NP Exam1 Shortened.docx
Document Details
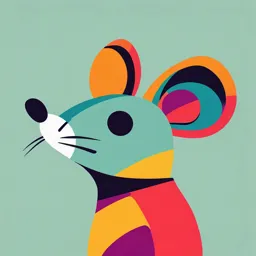
Uploaded by SensibleConnemara7909
Full Transcript
Introduction to the pharmacology of CNS drugs The fundamental cell of the nervous system is the neuron. The cell body (soma) of the neuron houses the nucleus and is also called the soma. The neuron also has processes: axons, which receive messages and the dendrites, which sends messages. The axo...
Introduction to the pharmacology of CNS drugs The fundamental cell of the nervous system is the neuron. The cell body (soma) of the neuron houses the nucleus and is also called the soma. The neuron also has processes: axons, which receive messages and the dendrites, which sends messages. The axon hillock (initial segment) is the area where messages are initiated, lying close the soma. The messages are carried down to the terminus. Structural classes of neurons Multipolar have more than 2 process(es) off the cell body (ex. motor neurons). Bipolar have 2 process(es) off the cell body. Pseudo-unipolar have 1 process off the cell body. Define the function of each glial cell (more than just glue): Astrocytes (looks like a star): supporting cell that is responsible for regulating neuronal micro-environment; role in BBB Schwann cells: Peripheral nervous system; myelinating cells around axons of neurons Oligodendrocytes: Found in CNS, myelinating cells around axons of neurons Microglial cells: only in CNS, resident immune cells Ependymal cells: secrete + circulate CSF Primary active transport: requires energy directly from ATP, moving things against the gradient (low concentration to high concentration) Na+/K+ pump and ATPase are important in establishing ionic gradients across the membrane of cells, including neurons Intracellular fluid: Low Na+, High K+; Extracellular fluid: High Na+, Low K+ WITHOUT a pump, Na+ would move down the concentration gradient INTO the cell (high to low) and K+ would move out of the cell WITH a pump + 1 ATP ion – this pump is constitutively active ATP attaches to the pump while it loads 3 Na+ ADP detaches to activate the pump (open), a phosphate is left behind 3 Na+ out of the cell 2K+ loads into the pump, phosphate detaches 2 K+ into the cell Facilitated diffusion is passive, so moves with the gradient. Ion channel charge or size specific Important sites of action: action potential, synaptic transmission Voltage-gated channel Ligand-gated channel In addition to concentration gradient, electrochemical gradient will also determine the flow or movement of ions across a membrane. Each ion has an equilibrium potential – all forces that control ion movement are balanced. Nernst equation is used to calculate this (impacted by gas, temperature, faraday constant, ion valence, and concentrations inside and outside the cell). K= -90mV, Na= 72 mV, Cl= -89.2mV Sodium, potassium, and chloride contribute to the resting membrane potential in neurons. This is calculated using the Goldman-Hodgkin-Katz equation (impacted by membrane potential voltage, permeability coefficient) In this equation, Cl is flipped (in/out) compared to K+ and Na+ (out/in) because it has a negative charge. Potassium leak channels have the greatest influence on resting membrane potential Resting membrane potential= -73.2mV Equilibrium potential for an ion = membrane potential that would occur if membrane was permeable to only that ion If K channels open in the membrane, K+ would move OUT to make the cell more negative towards -90mV If Na channels open in the membrane, Na+ would move IN to make the cell more positive to increase membrane potential towards 72 mV If Cl channels open in the membrane, Cl- would move IN to make the cell more negative to decrease the membrane potential towards -89.2 Driving force for ion movement is related to the DIFFERENCE between equilibrium potential and membrane potential. Large differences create a steep gradient that favors ion movement Membrane potential (-70)l: A cell is “polarized” because its interior is more negative than its exterior. Depolarization occurs when ion movement (Na+ moves into the cell) reduces the charge imbalance (becomes more positive). Overshoot refers to the development of a charge reversal (inside becomes more positive than outside) Repolarization is movement back towards resting potential Hyperpolarization is the development of even more negative charge inside the cell (K+ moving out of the cell, gates are slow to close). Action potentials: When do voltage-gated K+ channels open? Voltage-gated K+ channels open at the peak of the AP, to let K+ out of the cell and to repolarize/hyperpolarize. These open and close slowly. Voltage-gated Na+ channels have 2 gates: activation and inactivation. The activation gates are closed at resting and open at threshold and the inactivation gate closes (rapidly) at peak. The absolute refractory period is the period of time following an AP when the neuron cannot fire. It is due to the inactivated state of Na+ channels (ensures one direction of AP flow). The relative refractory period is the time following an AP when the neuron can fire, but threshold is higher and the AP isn’t as strong (stronger stimulus, higher threshold, weaker action potential or lower peak). Outline the steps that leads to release of neurotransmitter. An action potential reaches the terminal. Voltage-gated calcium channels open in the axon terminus. Calcium ions enter the presynaptic cell. The rise in calcium ions causes vesicles that contain neurotransmitters to fuse with the plasma membrane. Neurotransmitters are released into the synaptic cleft. Other than diffusion away, neurotransmitters may be turned off via uptake into a presynaptic cell (reuptake) or glial cell (regulates neuronal micro-environment, astrocytes) or degradation via an enzyme. Types of receptors: Ionotropic receptors are ligand-gated ion channels (gated by binding of a ligand, in this case NT). Metabotropic receptors are G-protein coupled receptors (can regulate ion channels, receptor itself is not an ion channel; have the ability to influence more than just ion movement across the movement because sometimes their target is an enzyme) Postsynaptic responses: EPSPs (excitatory post-synaptic potentials) are depolarizing changes in the membrane potential that make it more likely an AP will fire. Ex.: sodium channels opening or potassium ion channels closing IPSPs (inhibitory post-synaptic potentials) are hyperpolarized changes in the membrane potential that make it less likely an AP will fire. Ex.: potassium channels opening, chloride channels opening) Summation (single EPSP can’t get us to an AP) Temporal summation is summation in time Spatial summation is summation in space (same vicinity) Presynaptic receptors When presynaptic receptors increase activity of voltage-gated Ca2+ channels, then presynaptic facilitation occurs. When presynaptic receptors decrease activity of voltage-gated Ca2+ channels, then presynaptic inhibition occurs. Auto-receptors are receptors located on a neuron that bind the neurotransmitter released by that neuron. Ionotropic: ligand gated Metabotropic: G-coupled receptors