors Influencing Breathing Rate and Depth (cont.) Influence of PCO2 * Most potent and most closely controlled blood PCO2 levels rise (hypercapnia), its most powerful respiratory stimulant*** Respiratory centers increase depth and rate of breathing, which act to lower blood PCO2, and pH rises to normal levels If blood PCO2 levels decrease (hypocapnia), respiration becomes slow and shallow © 2016 Pearson Education, Inc. Factors Influencing Breathing Rate and Depth (cont.) Chemical factors (cont.) Influence of PO2 Peripheral chemoreceptors in aortic and carotid bodies sense arterial O2 levels Declining PO2 normally has only slight effect on ventilation because of huge O2 reservoir bound to Hgb Requires substantial drop in arterial PO2 (below 60 mmHg) to stimulate increased ventilation When excited, chemoreceptors cause respiratory centers to increase ventilation © 2016 Pearson Education, Inc. Factors Influencing Breathing Rate and Depth (cont.) Influence of arterial pH pH can modify respiratory rate and rhythm even if CO2 and O2 levels are normal Mediated by peripheral chemoreceptors Decreased pH may reflect CO2 retention, accumulation of lactic acid, or excess ketone bodies Respiratory system controls attempt to raise pH by increasing respiratory rate and depth © 2016 Pearson Education, Inc. Factors Influencing Breathing Rate and Depth (cont.) Summary of chemical factors* Rising CO2 levels are most powerful respiratory stimulant (via central chemoreceptors) Normally, blood PO2 affects breathing only indirectly by influencing peripheral chemoreceptor sensitivity to changes in PCO2 When arterial PO2 falls below 60 mmHg, it becomes major stimulus for respiration (via peripheral chemoreceptors) Changes in arterial pH resulting from CO2 retention or metabolic factors act indirectly through peripheral chemoreceptors © 2016 Pearson Education, Inc. Factors influencing Breathing Rate and Depth (cont.) Influence of higher brain centers Hypothalamic controls: modify rate and depth of respiration Example: breath holding that occurs in anger or gasping with pain Change in body temperature changes rate Cortical controls: bypass medullary controls Example: voluntary breath holding at least until brain stem reinstates breathing when blood CO2 becomes critical © 2016 Pearson Education, Inc. Factors influencing Breathing Rate and Depth (cont.) Pulmonary irritant reflexes Receptors in bronchioles respond to irritants such as dust, accumulated mucus, or noxious fumes Receptors communicate with respiratory centers via vagal nerve Promote reflexive constriction of air passages Same irritant triggers a cough in trachea or bronchi or a sneeze in nasal cavity © 2016 Pearson Education, Inc. Factors influencing Breathing Rate and Depth (cont.) Inflation reflex Hering-Breuer reflex (inflation reflex) Stretch receptors in pleurae and airways are stimulated by lung inflation Send inhibitory signals to medullary respiratory centers to end inhalation and allow expiration May act as protective response more than as a normal regulatory mechanism a reflex triggered to prevent over-inflation of the lung* © 2016 Pearson Education, Inc. Crash Course Videos Part 1: https://www.youtube.com/watch?v=RnnibQkbL3M Part 2: https://www.youtube.com/watch?v=Ppcddv2HDfw Song: https://www.youtube.com/watch?v=p4zOXOM6wgE Review Quizzes https://quizlet.com/770430/respiratory-system-review-flash-cards/ https://quizlet.com/5239338/ap-ch23-respiratory-system-review-flashcards/ https://www.proprofs.com/quiz-school/story.php?title=p-respiratory-system https://quizlet.com/1741092/ap-respiratory-system-flash-cards/ https://quizlet.com/5239338/ap-ch23-respiratory-system-review-flash-cards https://quizlet.com/110760915/respiratory-system-ap-review-questionsflash-cards/ https://quizlet.com/289106560/ap-exam-3-review-respiratory-system-flashcards/ https://quizlet.com/131171976/ap-ii-review-sheet-36-anatomy-of-therespiratory-system-flash-cards/ https://quizlet.com/1969368/respiratory-system-ap-flash-cards/