Ciliary Body Musculature PDF
Document Details
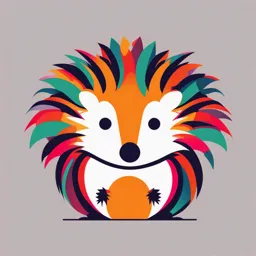
Uploaded by AdroitMossAgate
Tags
Summary
This document describes the ciliary body musculature in mammals. It categorizes the musculature based on different animal groups such as herbivores, carnivores, and anthropoids. The document provides detailed descriptions of the muscle types and their functions.
Full Transcript
Ciliary Body Musculature The CBM is comprised of smooth muscle fibers in mammals. Contraction of the CBM draws the ciliary processes and body both forward and inward, thus relaxing the lenticular zonules (suspensory ligament of the lens) and altering the shape and refraction of the lens. This muscle...
Ciliary Body Musculature The CBM is comprised of smooth muscle fibers in mammals. Contraction of the CBM draws the ciliary processes and body both forward and inward, thus relaxing the lenticular zonules (suspensory ligament of the lens) and altering the shape and refraction of the lens. This muscle is often weakly developed in many nonprimate species and as a result, offers poor accommodative ability. On the basis of ciliary body musculature development, the placental mammalian ICA has been categorized into three main groups: the herbivorous, the carnivorous, and the anthropoid (Henderson, 1926; Tripathi, 1974). The categorization depicted in Fig. 2.47 was originally inspired from observations of the ciliary regions of the dog, pig, and ape (Duke‐Elder, 1958). The herbivorous type has been characterized as the most common and primitive in orders of mammals up to and including ungulates. This type of angle consists of an inner layer of connective tissue that forms a baseplate of the ciliary body and extends from the root of the iris to the ora ciliaris retinae. It also consists of an outer layer of smooth muscle that presses against the sclera externally and runs meridionally from the corneoscleral junction toward the ora ciliaris retinae (Fig. 2.47A and Fig. 2.48A). The two layers are often referred to as “leaves” that separate anteriorly and form the ciliary cleft. The ciliary cleft is then a triangular area that varies both in depth (i.e., length) and height, and functionally may be considered a posterolateral extension of the anterior chamber into the ciliary body. Historically, this region was called the cilioscleral sinus, but because it neither separates the ciliary body from the sclera nor is a part of the ciliary venous sinus, the term cilioscleral sinus has been replaced with ciliary cleft (Duke‐Elder, 1958; Tripathi, 1974; Troncoso, 1938). The ciliary cleft is an area containing wide spaces filled with aqueous humor and interspersed with cell‐lined cords of connective tissue. The spaces between the fibrous cords were initially described in cattle and horses, and they have been often referred to as Fontana’s spaces (Samuelson, 1996). The carnivorous type possesses a bi‐leaflet configuration as well, but the fibrous inner leaf or layer is usually replaced by meridionally oriented smooth muscle and some radially oriented muscle fibers (see Fig. 2.47 and Fig. 2.48B) (Duke‐ Elder, 1958). Similar to the herbivorous type, the two leaves separate anteriorly and hold a wide, deep ciliary cleft. In both the herbivorous and carnivorous types, the ciliary cleft offers little support to properly anchor the iris. Compensation for wide and deep ciliary clefts is provided by a series of pectinate ligaments attaching the anterior iridal root and inner ciliary baseplate to the limbal cornea. The anthropoid type differs sharply in its 30 configuration compared with the other types (see Fig. 2.47C and Fig. 2.48C). The ciliary body musculature of primates is believed to be the most highly developed among mammals. The muscle, which has three components (i.e., radial, meridional, and circular), forms a large, anterior pyramidal structure that provides a strong baseplate for iridal attachment. The anterior portion of the CBM has replaced both the ciliary cleft, which barely exists in the anthropoid angle, and the pectinate ligaments, which vestigially consist of scattered iridal processes. In addition to the three basic forms of CBM and ICA, three more configurations have been described among nonprimate mammals (Fig. 2.49; Samuelson, 1996). Among herbivores, two other types have been identified: the small diurnal herbivore and the large diurnal herbivore. In the small diurnal herbivore, such as the squirrel, a deep ciliary cleft is lined by inner and outer leaves of fibrous tissue and smooth muscle of the ciliary body. In the large diurnal herbivore, such as the pig, there is a well‐developed ciliary cleft and outer leaf of ciliary muscle, which anteriorly includes circularly oriented muscle embedded within a scleral spur. The scleral spur is a small ridge of dense connective tissue that separates the muscle fibers from the posterior ICA. TEM of different ungulates has revealed that many of the muscle fibers, especially those located anteriorly, course more circumferentially than meridionally (Samuelson & Lewis, 1995). This difference in orientation of the musculature is most evident in the pig and water buffalo. In these animals, circular muscle fibers are located near the innermost portion of the posterior end of the ciliary cleft. A third type occurs in “high” accommodative carnivores, such as raccoons and ferrets. In this configuration, the anterior CBM is highly developed but not as an outer leaflet. Instead, the anterior musculature extends as a single leaflet either internally (i.e., raccoons) or intermediately (i.e., ferrets) (Fig. 2.49C and Fig. 2.50). In birds and other nonmammalian species, the CBM consists of skeletal muscle cells that are primarily meridional. At least two distinct muscle bundles are located in this region of the avian eye: an anterior bundle, which is known as the muscle of Crampton, arises near the corneal margin; and a posterior bundle, which is known as Brücke’s muscle. In birds such as the hawk, Brücke’s muscle is well developed and sometimes is referred to as two muscles: Müller’s (anterior) and Brücke’s (Duke‐Elder, 1958). Contraction of Brücke’s muscle causes the ciliary body to push against or compress the lens, thus deforming it, whereas contraction of Crampton’s muscle alters the shape of the cornea by shortening its radius of curvature. 31 Ciliary Body Vasculature The blood supply of the ciliary body derives from the two long posterior ciliary arteries and the anterior ciliary arteries. As the long posterior arteries pass into the suprachoroidal space equatorially along the lateromedial horizontal plane, they undergo several divisions. These divisions anastomose anteriorly with branches of the anterior ciliary arteries to form the major arterial circle, which is located either in the base of the iris or the anterior ciliary body (see Fig. 2.37 and Fig. 2.38). The anterior ciliary arteries, which arise from branches of the ophthalmic artery, typically enter the globe at the attachment sites for the recti muscles and help to supply the ciliary muscles (Streeten, 1988). The major arterial circle is the primary vasculature supply of the ciliary processes. Numerous anatomic variations of this vasculature have been found among mammals (Funk & Rohen, 1990; Matsuo, 1973; Morrison & Van Buskirk, 1983; Morrison et al., 1987a, 1987b; Natiello & Samuelson, 2005). In primates and rabbits, two types of arterioles supply the major and minor processes, whereas in other species, a single type of arteriole originates from the major arterial circle and supplies the ciliary process vasculature (Morrison & Van Buskirk, 1983; Morrison et al., 1987a, 1987b; Natiello & Samuelson, 2005). Discrete interspecies variations occur in the angioarchitecture of the ciliary processes (Fig. 2.51). Rodents, rats and Guinea pigs have extensive inter-process connections and concentrically parallel capillaries that are irregularly dilated and travel posteriorly, emptying into the anterior most choroidal veins. Carnivores such as dogs and cats have processes supplied by one arteriole that is directed posteriorly throughout its length, with capillary arcades that extend to each process margin, from which they empty into venous sinuses. The mammalian CBM is supplied by parasympathetic fibers from the oculomotor nerve and by sympathetic nerve fibers. The parasympathetic fibers leave the oculomotor nerve, penetrate the ventral oblique muscle, and synapse in the ciliary ganglion. From the ciliary ganglion, short ciliary nerves penetrate the sclera around the optic nerve to pass into the sclera and suprachoroidal space innervating the ciliary muscle and iris muscles. The sympathetic fibers arrive via the long ciliary nerves from the dorsal or superior cervical ganglia in a similar manner (Gum et al., 2007). 32 Iridocorneal Angle Aqueous humor is produced by the ciliary body epithelium and enters the posterior chamber before flowing through the pupil into the anterior chamber. In the conventional outflow pathway, aqueous humor exits the eye through the corneoscleral trabecular meshwork. The anatomy of the aqueous humor outflow system has been extensively studied in humans, nonhuman primates, dogs, cats, rabbits, horses, and other ungulates. This system primarily consists of the ICA, which is bounded anteriorly by the peripheral cornea and perilimbal sclera, and posteriorly by the peripheral iris and anterior CBM. From amphibians to higher mammals, the ICA consists of an irregular, reticular network of connective tissue beams called trabeculae that are lined partially or entirely by a single layer of cells (Samuelson, 1996; Tripathi, 1971a, 1974). The size of the ICA varies among species. In dogs of different ages and breeds that had undergone cataract surgery, the size of the ICA as determined by the angle opening distance (the distance between the internal limbus and the base of the iris) using ultrasound biomicroscopy was found to vary considerably (Crumley et al., 2009). At the expense of the CBM, a proportionally larger sinus is found in most domestic animals than in humans. The pectinate ligaments consist of long strands anchoring the anterior base of the iris to the inner peripheral cornea (Fig. 2.52 and Fig. 2.53). In the dog and cat, these strands are usually slender and widely separated from each other, thus making it difficult to visualize histologically an intact pectinate ligament fiber for its entire length. In the rabbit and pig, the strands are somewhat shorter and thicker than those of domestic carnivores (Simones et al., 1996). In contrast, most ungulates possess moderately broad to very stout pectinate ligaments (Fig. 2.54). The pectinate ligaments are entirely lined by cells that are confluent with the anterior surface of the iris. In ICAs with stout pectinate ligaments, the anterior chamber freely communicates with the ICA through pores that lead into a collection of small channels surrounded by cords of densely packed collagen. Posteriorly, the pectinate ligament anastomoses with anterior beams of the trabecular meshwork. In mammals, the network of trabeculae is usually subdivided into two regions. The uveal trabecular meshwork, which in most animals comprises most of the inner ICA area, thus forming the ciliary cleft, and the corneoscleral trabecular meshwork, which is similar in construction to the uveal meshwork but smaller both in size of the trabecular beams and the channels or spaces between the cell‐lined beams. The uveal meshwork interconnects the inner, anterior CBM with the pectinate ligament. For the most part, the uveal trabeculae are oriented meridionally. In most animals, these beams are completely encased by an 33 endothelium referred to as trabecular cells. The beams branch increasingly toward the CBM in radial and circular directions. As a result, the posterior uveal trabeculae are smaller, with smaller intertrabecular spaces separating them. The posterior uveal meshwork often merges imperceptibly with the posterior, innermost regions of the corneoscleral trabecular meshwork. The corneoscleral trabecular meshworks of domestic animals are characterized mainly by small trabeculae separated by small intertrabecular spaces. In carnivores, these trabeculae are incompletely lined by trabecular cells (Fig. 2.55). By comparison, in certain ungulates, including horses, bison, and water buffalo, each trabecula is completely lined by trabecular cells. In ruminants and pigs, individual trabeculae can be completely lined, whereas others are incompletely lined. Composition of the trabeculae varies very little among species. The core, or center, of each beam is made up of circularly and meridionally oriented collagen fibers interspersed with a modified elastin (Gong et al., 1989). The core is usually enveloped by a cortical zone consisting of amorphous, granular material surrounded by basement membrane‐like material. In cats, horses, and water buffalo, the larger corneoscleral trabeculae and smaller uveal trabeculae have multiple layers of basement membrane‐like material within the cortical zone (Samuelson et al., 1989; Tripathi, 1974). In horses, a narrow zone of thickened, rounded, corneoscleral trabeculae is present between the outflow veins of the ICA and the pectinate ligament. These trabeculae possess no basement membrane‐like material; instead, they have long‐spacing collagen. Trabecular cells are similar across species, being fibroblast‐ like with slender cell processes that attach to adjacent cells and their processes. These processes allow the corneoscleral trabecular meshwork to act as a sieve, thus reducing the size of the particles that can move into the meshwork (Garcia et al., 1986; Johnson et al., 1990; Samuelson et al., 1985; Smith et al., 1986). The degree of meshwork porosity varies between species, with the dog having a more porous meshwork when compared with the horse. The trabecular cell also has the ability to ingest a wide variety of particles, which can range greatly in size (Samuelson et al., 1984b). The phagocytic‐like quality of the trabecular cell provides the ICA with an indigenous clearance mechanism for debris, thus reducing possibilities for an inflammatory response (Grierson & Lee, 1973; Sherwood & Richardson, 1981). An operculum is located within the canine corneoscleral trabecular meshwork (Samuelson et al., 2001). The operculum comprises much of the nonfiltering portion of the trabecular meshwork in the anterior portion of the ICA (Rohen & Lutjen‐Drecoll, 1989). It consists of the peripheral extension of the corneal endothelium (and its subjacent basement membrane, i.e., Descemet’s membrane) and the underlying, anterior most portion of the corneoscleral trabecular meshwork. The operculum is especially well developed in dogs, 34 rabbits, and nonhuman primates (Fig. 2.56; Samuelson, 1996). The peripheral termination of the corneal endothelium and its basement membrane leads to what is referred to as the cribriform ligament because it branches both posteroexternally, into the corneoscleral trabecular meshwork, and poster internally, joining with the anterior uveal trabeculae. The function of the operculum is unknown; however, cells intimately associated with the operculum (i.e., Schwalbe’s line cells) are secretory in nature, able to form and release certain enzymes, including enolase and hyaluronans synthase, and phospholipid surfactant‐like material (Fig. 2.57; Allen et al., 1955; Lutjen‐Decoll & Rohen, 1992; Raviola, 1982; Samuelson et al., 2001; Stone et al., 1984). Although the role these cells play in the mature ICA has yet to be determined, Schwalbe’s line cells represent a distinct subpopulation within most mammalian ICAs. The external boundary of the corneoscleral trabecular meshwork is formed by the sclera and a plexus of aqueous humor collector vessels. In mammals and most lower vertebrates, the aqueous humor chiefly exits the eye through the trabecular meshworks into these vessels (see Fig. 2.55). In most mammals, these vessels consist of a small network of veins collectively termed the AAP (Tripathi, 1971a, 1974). These vessels have radially oriented lumens, differing from the circumferentially coursing canal of Schlemm in primates (Grierson et al., 1977; Kayes, 1967; Tripathi, 1971b). The plexiform nature of the drainage vessels in most mammals allows removal of a substantial amount of aqueous humor. The single canal in primates most likely represents an evolutionary adaptation for removal of aqueous humor from a relatively small anterior chamber through a small, compact ICA bordered by a large and highly developedvCBM essential for accommodation. Adjacent to thevmeshwork are aqueous collecting channels, which in turn empty into the intrascleral venous plexus and then the vortex veins. The size of the individual collector vessels (i.e., trabecular veins) and the tissue immediately adjacent to the AAP varies considerably among mammals. The trabecular veins in cattle, sheep, and water buffalo are large and extensive. Those associated with dogs, cats, pigs, and horses are less prominent but are still extensive. The manner by which aqueous humor flows into the trabecular veins of the AAP or canal of Schlemm is not completely understood. Most of the aqueous humor is thought to move through large, vacuole‐like structures of the inner endothelial cells (see Fig. 2.55). The area adjacent to the trabecular veins typically consists of a zone of cellular elements intermixed with irregularly arranged elastin, collagen, and basement membrane‐like material. These elements are much more compact in the horse than in other mammals, except for humans. They constitute a separate zone, called the juxtacanalicular zone that is readily distinguished from the rest of the outer corneoscleral 35 trabecular meshwork. The function of this zone has yet to be determined, but it may contribute substantially to aqueous humor outflow resistance. In some species, including dogs, rats, rabbits, and humans, smooth muscle‐like cells (myofibroblastic cells) have been observed in the trabecular meshwork, especially adjacent to the aqueous humor outflow channels and along the distal or outer walls of the AAP and Schlemm’s canal (de Kater et al., 1990; Ryland et al., 2003). In the dog, the presence of myofibroblastic cells within the ICA suggests that these cells and the smooth muscle cells of the ciliary body along the same plane of orientation function to facilitate the removal of aqueous humor and are likely to be influenced by vascular mediators. Whether all myofibroblastic cells play a significant role in regulation of aqueous humor outflow is unknown. GAGs form an integral component of trabeculae within the ICA and the area adjacent to the AAP (and canal of Schlemm). Treatment with hyaluronidase results in lowering of the IOP. GAGs appear to regulate IOP via their state of polymerization, which controls hydration capacity and swelling or shrinking. 36 Uveoscleral Outflow Aqueous humor is not entirely removed by a plexus of collector vessels via the ICA. Some aqueous humor drains either posteriorly into the vitreous humor, anteriorly within the iridal stroma and across the cornea, or exteroposteriorly along a supraciliary–suprachoroidal space into the adjacent sclera (Fig. 2.58; Bill, 1985; Bill & Phillips, 1971; McMaster & Macri, 1968; Smith et al., 1988). The lattermost pathway is called the uveoscleral, or unconventional, outflow pathway. The degree of uveoscleral outflow varies remarkably between species, with cats experiencing the least drainage (3%), followed by humans (4%– 14%), rabbits (13%), dogs (15%), and nonhuman primates (30%–65%) (Barrie et al., 1985; Bill & Phillips, 1971). In the horse, the uveoscleral pathway may be just as important as the conventional route for aqueous humor removal. Large spaces of the outer uveal meshwork become confluent posteriorly, with a uniquely wide and well‐defined meshwork between the CBM and the sclera (i.e., the supraciliary space). This region, which has been found only in the horse, is called the supraciliary meshwork, and most likely represents a major pathway for aqueous humor removal (Fig. 2.59; Samuelson et al., 1989). In pigs, cattle, dogs, cats, and horses, the outer anterior CBM forms longitudinal and circumferential attachments to trabeculae of the ICA (Samuelson & Birkin‐Streit, 2011; Sedacca et al., 2011). Spaces between the endings of the CBM form avenues for the beginning of the uveoscleral pathway (Fig. 2.60). In pigs, the corneoscleral trabecular meshwork is anchored by a scleral spur, which in turn anchors much of the outer CBM. As a result, the uveoscleral pathway is limited to small intertrabecular spaces of the posterior ICA that interface with the anterior CBM. Within the outer ciliary body, there are blood vessels in close proximity to the sclera. More posteriorly, the supraciliary space contains occasional collagenous trabeculae (Sedacca et al., 2011). In cattle and domestic carnivores, outer anterior muscle bundles attach the CBM to the sclera with an anterior elastic sheath, which is especially well developed in dogs. Initially, the uveoscleral pathway consists of small intercellular spaces lying between the attachments of the anterior smooth muscle bundles of the ciliary body and the posterior uveal trabecular meshwork. Within the supraciliary space, slender trabeculae are most numerous anteriorly extending from the ciliary body obliquely and radially. In horses, the outer anterior muscle bundles of the ciliary body are connected to branching connective tissue trabeculae within the uveoscleral pathway that are attached radially to the sclera (Samuelson et al., 1989; Sedacca et al., 2011). An elastic sheath lines this portion of the uveoscleral pathway. Trabeculae within the supraciliary space are extensive, consisting of either collagen or muscle. Both 37 types of trabeculae are often branched and lined by melanocytes, becoming less numerous, narrower, and less branched posteriorly. Aging A number of age‐related changes occur in the ciliary body. As the nonpigmented epithelium grows older, its basement membrane thickens greatly, becoming multilaminar (Streeten, 1988). The pigmented epithelial basement membrane similarly thickens with age, producing a nearly identical multilaminar appearance. The nonpigmented epithelium thins irregularly. The stroma of the ciliary body concomitantly thickens because of increased amounts of collagen and other extracellular materials. The degree of pigmentation in the ciliary body lessens with age, particularly in the pigmented epithelium along the crests of the ciliary processes (Streeten, 1988). Age‐related changes associated with the ICA are well documented in humans and dogs (Bedford & Grierson, 1986; Hogan et al., 1971; Samuelson & Gelatt, 1984a, 1984b, 1998). The cortical zone within the trabecula broadens in older individuals. This thickening is caused by the presence of additional basement membrane‐like material and additional amorphous material. The thickened cortical zone could contribute to increased thickening of the corneoscleral trabeculae within the canine ICA. Use of PAS stain, which reacts strongly with basement membrane material, on normal laboratory‐quality Beagle ICAs of different ages (from 3 months to 8 years) demonstrates positive reactions within the corneoscleral trabecular meshwork, especially along the posterior extension of Descemet’s membrane. The apparent increase in PAS staining with age occurs mostly within the anteriormost portion of the corneoscleral trabecular meshwork. No age‐related changes have been observed or reported within the uveal trabeculae of the canine ICA (Bedford & Grierson, 1986). Perhaps the most discernable alterations of the canine ICA with regard to age are those associated with the extracellular fibers comprising the bulk of each trabecula. When comparing the average diameter of the collagen fibril as well as the distribution of diameter size within the inner and outer regions of the corneoscleral trabecular meshwork and subjacent sclera next to the aqueous plexus at different ages, several changes are recognized (Gelatt & Samuelson, 1986). The average diameter of collagen fibrils within the outer corneoscleral trabecular meshwork increases by approximately 25% during the second and third years of life compared with the latter half of the first year (Samuelson & Gelatt, 1998). On the other hand, collagen fibrils within the inner corneoscleral trabecular meshwork 38 and adjacent outer uveal trabecular meshwork become progressively thinner during the first 3 years of life. Early age‐related changes in collagen fibrillar size are most remarkable within the sclera forming the outermost lining of the ICA, increasing nearly 100% in average diameter over a 2.5‐year period. The increase of collagen fibrillar size within the inner scleral wall of the limbal region occurs unevenly, with some fibrils remaining small and others attaining considerable widths. The appearance of the trabecular (i.e., endothelial) cells in both the uveal and corneoscleral meshworks changes mostly during the first year of life. As the cells become more separated spatially, they elongate and possess less clustered cytoplasmic processes. In older animals (>4 years of age), fewer cells are observed in both meshworks compared with those in younger animals (<1 year of age). By the end of the first year, the average cellular density of both meshworks has dropped by nearly 30% (Samuelson & Gelatt, 1998). The lowering of cellular density during this period undoubtedly results from the overall increase in ICA size during the first year of life in dogs (Samuelson & Gelatt, 1984a, 1984b). A further substantial decrease in cellular density is not observed for another 5 years, until the seventh and eighth years of age. The size of the ICAs in older dogs (7–8 years of age) is, in fact, slightly smaller than that in the younger mature dogs (1–6 years of age), which may be attributed to the age‐related increase in lens size. These changes appear to reduce the area available for aqueous humor outflow. Innervation As mentioned previously, the ciliary musculature is innervated both sympathetically and parasympathetically (Gum et al., 2007; Murphy et al., 2012). Cholinergic and adrenergic nerve endings have been observed in the various components of the ciliary body, including the trabecular meshwork and within the ICA. In the dog, cholinergic activity is most intense in the musculature, ciliary processes, and epithelium. Dense adrenergic innervation, however, is located in the subepithelial portions of the ciliary body (Gwin et al., 1979). Species differences in the distribution of these nerves among many lower mammals have been demonstrated (Ehinger, 1966a), but the function of the cholinergic and adrenergic nerves within the ciliary body remains speculative (Ehinger, 1966b; Nomura & Smelser, 1974). 39