null.docx
Document Details
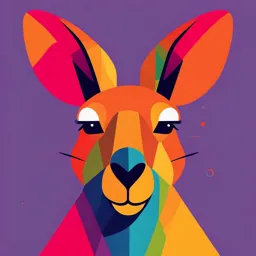
Uploaded by DecisiveHonor
Department of Anesthesia
Full Transcript
62 Haemorrhagic diseases 78 63 Thrombocytopenia 78 63 Vitamin K deficiency 79 68 Disseminated intravascular coagulation 79 70 Congenital disorders 79 73 Review and revise 79 73 Animations 76 4.1 Blood cell formation and functions 63 77 4.2 Red blood cells 63 77 4.3 Haemolyt...
62 Haemorrhagic diseases 78 63 Thrombocytopenia 78 63 Vitamin K deficiency 79 68 Disseminated intravascular coagulation 79 70 Congenital disorders 79 73 Review and revise 79 73 Animations 76 4.1 Blood cell formation and functions 63 77 4.2 Red blood cells 63 77 4.3 Haemolytic disease of the newborn 67 77 4.4 Whiteblood cells 68 77 4.5 Platelets and clot formation 70 Plasma Cellular content of blood Erythrocytes (red blood cells) Leukocytes(white blood cells) Platelets (thrombocytes) Erythrocyte disorders Anaemias Polycythaemia Leukocyte disorders Leukopenia Leukocytosis Leukaemia Blood is a fluid connective tissue. It circulates constantly around the body, propelled by the pumping action of the heart. It transports: ·oxygen · nutrients ·hormones ·heat ·antibodies and cells of the immune system · clotting factors · wastes. Blood is composed of a clear,straw-coloured,watery fluid called plasma, in which several different types of blood cell are suspended. Plasma normally constitutes 55% of the volume of blood and the cell fraction 45%. Blood cells and plasma can be separated by centrifugation (spinning) or by simple gravity when blood is allowed to stand (Fig.4.1A).The cells are heavier than plasma and sink to the bottom of the sample. Blood makes up about 7% of body weight (about 5.6litres in a 70 kg man). This proportion is less in women and considerably greater in children, gradually decreasing until the adult level is reached. The total blood volume in adults is about 80 mL/kg body weight in males and 70 mL/kg in females. The continual blood flow maintains a fairly constant environment for body cells. Blood volume and the concentration of blood constituents are kept within narrow limits by homeostatic mechanisms. Heat produced from metabolically active organs, such as working skeletal muscles and the liver, is distributed around the body by the bloodstream,maintaining core body temperature. SECTION 2 Communication Figure 4.1 Whole blood. (A) The proportions of blood cells and blood clot in serum. The first part of the chapter discusses normal blood physiology and the later sections describe some blood disorders. Effects of ageing on white blood cell function are described in Chapter 15. Plasma After studying this section,you should be able to: list the constituents of plasma describe their functions. The main constituent of plasma is water (90-92%), carrying a range of dissolved and suspended substances,including: ·plasma proteins ·inorganic salts (electrolytes) ·nutrients, principally from digested foods waste products · hormones ·gases. Plasma proteins Plasma proteins, which make up about 7% of plasma, are normally retained within the blood because they are too big to escape through the capillary pores into the tissues.They are largely responsible for creating the osmotic pressure of blood (p. 85), which keeps plasma fluid within the circulation. If plasma protein levels fall, because of either reduced production or loss from the blood vessels, osmotic pressure is also reduced, and fluid shifts into the tissues (oedema) and body cavities. Plasma viscosity (thickness) is due to the presence of plasma proteins, mainly albumin and fibrinogen. Plasma proteins, with the exception of immunoglobulins,are formed in the liver. Albumins These are the most abundant plasma proteins (about 60% of total) and their main function is to maintain normal plasma osmotic pressure. Albumins also act as carrier molecules for free fatty acids, some drugs and steroid hormones. Globulins The main functions of globulins are: · As antibodies (immunoglobulins), complex proteins produced by lymphocytes play an important part in immunity. They bind to, and neutralise,foreign materials (antigens) such as microorganisms (see also p.414). Transport of some hormones and mineral salts.For example,thyroglobulin carries the hormone thyroxine and transferrin carries the mineral iron. Inhibition of some proteolytic enzymes. For example,a2macroglobulin inhibits trypsin activity. Clotting factors These are responsible for coagulation of blood (p. 71). Serum is plasma from which clotting factors have been removed (Fig.4.1B). The most abundant clotting factor is fibrinogen. Electrolytes These have a range of functions, including muscle contraction (e.g. Ca2+), transmission of nerve impulses (e.g.Ca2+, K+ and Na+), and maintenance of acid-base balance (e.g. phosphate, PO-). Blood pH is maintained between 7.35and 7.45 (slightly alkaline) by an ongoing buffering system (p.31). Nutrients Nutrients, substances essential for cellular growth and metabolism, include glucose, amino acids and vitamins.They are transported in the bloodstream from sites of production or absorption to the tissues for immediate use or storage. Waste products Urea,creatinine and uric acid are the waste products of protein metabolism. They are formed in the liver and carried in blood to the kidneys for excretion. Carbon dioxide from tissue metabolism is transported to the lungs for excretion. Hormones Hormones (Ch. 9) are chemical messengers synthesised by endocrine glands; they are secreted into the blood and transported to their target tissues and organs around the body. Gases Oxygen is not very soluble in water, so only a small amount (less than 2%) can be transported dissolved in plasma. This is nowhere near enough to supply the body's needs, so an additional oxygen transport mechanism is needed: oxygen is bound to haemoglobin in red blood cells. Over 98% of oxygen in the blood is carried this way, as oxyhaemoglobin(p.65).Haemoglobin also binds some carbon dioxide, although most carbon dioxide is converted to bicarbonate ions in red blood cells, and then transported in the plasma (p.281). SPOT CHECK The blood CHAPTER 4 tissue. In the bone marrow, all blood cells originate from pluripotent (i.e. capable of developing into one of a number of cell types) stem cells and go through several developmental stages before entering the blood.Different types of blood cell follow separate lines of development. The process of blood cell formation is called haemopoiesis (Fig. 4.3). For the first few years of life, red marrow completely fills the space within bone. Over the next 20 years,it is largely replaced by fatty yellow marrow that has no haemopoietic function. In adults, haemopoiesis in the skeleton is confined to flat bones, irregular bones and the ends of long bones, the main sites being the sternum,ribs,pelvis and skull. 1.List the three main functions of the globulins. Erythrocytes (red blood cells) 4.2 2.What is the main constituent of plasma? Cellular content of blood 4.1 After studying this section, you should be ableto: discuss the structure, function and formation of red blood cells, including the systems used in medicine to classify the different types discuss the functions and formation of the different types of white blood cell outline the role of platelets in blood clotting. There are three types of blood cell (Fig. 4.2; see also Fig. 1.3). ·erythrocytes (red cells) ·leukocytes (white cells) platelets(thrombocytes). Most blood cells are synthesised in red bone marrow.Some lymphocytes,additionally, are produced in lymphoid Figure 4.2 A blood smear, showing erythrocytes, a monocyte,a neutrophil, a lymphocyte and a platelet. (Biophoto Associates/Science Photo Library.Reproduced with permission.) Red blood cells are by far the most abundant type of blood cell; 99% of all blood cells are erythrocytes (see Fig.4.2).They are biconcave discs with no nucleus, and their diameter is about 7μm (Fig. 4.4). Their main function is the transport of gas, mainly oxygen, but they also carry some carbon dioxide.Their characteristic shape is suited to their purpose;the biconcavity increases their surface area for gas exchange,and the thin central portion allows fast entry and exit of gases. The cells are flexible, so they can squeeze through narrow capillaries, and contain no intracellular organelles,leaving more room for haemoglobin, the large pigmented protein responsible for gas transport. Their flattened shape allows them to stack like dinner plates in the bloodstream,reducing turbulence. Measurements of red cell numbers, volume and haemoglobin content are routine and useful assessments made inclinical practice (Table 4.1). The letters in brackets in the first column of the table are the abbreviations commonly used in laboratory reports. Lifespan and function of erythrocytes Because they have no nucleus, erythrocytes cannot divide and so need to be continually replaced by new cells from the red bone marrow, present in the ends of long bones and in flat and irregular bones. They pass through several stages of development before entering the blood. Their lifespan in the circulation is about 120 days. There are approximately 30 trillion (1014) red blood cells in the average human body,about 25% of the body's total cell count, and around 1%,mainly older cells,are cleared and destroyed daily. The process of erythrocyte development from stem cells takes about 7 days and is called erythropoiesis (see Fig.4.3). The immature cells are released into the bloodstream as reticulcytes, and mature into erythrocytes over a few days within the circulation. During this time, they lose their nucleus and therefore become incapable of division (Fig.4.5). Both vitamin B12 and folic acid are required for red blood cell synthesis. They are absorbed in the small intestine, SECTION 2 Communication Figure 4.3 Haemopoiesis. Stages in the development of blood cells. (Photographic inserts from Telser AG,Young JK, Baldwin KM 2007Elsevier's integrated histology. Mosby: Edinburgh; and Young B, Lowe JS,Stevens A et al. 2006 Wheater's functional histology: a text and colour atlas.Edinburgh:Churchill Livingstone. Reproduced with permission.) A B C 7μm Figure 4.4 The red blood cell. (A) Under the light microscope. (B) Drawn from the front. (C) Drawn in section. (D) Coloured scanning electron micrograph of a group of red blood cells travelling along an arteriole. (A, Telser AG, Young JK,Baldwin KM 2007 Elsevier's integrated histology.Edinburgh:Mosby.Reproduced with permission. D, Professors PM Motta and S Correr/Science Photo Library.Reproduced with permission.) Measure Normal values Erythrocyte count-number of erythrocytes per litre, or cubic millilitre (mm),of blood Male:4.5x1012/L to 6.5x1012/L (4.5-6.5 million/mm3) Female:3.8x1012/L to 5.8x1012/L (3.8-5.8 million/mm3) Packed cell volume (PCV, haematocrit) -the volume of red cells in 1 litre or mm3 of blood 0.40-0.55 L/L Mean cell volume (MCV) - the volume of an average cell,measured in femtolitres (1 fL=10-15 lilitre) 80-96 fL Haemoglobin (Hb)-the weight of haemoglobin in whole blood, measured in grams/100 mL of blood Male: 13-18 g/100 mL Female: 11.5- 16.5 g/100 mL Mean cell haemoglobin (MCH) -the average amount of haemoglobin per cell, measured in picograms (1(1 pg=10-12 gram) 27-32 pg/cell Mean cell haemoglobin concentration (MCHC) - the weight of haemoglobin in 100 mL of red cells 30-35 g/100 mL of red cells Figure 4.5 Life cycle of the erythrocyte. The blood CHAPTER 4 Figure 4.6 The haemoglobin molecule. although vitamin B12 must be bound to intrinsic factor (p.328) for absorption. Both vitamins are present in dairy products, meat and green vegetables. The liver usually contains substantial stores of vitamin B12 - several years'worth-but signs of folic acid deficiency appear within a few months.The life cycle of the erythrocyte is shown in Fig.4.5. Haemoglobin Haemoglobin is a large, complex molecule containing a globular protein (globin) and a pigmented iron-containing complex called haem. Each haemoglobin molecule contains four globin chains and four haem units, each with one atom of iron (Fig. 4.6). As each atom of iron can combine with an oxygen molecule, this means that a single haemoglobin molecule can carry up to four molecules of oxygen. An average red blood cell carries about 280 million haemoglobin molecules, giving each cell a theoretical oxygen-carrying capacity of over a billion oxygen molecules! Iron is carried in the bloodstream bound to its transport protein, transferrin, and stored in the liver. Normal red cell production requires a steady supply of iron.Absorption of iron from the alimentary canal is very slow, even if the diet is rich in iron, meaning that iron deficiency can readily occur if losses exceed intake. Oxygen transport When all four oxygen-binding sites on a haemoglobin molecule are full, it is described as saturated. Haemoglobin binds reversibly to oxygen to form oxyhaemoglobin,according to the equation: SECTION 2 Communication As the oxygen content of blood increases,its colour changes too. Blood rich in oxygen (usually arterial blood)is bright red because of the high levels of oxyhaemoglobin it contains, compared with blood with lower oxygen levels (usually venous blood), which is dark bluish in colour because it is not saturated. The association of oxygen with haemoglobin is a loose one, so that oxyhaemoglobin releases its oxygen readily,especially under certain conditions. Low pH Metabolically active tissues, e.g. exercising muscle, release acid waste products, and so the local pH falls. Under these conditions, oxyhaemoglobin readily breaks down, giving up additional oxygen for tissue use. Low oxygen levels (hypoxia) Figure 4.7 Control of erythropoiesis: the role of erythropoietin. Where oxygen levels are low, oxyhaemoglobin breaks down,releasing oxygen. In the tissues, which constantly consume oxygen, oxygen levels are always low. This increases oxygen release to the cells. In addition, the lower the tissue oxygen level,the more oxygen is released, meaning that as tissue oxygen demand rises, so does the supply to match it. On the other hand, when oxygen levels are high, as they are in the lungs, oxyhaemoglobin formation is favoured. Temperature Actively metabolising tissues, which have higher than normal oxygen needs, are warmer than less active ones,driving the equation above to the left and increasing oxygen release. This ensures that very active tissues receive a higher oxygen supply than less active ones. In the lungs,where the alveoli are exposed to inspired air,the temperature is lower,favouring oxyhaemoglobin formation. Control of erythropoiesis Red cell numbers remain fairly constant because the bone marrow produces erythrocytes at the rate at which they are destroyed. This is due to a homeostatic negative feedback mechanism. The hormone that regulates red blood cell production is erythropoietin, produced mainly by the kidney. The primary stimulus for increased erythropoiesis is hypoxia, i.e. deficient oxygen supply to body cells.Hypoxia can result from anaemia, low blood volume, poor blood flow, reduced oxygen content of inspired air (as at altitude)or lung disease. Each of these stimulates erythropoietin production in an attempt to restore oxygen supplies to the tissues. Erythropoietin stimulates an increase in the production of proerythroblasts and the release of more reticulocytes into the blood. It also speeds up reticulocyte maturation. These changes increase the oxygen-carrying capacityof the blood and reverse tissue hypoxia, the original stimulus. When the tissue hypoxia is reversed, erythropoietin production declines(Fig.4.7).When erythropoietin levels are low, red cell formation does not take place even in the presence of hypoxia,and anaemia (the inability of the blood to carry adequate oxygen for body needs) develops. Destruction of erythrocytes The lifespan of erythrocytes (see Fig. 4.5) is about 120days and their breakdown, or haemolysis, is carried out by macrophages in the spleen, bone marrow and liver.As erythrocytes age, their cell membranes become more fragile and so more susceptible to haemolysis. Iron released by haemolysis is returned to the bone marrow to form new haemoglobin molecules. Biliverdin is formed from the haem part of the haemoglobin. It is almost completely reduced to the yellow pigment bilirubin, before being bound to plasma globulin and transported to the liver (see Fig. 4.5; see also Fig. 12.37). In the liver it is changed from a fat-soluble to a water-soluble form to be excreted in bile. Blood groups Early attempts to transfuse blood from one person to another or from animals to humans were only rarely successful,the recipient of the blood usually becoming very ill or dying. It is now known that the red blood cell membrane carries a range of different proteins (called antigens) that can stimulate an immune response if transferred from one individual (the donor) into the bloodstream of an incompatible individual.These antigens, which are inherited, determine the individual's blood group. In addition, individuals can make antibodies to these antigens, but not to their own type of antigen, since if they did the antigens and antibodies would react, causing a potentially fatal transfusion reaction. If individuals are transfused with blood of the same group,i.e. possessing te same cell surface antigens, their immune system will not recognise them as foreign and will not reject them. However, if they are given blood from an individual of a different blood type, i.e. with a different type of antigen on the red cells, their immune system will generate antibodies to the foreign antigens and destroy the transfused cells. This is the basis of the transfusion reaction:the two blood types, the donor and the recipient, are incompatible. There are many different collections of red cell surface antigens, but the most important are the ABO and the Rhesus systems. The ABO system About 55% of the UK populatin has either A-type antigens (blood group A), B-type antigens (blood group B) or both (blood group AB) on their red cell surface.The remaining 45% have neither A- nor B-type antigens (blood group O). The corresponding antibodies are called anti-A and anti-B. Blood group A individuals cannot make anti-A (and therefore do not have these antibodies in their plasma),since otherwise a reaction to their own cells would occur;they can, however, make anti-B. Blood group B individuals,for the same reason, can make only anti-A. Blood group AB makes neither,and blood group O makes both anti-A and anti-B (Fig.4.8). Because blood group AB people make neither anti-A nor anti-B antibodies, they are sometimes known as universal recipients: transfusion of either type A or type B blood The blood CHAPTER 4 into these individuals is likely to be safe, since there are no antibodies to react with them. Conversely, group O people have neither A nor B antigens on their red cell membranes,and their blood may be safely transfused into A,B,AB or O types;group O is sometimes known as the universal donor.The terms universal donor and universal recipient are misleading, however, since they imply that the ABO system is the only one that needs to be considered. In practice,although the ABO systems may be compatible,other antigen systems on donor/recipient cells may be incompatible and cause a transfusion reaction (p. 76). For this reason, prior to transfusion, cross-matching is still required to ensure that there is no reaction between donor and recipient bloods.Inheritance of ABO blood groups is described in Chapter 17(p.482). The Rhesus system 4.3 The red blood cell membrane antigen that is important here is the Rhesus (Rh) antigen, or Rhesus factor. About 85% of people have this antigen; they are Rhesus-positive (Rh+) and do not therefore make anti-Rhesus antibodies.The remaining 15% have no Rhesus antigen (they are Rhesus-negative,or Rh-). Rh- individuals are capable of making anti-Rhesus antibodies, but are stimulated to do so only in certain circumstances, e.g. in pregnancy (p. 75), or as the result of an incompatible blood transfusion. Blood group A Antigen +antibody(ies) present B 8 Antigen A Makes anti-B As donor,is As recipient, is Compatible with: A and AB Incompatible with: B and O,because both make anti-A antibodies that will react with A antigens Compatible with: A and O Incompatible with: B and AB,because type A makes anti-B antibodies that will react with B antigens B B Antigen B Makes anti-A Compatible with: B and AB Incompatible with: A and O,because both make anti-B antibodies that will react with B antigens Compatible with: B and O Incompatible with: A and AB,because type B makes anti-A antibodies that will react with A antigens AB B Makes neither Antigens A and B anti-A nor anti-B Compatible with: AB only Incompatible with: A,B and O,because all three make antibodies that will react with AB antigens Compatible with all groups UNIVERSAL RECIPIENT AB makes no antibodies and therefore will not react with any type of donated blood 0 B A B Neither A nor Makes both B antigen anti-A and anti-B Compatible with all groups UNIVERSAL DONOR O red cells have no antigens, and will therefore no stimulate anti-A or anti-B antibodies Compatible with: O only Incompatible with: A,AB and B,because type O makes anti-A and anti-B antibodies Figure 4.8 The ABO system of blood grouping. Antigens, antibodies and compatibility. SECTION 2 Communication Table 4.2 Normal leukocyte counts in adult blood Numberx10°/L Percentage of total Granulocytes Neutrophils 2.5-7.5 40-75 Eosinophils 0.04-0.44 1-6 Basophils 0.015-0.1 <1 Agranulocytes Monocytes 0.2-0.8 2-10 Lymphocytes 1.5-3.5 20-50 Total 5-9 100 Basophil Neutrophil Eosinophil Figure 4.9 The granulocytes (granular leukocytes). (Adapted from Telser AG,Young JK,Baldwin KM 2007 Elsevier's integrated histology. Edinburgh: Mosby; and Young B, Lowe JS, Stevens A et al. 2006 Wheater's functional histology: a text and colour atlas.Edinburgh:Churchill Livingstone.Reproduced with permission.) Leukocytes (white blood cells) 4.4 These cells have an important function in defence and immunity. They detect foreign or abnormal (antigenic)material and destroy it, through a range of defence mechanisms described below and in Chapter 15. Leukocytes are the largest blood cells but they account for only about 1% of the blood volume. They contain nuclei and some have granules in their cytoplasm(Table 4.2;see also Fig. 4.2).There are two main types: ·granulocytes (polymorphonuclear leukocytes)-neutrophils, eosinophils and basophils ·agranulocytes-monocytes and lymphocytes. Rising white cell numbers in the bloodstream (leukocytosis) usually indicate a physiological problem, e.g.infection,trauma or malignancy. Granulocytes (polymorphonuclear leukocytes) During their formation, called granulopoiesis, granulocytes follow a common line of development through myeloblast to myelocyte before differentiating into the three types (Fig.4.9;see also Fig. 4.3). All granulocytes have multilobed nuclei in their cytoplasm. Their names represent the dyes they take up when stained in the laboratory.Eosinophils take up the red acid dye, eosin; basophils take up alkaline methylene blue;and neutrophils are purple because they take up both dyes. Neutrophils These small, fast and active scavengers protect the body against bacterial invasion, and remove dead cells and debris from damaged tissues. They are attracted in large numbers to any area of infection by chemicals called chemotaxins,released by damaged cells. Neutrophils are highly mobile,and squeeze through the capillary walls in the affected area by diapedesis (Fig. 4.10). Their numbers rise very quickly in an area of damaged or infected tissue. Once there,they engulf and kill bacteria by phagocytosis (Fig. 4.11;see also Figure 4.10 Diapedesis of leukocytes across the capillary wall. Fig.15.1).Their nuclei are characteristically complex,with up to six lobes (see Fig. 4.2), and their granules are lysosomes containing enzymes to digest engulfed material. Neutrophils live on average for 6-9 hours in the bloodstream. Pus forming in an infected area consists of dead tissue cells,dead and live microbes, and phagocytes killed by microbes. Eosinophils Eosinophils,although capable of phagocytosis, are less active in this process than neutrophils; their specialised role appears to be in the elimination of parasites, such as worms,which are too big to be phagocytosed. They are equipped with certain toxic chemicals, stored in their granules,which they release (degranulation) when the eosinophil binds to an infecting organism. The blood CHAPTER 4 Microbes Pseudopodium Pseudopodium Microbes engulfed Lymphocyte Monocyte Figure 4.11 Phagocytic action of neutrophils. Local accumulation of eosinophils may occur in allergic inflammation, such as the asthmatic airway and skin allergies. There, they promote tissue inflammation by releasing their array of toxic chemicals, but they may also dampen down the inflammatory process through the release of other chemicals, such as histaminase, an enzyme that breaks down histamine(p.410). Basophils Basophils, which are closely associated with allergic reactions, contain cytoplasmic granules packed with heparin (an anticoagulant), histamine (an inflammatory agent) and other substances that promote inflammation.Usually,the stimulus that causes basophil degranulation is an allergen (an antigen that causes allergy) of some type. This binds to antibody-type receptors on the basophil membrane.Mast cells are very similar to basophils, except that they are fixed in the tissues. Mast cells degranulate within seconds of binding an allergen, which accounts for the rapid onset of allergic symptoms following exposure to, for example,pollen in hay fever(p.417). Figure 4.12 The agranulocytes. Figure 4.13 The body's main fixed macrophage collections. ·acts on the hypothalamus, causing the rise in body temperature associated with microbial infections · stimulates the production of some globulins by the liver ·enhances the production of activated T-lymphocytes. Macrophages have important functions in inflammation (p.409)and immunity(Ch.15). Agranulocytes The monocytes and lymphocytes make up 25-50% of the total leukocyte count (Fig. 4.12; see also Fig. 4.3).They have a large nuceus and no cytoplasmic granules. Monocytes These are the largest of the white blood cells(see Fig. 4.2).Some circulate in the blood and are actively motile and phagocytic,while others migrate into the tissues and develop into macrophages. Both types of cell produce interleukin 1,which: The mononuclear phagocyte system This is sometimes called the reticuloendothelial system;it consists mainly of the body's complement of monocytes and macrophages. Some macrophages are mobile, whereas others are fixed, providing effective defence at key body locations. The main collections of fixed macrophages are shown in Fig.4.13. Macrophages have a diverse range of protective functions.They are actively phagocytic (their name means 'big eaters') and are much more powerful and longer-lived than the smaller neutrophils. They synthesise and release an array of biologically activechemicals,called cytokines, SECTION 2 Communication including interleukin 1 as mentioned earlier. They also have a central role linking the non-specific and specific (immune) systems of body defence(Ch. 15), and produce factors that are important in inflammation and repair.They can'wall off' indigestible pockets of material, isolating them from surrounding normal tissue. In the lungs, for example,resistant bacteria such as tuberculosis bacilli and inhaled inorganic dusts can be sealed off in such capsules. Lymphocytes Lymphocytes are smaller than monocytes and have large nuclei. Some circulate in the blood but most are found in tissues, including lymphatic tissue such as lymph nodes and the spleen. Lymphocytes develop from pluripotent stem cells in red bone marrow and from precursors in lymphoid tissue. Although all lymphocytes originate from only one type of stem cell, the final steps in their development lead to the production of two distinct types of lymphocyte -T-lymphocytes and B-lymphocytes.The specific functions of these two cell types are discussed in Chapter 15. Platelets (thrombocytes) 4.5 These are very small, disc-shaped cell fragments, 2-4 μm in diameter, budded off from the cytoplasm of megakaryocytes in red bone marrow (see Figs 4.2 and 4.3). Although they have no nucleus, their cytoplasm is packed with granules containing a variety of substances that promote blood clotting,which causes haemostasis (cessation of bleeding). The normal blood platelet count is between 200 x10°/L and 350 x10°/L (200,000-350,000/mm3). The mechanisms that regulate platelet numbers are not fully understood,but the hormone thrombopoietin from the liver stimulates platelet production. The lifespan of platelets is between 8 and 11 days and those not used in haemostasis are destroyed by macrophages,mainly in the spleen. About a third of platelets are stored within the spleen rather than in the circulation; this is an emergency store, released as required to control excessive bleeding. Haemostasis When a blood vessel is damaged, loss of blood is stopped (haemostasis; Fig. 4.14) and healing occurs in a series of overlapping processes, in which platelets play a vital part.The more badly damaged the vesse wall is, the faster coagulation begins, sometimes as quickly as 15 seconds after injury. 1. Vasoconstriction When platelets come into contact with a damaged blood vessel, their surface becomes sticky and they adhere to the damaged wall. They then release serotonin Vessel injury-Damaged blood vessel allows blood and blood components to escape into surrounding tissue Vasconstriction-Smooth muscle in the vessel wall contracts to reduce blood flow Platelet plug formation -Platelets stick to each other and form a temporary seal Coagulation-Thrombin converts soluble fibrinogen to an insoluble mesh of fibrin,trapping red blood cells and platelets and forming a stronger clot Figure 4.14 The stages of blood cloting. (5-hydroxytryptamine, 5-HT) and thromboxanes, which constrict the vessel, reducing or stopping blood flow through it. Other vasoconstrictors, e.g. endothelins,are released by the damaged vessel itself. 2.Platelet plug formation The sticky platelets clump together and release other substances, including adenosine diphosphate (ADP),which attract more platelets to the site. Passing platelets stick to those already at the damaged vessel and they too release their chemicals. This is a positive feedback system by which many platelets rapidly gather at the site of vascular damage The blood CHAPTER 4 Figure 4.15 The final common pathway in blood clotting. and quickly form a temporary seal - the platelet plug. Platelet plug formation is usually complete within 6 minutes of injury and can plug small holes in blood vessel walls. The plug is, however, soft and easily disrupted, and is the precursor to the far more durable blood clot. White blood 3. Coagulation (blood clotting) This is a complex process that also involves a positive feedback system and only a few stages are included here.The clotting factors involved are listed in Box 4.1. Their numbers represent the order in which they were discovered and not the order of participation in the clotting process.These clotting factors activate each other in a specific order, eventually resulting in the formation of prothrombin activator, which is the first step in the final common pathway.Prothrombin activates the enzyme thrombin, which converts inactive fibrinogen to insoluble threads of fibrin (Fig. 4.15).As clotting proceeds, the platelet plug is progressively stabilised by increasing amounts of fibrin laid down in a three-dimensional meshwork within it. The maturing blood clot traps blood cells and other plasma proteins,including plasminogen (which will eventually destroy the clot),and is much stronger than the rapidly formed platelet plug. The final common pathway can be initiated by two processes,which often occur together: the extrinsic and intrinsic pathways (Fig. 4.15). The extrinsic pathway is activated rapidly (within seconds) following tissue damage and is probably the more important of the two. Damaged tissue releases a complex of chemicals called thromboplastin or tissue factor, which initates coagulation. The intrinsic pathway is slower (3-6 minutes) and is triggered when Red blood cells Fibrin strands Figure 4.16 A blood clot. Scanning electron micrograph showing the fibrin meshwork (pink strands), red blood cells, platelets and a white blood cell. (CNRI/Science Photo Library. Reproduced with permission.) blood comes into contact with damaged blood vessel lining (endothelium). After a time the clot shrinks (retracts) because the platelets contract, squeezing out serum, a clear sticky fluid that consists of plasma from which clotting factors have been removed. Clot shrinkage pulls the edges of the damaged vessel together, reducing blood loss and closing off the hole in the vessel wall. Fig.4.16 shows a scanning electron micrograph of a blood clot. The fibrin strands (pink) have trapped red blood cells,platelets and a white blood cell. 4.Thrombolysis After the clot has formed, the process of removing it and healing the damaged blood vessel begins. The breakdown SECTION 2 Communication of fibrin, or fibrinolysis, is the first stage. Plasminogen,trapped within the clot as it forms, is converted to the enzyme plasmin by activators released from the damaged endothelial cells. Plasmin breaks down fibrin, progressively removing the clot to allow tissue repair to proceed. Control of coagulation The process of blood clotting relies heavily on several self-perpetuating processes- that is, once they have started,a positive feedback mechanism promotes their continuation.For example, thrombin is a powerful stimulator of its own production. Control and braking mechanisms are therefore essential to limit clotting to the affected area and terminate the process at the appropriate time. These include: The perfect smoothness of normal blood vessel lining prevents platelet adhesion in healthy, undamaged blood vessels. Activated clotting factors are rapidly deactivated by anticoagulants, such as heparin and antithrombin III. Activated clotting factors are quickly cleared from the blood by the liver. SPOT CHECK 3.Relate the main structural features of the red blood cell to its function. 4.Define diapedesis. 5.What is the difference between a platelet plug and a blood clot? Erythrocyte disorders After studying this section, you should be able to: define the term anaemia The blood CHAPTER 4 Anaemia may be asymptomatic. Signs and symptoms relate to the inability of the blood to supply body cells with enough oxygen, and may include pallor, fatigue and breathlessness on exertion. Additionally,the body's attempt to compensate for this can lead to: ·tachycardia -the heart rate increases to improve blood supply and speed up circlation compare and contrast the causes and effects of iron deficiency,megaloblastic,aplastic,hypoplastic and haemolytic anaemias explain why polycythaemia occurs. Anaemias Anaemia is the inability of the blood to carry enough oxygen to meet body needs. Usually, this is because there are low haemoglobin levels, but sometimes it is due to production of faulty haemoglobin. Anaemia is classified depending on the cause: ·Production of insufficient or defective erythrocytes -if the number of red blood cells being released is too low or the red blood cells are defective in some way, anaemia may result. Important causes include iron deficiency,vitamin B12/folic acid deficiency and bone marrow failure. Blood loss or excessive erythrocyte breakdown (haemolysis) -if erythrocytes are lost from the circulation, either through blood loss in haemorrhage or by accelerated haemolysis,anaemia can result. Anaemia can cause abnormal changes in red cell size or colour, detectable microscopically. Characteristic changes are listed in Table 4.3.Anaemia may be associated with a normal red cell count and no abnormalities of erythrocyte structure (normochromic normocytic anaemia). For example, following sudden haemorrhage,the red cells in the bloodstream are normal in size, shape and colour, but their numbers are fewer. Table 4.3 Terms used to describe red blood cell characteristics Term Definition Normochromic Cell colour normal Normocytic Cells normal-sized Microcytic Cells smaller than normal Macrocytic Cells bigger than normal Hypochromic Cells paler than normal Haemolytic Rate of cell destruction raised Megaloblastic Cells large and immature ·palpitations or angina pectoris (p.133) -caused by the increased effort of the overworked heart muscle. Iron deficiency anaemia This is the most common form of anaemia in many parts of the world. Dietary iron comes mainly from red meat,fortified cereals and highly coloured vegetables. Daily iron requirement in men is about 1-2mg. Women need 3 mg daily to counter blood loss during menstruation and to meet the needs of the growing fetus during pregnancy.Children require more than adults to meet their growth requirements.Normally, only about 10% of dietary iron is absorbed,although this increases in iron deficiency, such as following haemorrhage or in pregnancy. In iron deficiency anaemia, the red blood cell count is often normal, but the cells are small, pale and of variable size and they contain less haemoglobin than normal. The amount of haemoglobin in each cell is regarded as below normal when the mean cell haemoglobin (MCH) is less than 27 pg/cell (see Table 4.1).The anaemia is regarded as severe when the haemoglobin level is below 9 g/100 mL blood. Iron deficiency anaemia can result from deficient intake,unusually high iron requirements, or poor absorption from the alimentary tract. Deficient intake Because of the relative inefficiency of iron absorption,deficiency occurs frequently, even in individuals whose requirements are normal. It generally develops slowly over a prolonged period of time, and symptoms appear only once the anaemia is well established. The risk of deficiency increases if the daily diet is restricted in some way, as in poorly planned vegetarian diets, or in weight-reducing diets where the range of foods eaten is small. Babies dependent on milk may also suffer mild iron deficiency anaemia if weaning on to a mixed diet is delayed much past the first year,since the liver carries only a few months' store and milk is a poor source of iron. Other at-risk groups include older adults and the alcohol-dependent,whose diet can be poor. High requirements In pregnancy, iron requirements are increased to support both fetal growth and the additional load on the mother's cardiovascular system. Iron requirements also rise when there is chronic blood loss, the causes of which include peptic ulcers (p. 352),heavy menstrual bleeding (menorrhagia), 73