NU Master Doc PDF - Cell Biology of Neurons & Neurotransmission Exam 1
Document Details
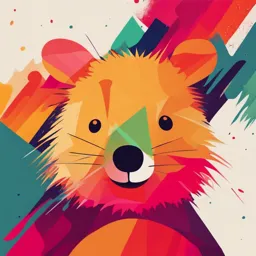
Uploaded by ProblemFreeCadmium2725
NU
Tags
Related
- Synapses and Synaptic communication 2023-2024 PDF
- Neuroscience 1 - Trans 1 - Nerves and Neurons PDF
- Neuroscience 1 - Trans 1 - Nerves and Neurons PDF
- Neuroscience 1 - Trans 4 - Neuron and Neuroglia PDF
- Neuro 355 Fundamentals of Behavioral Neurobiology Notes PDF
- Lecture 1 Notes - Brain and Behaviour - PSYC 304B 2024
Summary
This document provides a comprehensive overview of Cell Biology of Neurons and Neurotransmission, suitable for an undergraduate level. It includes definitions, concepts, and details on neurons, their functions, and types, along with a discussion of relevant processes.
Full Transcript
Exam Review Cell Biology of Neurons and Neurotransmission (Exam 1) Lecture 1: History & Intro to Neurons Definitions: Trephination: Surgical procedure that involves making a hole in the skull Ventricles: Cavities in the brain that produce and circulate cerebrospinal fluid (CSF)...
Exam Review Cell Biology of Neurons and Neurotransmission (Exam 1) Lecture 1: History & Intro to Neurons Definitions: Trephination: Surgical procedure that involves making a hole in the skull Ventricles: Cavities in the brain that produce and circulate cerebrospinal fluid (CSF) Human brain has 4 ventricles: left & right lateral ventricles, third ventricle, and fourth ventricle. Phrenology: The idea that different bumps on the skull could reveal our mental abilities & character traits Equipotentiality: Theory that different areas of the brain have the potential to take over the functions of damaged region in the brain ○ No single area is solely responsible for a specific cognitive function Golgi Staining: Technique in neuroscience where silver staining of a small percentage of neurons in a tissue sample is used to visualize nervous tissue under light microscopy. Synapse: Places where neurons connect and communicate with each other; allows transmission of electrical or chemical signals Nucleus: Cluster of neurons located within the central nervous system (CNS) deep within the cerebral hemispheres and brainstem ○ Work together to perform a specific function Ribosomes: Cellular structure found within neurons responsible for protein synthesis by translating the base sequence of an mRNA molecule into an amino acid sequence Cell Theory: All living organisms (including neurons) are composed of cells, with each cell acting as the basic unit of structure and function within the nervous system Neuron Doctrine: Nervous system is composed of individual cells, neurons, which are the fundamental structural and functional units ○ They communicate with each other through specialized junctions without directly fusing together Neurons: Nerve cells that send messages throughout the body to perform functions; makeup the brain and nervous system Axon: Long, thin nerve fiber that transmits electrical impulses (action potentials) away from a neuron’s cell body Neurite conveys information away from the soma, sometimes over long distances (sending information) Dendrite: Small branching extensions of a neuron that receives signals from other neurons (acts as input part of cell) – collects info from surrounding cells before transmitting into the cell body for further processing Neurite conveys information toward the cell body (receives information) Soma: Cell body (contains nucleus & organelles) integrates information, also the metabolic center Synaptic terminal: Bulb-shaped end of axon that converts electrical signals (action potential) into chemical signals (neurotransmitter release) Law of Dynamic Polarization: “Nerve impulses are exactly polarized in the neuron” “Within a neuron, electrical impulses travel in one direction only: from the dendrites and cell body, where signals are received, to the axon, which then transmits the signal to other neurons, meaning information always flows in a polarized manner from the input to the output of a neuron” Concepts: describe, define and/or differentiate: Aristotle v. Hippocrates view of brain’s purpose Aristotle: Believed the brain cooled the blood and that the heart was the center of thought and emotion. Hippocrates: Recognized the brain as the seat of intelligence, sensation, and emotions. Function of axon, dentrite, soma, axon terminal Axon: Transmits electrical impulses away from the soma to other neurons. Dendrite: Receives input (signals) from other neurons and relays them to the soma. Soma (Cell Body): Integrates incoming signals and maintains cell function. Axon Terminal: Releases neurotransmitters to send signals to the next neuron. Presynaptic v. postsynaptic neuron Presynaptic Neuron: Sends the signal via neurotransmitters into the synaptic cleft. Postsynaptic Neuron: Receives the signal via receptors on its dendrites or soma. Neurons v. glial cells Neurons: Primary cells for communication; transmit electrical and chemical signals. Glial Cells: Support neurons by providing nutrients, maintaining homeostasis, and insulating axons (e.g., myelin). Sensory v. motor v. internerouns Sensory Neurons: Carry signals from sensory organs to the central nervous system (CNS). Motor Neurons: Transmit signals from the CNS to muscles or glands. Interneurons: Connect sensory and motor neurons within the CNS for processing and reflexes. How modern neuroscience is a combination of Phrenology and equipotentiality Phrenology: Incorrectly localized functions to specific brain regions based on skull shape. Equipotentiality: Proposed all brain regions are equally capable of processing information. Modern Neuroscience: Combines these by acknowledging that while certain brain areas specialize in specific functions, neural plasticity allows compensation. Ramon y Cajal's contribution to neuroscience Established the neuron doctrine, proving that neurons are separate, discrete cells, not a continuous network. ○ Neuron Doctrine: Neurons are individual units, the basic building blocks of the nervous system. Defined the Law of Dynamic Polarization, showing signals flow in one direction: dendrites → soma → axon. ○ Law of Dynamic Polarization: Information flows directionally from dendrites to soma to axon to synapse. The parts of the neuron and what they do (functional boundaries) Dendrites: Receive signals from other neurons. Soma (Cell Body): Processes signals and supports cell metabolism. Axon: Transmits electrical impulses to target cells. Myelin Sheath: Insulates the axon, speeding up signal transmission. Nodes of Ranvier: Gaps in myelin where signal "jumps," increasing speed (saltatory conduction). Axon Terminal: Releases neurotransmitters to communicate with the next neuron. Note: "polarization" refers to the distinct structural asymmetry within a neuron, where one end of the cell develops into a long axon for transmitting electrical signals, while the other end forms multiple dendrites that receive signals, creating a clear separation of function within the neuron - Ability to initiate the formation of a structure that becomes a functional axon Lecture 2: Cell Biology of Neurons Definitions: Endoplasmic reticulum: A cellular organelle that plays a crucial role in protein synthesis, calcium signaling, and maintaining cellular homeostasis within neurons Regulates the release of calcium ions (vital for neurotransmitter release at synapses) ○ dynamic calcium store within the neuron and influencing various aspects of neuronal function like synaptic plasticity and axonal transport Golgi apparatus: A cell organelle that plays a crucial role in processing, sorting, and packaging proteins and lipids destined for transport within a neuron, particularly to the synapse Acts as a "post office" for the cell → ensures correct delivery of important molecules like neurotransmitters, receptors, and ion channels to their designated locations within the neuron Mitochondria: Small organelles within neurons = "powerhouse" of the cell; generate majority of cell's energy (ATP) ○ ATP needed to support neuronal activity: synaptic transmission, calcium homeostasis, regulating neuronal development, and maintaining overall cellular health Cytoskeleton: Complex network of protein fibers within a neuron ○ provides structural support, maintains the cell's shape, and facilitates the transport of essential components like organelles and neurotransmitters throughout the neuron's long, specialized extensions (axons and dendrites) 3 main types of filaments: microtubules, actin filaments (microfilaments), intermediate filaments (neurofilaments) ○ ensure proper neuronal function communication Plasma Membrane: Outer boundary of a neuron; separates the inside of the neuron from the extracellular fluid ○ Helps generate and transmit electrical signals → regulating the movement of ions across its surface Genotype: Internally coded, genetic blueprint (genetic potential) Phenotype: The outward physical manifestation (the extent to which its been expressed) Concepts: Kinesis v. dynein motor proteins Kinesin: Moves cargo toward the positive (+) end of microtubules (anterograde transport, away from the nucleus). Dynein: Moves cargo toward the negative (-) end of microtubules (retrograde transport, toward the nucleus). Anterograde v. retrograde transport Anterograde Transport: Movement of materials from the soma to the axon terminal (uses kinesin). Retrograde Transport: Movement of materials from the axon terminal back to the soma (uses dynein). Condensation reaction to form polypeptides A condensation reaction (dehydration synthesis) occurs when amino acids join by forming a peptide bond, releasing a water molecule. How proteins are made start to finish Transcription: DNA is copied into mRNA in the nucleus. mRNA Processing: Introns are removed, and a 5' cap and poly-A tail are added. Translation: Ribosomes read mRNA in the cytoplasm, tRNA brings amino acids, and a polypeptide is assembled. Post-Translational Modifications: Folding, cleavage, and chemical modifications occur for proper function. How many levels of structure can a protein have Primary: Sequence of amino acids. Secondary: Folding into α-helices and β-pleated sheets stabilized by hydrogen bonds. Tertiary: 3D structure formed by interactions between R-groups. Quaternary: Multiple polypeptides forming a functional protein complex. Defining characteristics of proteins/amino acids β-amyloid and neurofibrillary tangles/plaques (differences between brain injury and Alzheimer’s) Proteins: Made of amino acids, perform structural, enzymatic, and signaling roles. Amino Acids: Contain an amino group, carboxyl group, hydrogen, and variable R group. β-Amyloid Plaques: Extracellular deposits formed by the aggregation of β-amyloid proteins, seen in Alzheimer's disease. Neurofibrillary Tangles (NFTs): Intracellular tangles of hyperphosphorylated tau protein, also associated with Alzheimer's. Brain Injury vs. Alzheimer's: Brain injuries may cause temporary or localized tau tangles, while Alzheimer's involves widespread and progressive accumulation of plaques and tangles. Properties of the plasma membrane - Hydrophobic vs. Hydrophilic: Hydrophobic: Water-repelling, nonpolar tails of phospholipids. Hydrophilic: Water-attracting, polar heads of phospholipids. - Diffusion vs. Facilitated Diffusion: Diffusion: Movement of molecules from high to low concentration without assistance. Facilitated Diffusion: Movement via protein channels or carriers. - Active vs. Passive Transport: Active: Requires energy (ATP) to move molecules against the concentration gradient. Passive: No energy required; molecules move down their concentration gradient. - Endocytosis vs. Exocytosis: Endocytosis: Uptake of materials into the cell by vesicle formation. Exocytosis: Release of materials out of the cell by vesicle fusion with the plasma membrane. Lecture 3: Membrane Potentials Definitions: Selectively Permeable: The property of a neuron's cell membrane that allows certain ions or molecules to pass through while blocking others Electrochemical Gradient: Combined force driving the movement of ions across a neuron's cell membrane, resulting from both the difference in ion concentration (chemical gradient) and the difference in electrical charge (electrical gradient) between the inside and outside of the cell Driving force of Ion movement: Electrochemical gradient Ohm’s Law: l = g x V ○ l = current = movement of charge, + or - ○ g = conductance = pathway for ions to move ○ V = voltage = net driving force/electrical potential Nernst Equation: Calculates the equilibrium potential of a specific ion across a cell membrane Goldman equation: Solves for the membrane potential given conductance and equilibrium potential Conductance: The ability of charged particles, like ions, to move across a cell membrane Equilibrium potential: The membrane potential at which there is no net movement of a specific ion because its electrical and concentration gradients are balanced. Membrane potential: Electrical voltage difference across a neuron's cell membrane, created by the unequal distribution of ions between the inside and outside of the cell Crucial for transmitting neural signals Current: The movement of ions across a neuron's cell membrane, flow of electrical charges Na+/K+ Pump: A protein embedded in the cell membrane of neurons that actively transports sodium ions out of the cell and potassium ions into the cell Leak Channels: Ion channels that are always open, allowing ions to move passively across the cell membrane Concepts: Ion concentrations inside v. outside the neuron Inside the Neuron: High concentrations of K⁺ (potassium), low concentrations of Na⁺ (sodium) and Cl⁻ (chloride). Outside the Neuron: High concentrations of Na⁺ and Cl⁻, low concentrations of K⁺. Current v. membrane potential Current: Flow of charged ions across the cell membrane (measured in amperes). Membrane Potential (Vm): The difference in electrical charge across the plasma membrane (measured in millivolts, mV). Equilibrium potential v. resting membrane potential Equilibrium Potential (Eion): The membrane potential at which there is no net movement of a specific ion because its electrical and concentration gradients are balanced. Resting Membrane Potential (RMP): The steady-state voltage of the neuron, typically around -70 mV, maintained by ion gradients and selective permeability. Concentration v. electrical gradient Concentration Gradient: Difference in ion concentration across the membrane, driving diffusion. Electrical Gradient: Difference in charge across the membrane, driving ions toward opposite charges. Ionic basis of the resting membrane potential The RMP is primarily maintained by: ○ Potassium (K⁺) Leak Channels: K⁺ diffuses out of the cell, creating a negative charge inside. ○ Sodium-Potassium Pump (Na⁺/K⁺ ATPase): Actively pumps 3 Na⁺ out and 2 K⁺ in to maintain gradients. ○ Selective Permeability: Membrane is more permeable to K⁺ than Na⁺ or Cl⁻. How does changing ion concentrations (inside or outside of the cell) or conductance affect resting Vm Increasing Extracellular K⁺: Depolarizes the membrane (Vm becomes less negative). Increasing Extracellular Na⁺: Slight depolarization but limited due to low resting Na⁺ conductance. Decreasing K⁺ Conductance: Depolarizes the membrane. Increasing Na⁺ Conductance: Depolarizes the membrane. Increasing Cl⁻ Conductance: Hyperpolarizes the membrane (Vm becomes more negative). Lecture 4: Action Potentials Definitions: Action Potential (AP): An electrical impulse that travels down an axon, carrying information away from the cell body Rising Phase: Rapid depolarization of a neuron's membrane potential during an action potential Caused by the sudden opening of voltage-gated sodium channels Allows large influx of positive sodium ions into the cell → significant positive shift in the membrane voltage Falling Phase: Rapid decrease in membrane potential during an action potential where the neuron returns to its resting state after reaching its peak depolarization Caused by the closing of sodium channels and the opening of potassium channels Repolarization: Process that restores a neuron's resting membrane potential after an action potential Undershoot: Phase during an action potential: membrane potential of a neuron dips below its resting potential Becomes more negative after peak of AP has passed Refractory Period: Period following an AP where a neuron is unable to fire another AP (a state of recovery) Threshold: The minimum level of stimulus that causes a neuron to fire an action potential Tetrodotoxin (TTX): Potent neurotoxin that selectively blocks voltage-gated sodium channels on nerve cell membranes Concepts: Depolarization v. hyperpolarization Depolarization: Membrane potential becomes less negative (closer to 0 or positive), often due to Na⁺ influx. Hyperpolarization: Membrane potential becomes more negative than the resting membrane potential, usually due to K⁺ efflux or Cl⁻ influx. Voltage-gated v. ligand gated v. leak channels Voltage-Gated: Open/close in response to changes in membrane potential (e.g., voltage-gated Na⁺ and K⁺ channels). Ligand-Gated: Open/close when a specific chemical (ligand) binds to them (e.g., neurotransmitter receptors). Leak Channels: Always open, allowing passive movement of ions (e.g., K⁺ leak channels). Voltage gated sodium channel inactivation After opening during depolarization, voltage-gated Na⁺ channels inactivate due to a conformational change that blocks the pore, preventing further Na⁺ entry despite continued depolarization. Voltage gated sodium channels v. Voltage gated potassium channel kinetics Na⁺ Channels: Open and inactivate rapidly during depolarization. K⁺ Channels: Open more slowly and do not inactivate as quickly, contributing to repolarization and hyperpolarization. Positive feedback loops in an AP Depolarization → Na⁺ Channel Opening → Na⁺ Influx → Further Depolarization ○ This feedback loop accelerates the rising phase of the action potential. What interrupts the positive feedback loop of the AP Inactivation of Voltage-Gated Na⁺ Channels: Stops further Na⁺ entry. Opening of Voltage-Gated K⁺ Channels: Leads to repolarization. Ionic basis of the action potential 1. Resting State: a. Voltage-gated channels are closed; K⁺ leak channels maintain resting membrane potential (~-70 mV). 2. Depolarization (Rising Phase): a. Voltage-gated Na⁺ channels open → Na⁺ influx → Vm becomes less negative. 3. Peak of AP: a. Na⁺ channels inactivate; Vm approaches Na⁺ equilibrium potential (~+30 mV). 4. Repolarization (Falling Phase): a. Voltage-gated K⁺ channels open → K⁺ efflux → Vm becomes more negative. 5. Hyperpolarization: a. K⁺ channels remain open temporarily, overshooting the resting potential. 6. Return to Resting Potential: a. K⁺ channels close, Na⁺/K⁺ pump restores ionic gradients. 7. All-or-None Response: a. Once the threshold is reached, the AP always occurs with the same amplitude and duration. How voltage gated ion channels function during the AP Na⁺ Channels: Open during depolarization, inactivate at the peak. K⁺ Channels: Open during repolarization and remain open during hyperpolarization. What two events cause membrane repolarization during the AP Inactivation of Voltage-Gated Na⁺ Channels: Stops Na⁺ influx. Opening of Voltage-Gated K⁺ Channels: Increases K⁺ efflux. What is the effect of using ion channel blockers on the AP or currents (Na+ or K+) Na⁺ Blockers (e.g., Tetrodotoxin): Prevent depolarization, blocking AP initiation. K⁺ Blockers (e.g., TEA): Prevent repolarization, prolonging the AP. How does changing ion concentrations (inside or outside) or conductance affect the action potential Increased Extracellular Na⁺: Enhances depolarization (larger AP). Decreased Extracellular Na⁺: Reduces AP amplitude. Increased Extracellular K⁺: Depolarizes resting Vm, making APs easier to trigger. Increased K⁺ Conductance: Speeds up repolarization. Decreased Na⁺ Conductance: Slows depolarization, possibly preventing AP firing. Lecture 5: AP Conduction Definitions: Conduction Velocity: The speed at which an electrical impulse travels along a nerve fiber or other neural pathway. Membrane Resistance (Rm): The electrical resistance of a cell membrane to the flow of ions or electrical current. Key factor in electrodialysis processes and in understanding how cells respond to stimuli. Membrane Capacitance: The electrical charge stored in a biological membrane Internal Resistance (Ri): The resistance of the axoplasm, or the resistance to the flow of charges along the inner surface of an axon. Myelination: The process of forming a fatty-protein sheath around nerve cell axons. Nodes of Ranvier: Characterized by short (1um), specialized regions in the axonal membrane that are not insulated by myelin. Saltatory Conduction: The rapid transmission of nerve impulses along a myelinated axon, where the electrical signal jumps from one node of Ranvier to the next ○ ("leaping" over the myelinated sections) significantly speeding up the conduction of the action potential compared to unmyelinated axons Central Nervous System (CNS): Network of neurons in the brain and spinal cord that control physiological functions. Concepts: Invertebrate vs. Vertebrate Solution for increasing AP conduction Invertebrates: Increase axon diameter, reducing internal resistance (Ri) to ion flow, thereby increasing conduction speed. Vertebrates: Use myelination to insulate axons, increasing membrane resistance (Rm) and reducing capacitance (Cm), leading to faster AP conduction. Relative conduction speed of unmyelinated vs. Myelinated axons Unmyelinated Axons: Slow conduction (continuous propagation as the entire membrane must depolarize). Myelinated Axons: Faster conduction (saltatory propagation, where AP jumps between nodes of Ranvier). Location of sodium channels vs. potassium channels myelinated axons Sodium Channels: Concentrated at the nodes of Ranvier for depolarization. Potassium Channels: Also at the nodes of Ranvier but may have lower density compared to sodium channels. Astrocytes vs. Schwann Cells Astrocytes (CNS): Provide metabolic support, regulate neurotransmitter levels, maintain the blood-brain barrier, and influence synaptic activity. Schwann Cells (PNS): Myelinate axons in the peripheral nervous system, enabling faster AP conduction and supporting axonal repair. How does myelination speed up the action potential? Increased Membrane Resistance (Rm): Reduces current leakage, keeping the signal strong. Decreased Membrane Capacitance (Cm): Less charge storage at the membrane allows faster changes in voltage. Saltatory Conduction: AP jumps between nodes of Ranvier, reducing the distance the current must travel. How do membrane properties (Cm, Rm, Ri) affect action potential propagation? Membrane Capacitance (Cm): Lower capacitance speeds up depolarization (myelination decreases Cm). Membrane Resistance (Rm): Higher resistance reduces current loss, improving conduction efficiency (myelination increases Rm). Internal Resistance (Ri): Lower resistance (larger axon diameter) enhances ion flow and increases conduction speed. Lecture 6: Multiple Sclerosis (MS) Concepts: Symptoms: Vision problems (blurred or double vision) Muscle weakness and spasticity Numbness or tingling in limbs Fatigue Loss of coordination and balance Cognitive issues (memory or concentration problems) Bowel and bladder dysfunction How and why is AP conduction affected? Cause: Demyelination of axons in the CNS due to the immune system attacking myelin. Effect: Loss of insulation increases current leakage, reducing membrane resistance (Rm). Slower AP conduction or conduction failure (no AP propagation). Disruption of saltatory conduction as sodium channels are not evenly distributed along the axon. How can partial recovery occur? Remyelination: Oligodendrocytes can partially regenerate myelin. Sodium Channel Redistribution: Sodium channels may spread along the demyelinated segments, allowing AP conduction without saltatory propagation. What does MRI tell us about MS? Detects lesions or plaques in the CNS where demyelination has occurred. Can distinguish between active (inflammatory) and inactive lesions. Provides insights into disease progression and treatment effectiveness. Why are Ca2+ channel blockers used as treatment? Reduce neuronal excitotoxicity caused by excessive calcium influx during inflammation. Help prevent further damage to neurons and glial cells. What factors affect susceptibility? Genetic Factors: Specific HLA gene variants increase risk. Environmental Factors: Vitamin D deficiency, viral infections (e.g., Epstein-Barr virus). Gender: Women are more susceptible. Lifestyle Factors: Smoking and stress may contribute. Why is it necessary for synaptic vesicle transmission? Calcium influx through voltage-gated Ca²⁺ channels triggers synaptic vesicle fusion with the presynaptic membrane, releasing neurotransmitters into the synaptic cleft. The mechanism of Botox v. Tetanus What happens at the NMJ in the presence of Botox? Botox (Botulinum Toxin): Blocks acetylcholine (ACh) release by cleaving SNARE proteins, preventing vesicle fusion and leading to muscle paralysis. Tetanus Toxin: Blocks inhibitory neurotransmitter (GABA and glycine) release by cleaving SNARE proteins in interneurons, leading to continuous muscle contraction (spasticity). Myasthenia Gravis: Symptoms ○ Muscle weakness (worsens with activity, improves with rest) ○ Drooping eyelids (ptosis) ○ Difficulty swallowing and speaking ○ Fatigue during repetitive movements Is the disruption by auto-antibodies presynaptic or postsynaptic? ○ Postsynaptic: Auto-antibodies target nicotinic ACh receptors at the NMJ. How is the synapse affected and how does this relate to treatments? ○ Effect: Fewer functional ACh receptors lead to reduced synaptic transmission and muscle weakness. ○ Treatments: Acetylcholinesterase Inhibitors (e.g., Pyridostigmine): Increase ACh availability by preventing its breakdown. Immunosuppressants: Reduce the immune attack on ACh receptors. Plasmapheresis/IVIG: Remove or neutralize auto-antibodies. Lecture 7&8: Synaptic Transmission Definitions: Affinity: The strength of the binding interaction between a molecule, like a neurotransmitter or drug, and its target receptor on a neuron Potency: The gene activation potential within a cell Begins with totipotency to designate a cell with the most differentiation potential. Agonist: Drug or substance that binds to a receptor in the brain and mimics the action of a neurotransmitter or hormone Produces cellular response that activates the receptor Antagonist: Substance that blocks the activity of a receptor, preventing it from being activated by an agonist. Ligand: A molecule that binds to a specific receptor on a neuron, triggering a cellular response by initiating a signal transduction pathway Includes neurotransmitters like dopamine, serotonin, or glutamate Exogenous: Something originating from outside the body, relating to stimuli or influences that come from the external environment. Endogenous: Something that originates within the body or nervous system itself (substance, process, or activity produced by body naturally) Neurotransmitter: A chemical molecule released by a neuron that transmits signals to another cell, acting as a chemical messenger between neurons (communicate across the synaptic cleft) Neuromuscular Junction: A specialized synapse where a motor neuron communicates with a muscle fiber. ○ Crucial role in voluntary muscle contraction Synaptic connection between the terminal end of a motor nerve and a muscle Synaptic Vesicle: A small, membrane-bound sac located within the presynaptic terminal of a neuron that stores neurotransmitters and releases them into the synaptic cleft when stimulated by an electrical signal. SNARE complex (syntaxin, SNAP25, synaptotagmin, synaptobrevin) Function: Facilitates synaptic vesicle fusion and neurotransmitter release. Components: ○ Syntaxin: Located on the presynaptic membrane, anchors the complex. ○ SNAP25: Binds syntaxin and synaptobrevin, forming the SNARE core complex. ○ Synaptotagmin: Calcium sensor that triggers vesicle fusion upon Ca²⁺ influx. ○ Synaptobrevin (VAMP): Located on synaptic vesicles, interacts with SNAP25 and syntaxin. Mechanism: ○ SNARE complex assembles to bring vesicles and membranes close ○ Calcium influx binds synaptotagmin. ○ Conformational change triggers vesicle fusion and neurotransmitter release. Acetylcholinesterase: An enzyme primarily located at the synaptic cleft that rapidly breaks down the neurotransmitter acetylcholine (ACh), terminating its signal and allowing for the recycling of the neurotransmitter. Acetylcholine Receptor: A protein on the surface of a cell that binds to the neurotransmitter acetylcholine, triggering a response within the cell Specialized channel that opens when acetylcholine binds, allowing ions to flow into the cell and generate an electrical signal, playing a key role in muscle contraction and various brain functions (memory and learning) GABA Receptor: A protein complex on the surface of neurons that responds to the neurotransmitter gamma-aminobutyric acid (GABA), acting as the primary inhibitory receptor in the central nervous system. Decreases activity of a neuron when activated Brain areas for memory formation and storage: hippocampus – memory consolidation; especially spatial/declarative ○ In medial temporal lobe of brain, plays a crucial role in memory and spatial navigation Concepts Local v. Long Distance signaling Local Signaling: Communication between nearby cells (e.g., synaptic signaling, paracrine signaling). Long Distance Signaling: Communication across the body (e.g., endocrine signaling through hormones). Agonist v. Antagonist Agonist: Activates a receptor to produce a biological response. Antagonist: Blocks a receptor, preventing its activation. Endogenous v. exogenous ligands Endogenous: Naturally produced within the body (e.g., dopamine). Exogenous: Introduced from outside the body (e.g., morphine). Excitatory v. Inhibitory Postsynaptic Potentials EPSPs: Depolarize the postsynaptic membrane, increasing the likelihood of an action potential (e.g., Na+ influx). IPSPs: Hyperpolarize the postsynaptic membrane, decreasing the likelihood of an action potential (e.g., Cl- influx). Locations of excitatory v. Inhibitory Synapses Excitatory: Often located on dendritic spines. Inhibitory: Often found on the soma and axon hillock. Spatial v. temporal summation Spatial Summation: Multiple signals from different locations combine at the postsynaptic membrane. Temporal Summation: Multiple signals from the same presynaptic neuron arrive in quick succession. Postsynaptic potentials v. action potentials Postsynaptic Potentials: Graded, local changes in membrane potential; can be depolarizing or hyperpolarizing. Action Potentials: All-or-none depolarizations that propagate along the axon. Describe the order of steps leading to transmitter release and how transmitters can change postsynaptic membrane potentials 1. Action potential arrives at the axon terminal. 2. Voltage-gated Ca²⁺ channels open. 3. Ca²⁺ influx triggers vesicle fusion with the presynaptic membrane. 4. Neurotransmitter release into the synaptic cleft. 5. Neurotransmitter binds to postsynaptic receptors. 6. Changes in postsynaptic membrane potential (EPSP or IPSP). How can the same neurotransmitter have both excitatory and inhibitory effects? Depends on the type of receptor it binds to: ○ Excitatory: If it binds to a receptor that opens Na+ channels (e.g., nicotinic ACh receptors). ○ Inhibitory: If it binds to a receptor that opens Cl- channels (e.g., GABA receptors). How benzodiazepines and barbiturates act as modulators on the GABA receptor? Benzodiazepines: Increase GABA receptor activity by enhancing the frequency of Cl- channel opening. Barbiturates: Increase the duration of Cl- channel opening, leading to stronger inhibitory effects. When does calcium enter the axonal terminal? When an action potential opens voltage-gated Ca²⁺ channels in the axon terminal. Procedural v. declarative memory Procedural: Implicit memory for skills and actions (e.g., riding a bike). Declarative: Explicit memory for facts and events (e.g., recalling a birthday). Conditioned v. unconditioned stimulus Conditioned Stimulus: A previously neutral stimulus that elicits a response after conditioning. Unconditioned Stimulus: Naturally triggers a response without prior conditioning. Synaptic depression v. facilitation Depression: Decreased neurotransmitter release with repeated stimulation. Facilitation: Increased neurotransmitter release with repeated stimulation. Sensitization v. Habituation Sensitization: Increased response to a repeated stimulus. Habituation: Decreased response to a repeated stimulus. AMPA v. NMDA receptors: Different gating properties ○ AMPA: Opens with glutamate binding alone. ○ NMDA: Requires both glutamate binding and membrane depolarization to relieve Mg²⁺ block. Activation allows what different ions to enter the neuron ○ AMPA: Na+ and K+. ○ NMDA: Na+, K+, and Ca²⁺ LTP v. LTD LTP (Long-Term Potentiation): Strengthening of synaptic connections with high-frequency stimulation. LTD (Long-Term Depression): Weakening of synaptic connections with low-frequency stimulation. The two effects of NMDA receptor activation Activation depends on frequency stimulation of postsynaptic neuron Calcium entry does what two things to AMPA receptors? ○ Calcium Entry: Activates intracellular signaling pathways ○ Effects on AMPA Receptors: Insertion of AMPA Receptors: Strengthens synaptic transmission (LTP). Removal of AMPA Receptors: Weakens synaptic transmission (LTD). Spatial learning assays in rats: Water maze v. radial arm maze ○ Water Maze: Rats navigate a pool to find a hidden platform; tests spatial memory and hippocampal function. ○ Radial Arm Maze: Rats explore arms of a maze to find food rewards; tests working and reference memory. Lecture 8&9: Learning & Memory Definitions: Learning: A relatively lasting change in behavior that is the result of experience Process of creating and strengthening connections between neurons in brain (causing physical changes to brain structure) Memory: The process of acquiring, storing, and retrieving information (cognitive function) Classical Conditioning: A fundamental learning process where a neutral stimulus becomes associated with a naturally occurring stimulus, leading to a learned automatic response to the previously neutral stimulus. Applied in immunology by associating neutral stimuli (NS) with immune-modulating agents (unconditioned stimuli, UCS). Over time, the NS becomes a conditioned stimulus (CS) capable of triggering immune responses (conditioned response, CR) without the UCS. (definition from Psych 101) Conditioned Stimulus: A previously neutral stimulus that through repeated association with a naturally occurring stimulus (unconditioned stimulus), eventually elicits a learned response (conditioned response) (becomes trigger for a specific behavior due to the learned association) Unconditioned Stimulus: A stimulus that naturally and automatically triggers a response without any prior learning required Elicits innate reaction Hebb’s Postulate: “When an axon of cell A is near enough to excite a cell B and repeatedly or persistently takes part in firing it, some growth process or metabolic change takes place in one or both cells such that A’s efficiency, as one of the cells firing B, is increased.” Easy → “Cells that fire together, wire together” Fundamental concept: when two neurons are repeatedly activated close together in time, the connection between them strengthens, making it more likely for one neuron to fire the other in the future (forming basis for learning and memory) Coordinated activity of presynaptic and postsynaptic neuron strengthens the synapse Magnesium Block: The action of magnesium ions (Mg2+) blocking the N-methyl-D-aspartate (NMDA) receptor, a type of glutamate receptor crucial for learning and memory, by occupying a site within the receptor's ion channel, preventing excessive calcium influx (natural regulator) Glutamate Receptor: A protein located on the surface of neurons that binds to the neurotransmitter glutamate, triggering an excitatory signal within the neuron, making it more likely to fire an action potential major excitatory neurotransmitter in the CNS Sensitizatization: A process where repeated exposure to a stimulus leads to an amplified response (nervous system becomes increasingly sensitive) Habituation: Occurs when repeated exposure to a given stimulus leads to a decline in individual response Decrease in behavioral response to a repeated stimulus Long-term Potentiation (LTP): Long-lasting increase in the strength of connections between neurons Long-term Depression (LTD): A synaptic plasticity mechanism that decreases the efficiency of synaptic transmission over time. Neural Plasticity: The brain’s ability to change its structure and function in response to new experiences, learning, or environmental stimuli. Concepts: What neural mechanisms underlie memory formation? Synaptic Plasticity: Strengthening or weakening of synapses (e.g., LTP and LTD). Hebbian Learning: "Neurons that fire together, wire together" principle. Neurogenesis: Formation of new neurons, particularly in the hippocampus. Neurotransmitter Release: Glutamate and dopamine play critical roles in learning and memory. Protein Synthesis: Required for long-term memory storage.