Nutrient Cycling in Soil PDF
Document Details
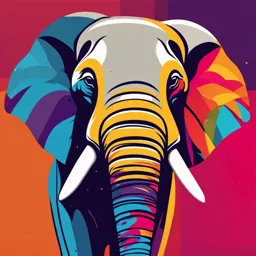
Uploaded by MarvellousOrphism7158
Tags
Summary
This document provides an overview of nutrient cycling in soil. It details the various biogeochemical processes involved and the indicators used to assess the cycling process. The document also explores the crucial role of decomposition and the impact of human activities on soil organic matter.
Full Transcript
Carbon and Nutrient cycling in soil Soil nutrient cycling involves different biogeochemical processes in which soil stores and moderates the release of nutrients from one pool into another in a cyclic manner. Nutrients can be transformed into plant available forms during these processes. Some of the...
Carbon and Nutrient cycling in soil Soil nutrient cycling involves different biogeochemical processes in which soil stores and moderates the release of nutrients from one pool into another in a cyclic manner. Nutrients can be transformed into plant available forms during these processes. Some of the nutrients are held in the soil or lost to air or water. Soil nutrient cycling can be assessed by measuring Fertility Indicators, Organic matter indicators and Soil reaction indicators. Fertility Indicators include mineral nitrogen (Total soil N), potentially mineralizable nitrogen, available N (ammonium and nitrate), available phosphorus, exchangeable cations (e.g. potassium, calcium, magnesium and sodium), sulphur, boron, and zinc, and micronutrients contents (e.g Mn, Fe, I, B, Cu, Zn, etc) Organic Matter Indicators include total soil organic carbon or total soil organic matter, C:N ratio, decomposition rate e.g. basal respiration or net N or C mineralization or immobilization, microbial biomass carbon, particulate organic matter, soil enzymes activity, lignin:N ratio, total extractable polyphenol:N ratio, etc. Soil Reaction Indicators include mainly soil pH and electrical conductivity. Global nutrient cycles that occur in soil include carbon, water, and nutrients (e.g. N, P, S, etc) cycles. During nutrient cycling, nitrogen, phosphorus, and many other nutrients are stored, transformed, and cycled through soil. Decomposition Decomposition is the breakdown of organic materials in the soil. Decomposition is driven by soil organisms , leading to transformation and cycling of nutrients through the environment (soil, water, and air). Decomposition liberates carbon and nutrients from the complex material that make up life forms, putting them back into biological circulation so that they are available to plants and other organisms. Decomposition also degrades compounds in soil that would be pollutants if they entered ground or surface water. Decomposition is a stepwise process involving virtually all soil organisms. Arthropods and earthworms chew the material and mix it with soil. A few fungi may break apart one complex compound into simpler components, then bacteria can attack the newly created compounds, and so on. Each organism gets energy or nutrients from the process. During decomposition, compounds often, but not always become simpler after each step. The portion of plant and animal residue that is not broken down, plays a crucial role in soil. It is transformed into the highly complex organic compounds called humic substances that can persist in soil for a long time. Humus is important for soil structure and nutrient storage. Terrestrial carbon cycle The carbon cycle illustrates the role of soil in cycling carbon through the environment. More carbon is stored in soil than in the atmosphere and above-ground biomass combined. Soil carbon originates from organic compounds which are created through photosynthesis in which plants convert atmospheric carbon dioxide (CO2) into organic carbon compounds, such as carbohydrates, proteins, oils, and fibers. When plants and animals die and leave their residue in or on the soil the organic compounds enter the soil system. Soil organisms consume the organic matter, extract energy and nutrients and release water, heat, and CO2 back to the atmosphere. Thus, if no new plant residue is added to the soil, soil organic matter will gradually disappear. If plant residue is added to the soil at a faster rate than soil organisms convert it to CO2, carbon will gradually be removed from the atmosphere and stored (sequestered) in the soil. However, human activities like tillage aerate the soil, triggering increased biological activity, and therefore rapid decomposition and loss of soil organic matter through release of CO2 into the atmosphere. Carbon dioxide (CO2) and methane (CH4) are the main carbon-based atmospheric gases. Autotrophic organisms (mainly plants), as well as photo- and chemo- autotrophic microbes synthesize atmospheric CO2 into organic material. Dead organic material (mainly in the form of plant residues and exudates) is incorporated into the soil by soil fauna, leading to carbon inputs into the soil through organic material transformation by heterotrophic microorganisms. The process of organic material transformation leads to the presence of a complex mixture of plant litter compounds and microbial decomposition products in various stages of decomposition. These products can be mixed with soil minerals and occluded within soil aggregates, enabling SOC persistence in soil for decades, centuries or even millennia. When soil organic matter (SOM) is decomposed (or mineralized) by microorganisms CO2 is emitted back into the atmosphere. Carbon loss can also be caused by root exudates such as oxalic acid, which liberate organic compounds from protective mineral associations. Furthermore, carbon is partly lost from soils to rivers and oceans as dissolved organic carbon (DOC) or as part of erosion material. In principle, the amount of SOC stored in any soil depends on the equilibrium between the amount of C that enters the soil and the amount of C that is lost from the soil as gas through respiration as a result of microbial mineralization and, to a lesser extent, leaching from the soil as DOC. Decomposition of organic matter in soil is influenced by soil temperature and water content, which are mainly determined by climatic conditions. The composition of the microbial community (e.g. the bacteria: fungi ratio) may also have an influence on the preferential decomposition of certain compounds. Some scientist argue that the persistence of SOM in soil is rather affected by SOC stabilization in the soil matrix through its interaction and association with soil minerals and that the chemical recalcitrance of complex molecules such as lignin or lipids that build up SOC does not substantially contribute to SOM persistence in soil. SOM can be divided into different pools based on the time needed for full decomposition and the derived residence time of the products in the soil (turnover time) as follows: Active pools - turnover in months or few years; Passive pools - turnover in up to thousands of years. Long turnover times of organic compounds are explained by : i. anaerobic conditions such as in peats ii. incorporation of SOM components into soil aggregates iii. attachment of organic matter to protective mineral surfaces iv. spatial disconnection between SOM and decomposers, and v. intrinsic biochemical properties of SOM. Fast pool (labile or active pool) - After addition of fresh organic carbon to the soil, decomposition results in a large proportion of the initial biomass being lost in 1–2 years. Intermediate pool - Comprises microbially processed organic carbon that is partially stabilized on mineral surfaces and/or protected within aggregates, with turnover times in the range 10-100 years. Slow pool (refractory or stable pool) - highly stabilized SOC, enters a period of very slow turnover of 100 to >1 000 years. SOIL: A Source and sink for carbon-based GHGs Anthropogenic activities can turn soil into either a net sink or a net source of GHGs. As a source, soil emits GHGs into the atmosphere where they trap thermal radiation that enhances the greenhouse effect and contributes to global warming. The carbon-based GHGs emitted by soil are CO2 and methane (CH4) which are two of the most leading anthropogenically emitted GHGs. Another form of GHG is nitrous oxide (N2O), the emission of which has become increasingly anthropogenically driven, largely from agricultural soils and livestock facilities. The inclusion of all three gases in soil CO2 budgets is important due to the interconnectedness of the processes involved in their emissions and ecosystem cycling (carbon- nitrogen, aerobic–anaerobic processes). The potential climate signal of these gases differs depending on their relative greenhouse efficiency, i.e. their global warming potential (GWP). CO2 is considered to have a GWP of 1, followed by CH4 with a 100-year GWP of 28 and N2O with the highest 100-year GWP of 265 (IPCC, 2014). As a sink soil can store carbon(C sequestration) Soil organic carbon sequestration is the process by which carbon is fixed from the atmosphere via plants or organic residues and stored in the soil. When dealing with CO2, SOC sequestration involves three stages: the removal of CO2 from the atmosphere via plant photosynthesis; the transfer of carbon from CO2 to plant biomass; and the transfer of carbon from plant biomass to the soil where it is stored in the form of SOC in the most labile pool. Soil carbon saturation The concept of soil carbon saturation implies that the soil carbon stock has reached its maximum carrying capacity for storing soil carbon inputs. It is also referred to in as the maximum carbon stabilization capacity. The soil carbon saturation depends on many factors including inherent and dynamic soil properties and their interactions with abiotic factors, When a C saturation level is reached, SOC sequestration comes to an end; soils stop being a net carbon sink and may become a net carbon source.