Nervous Systems.docx
Document Details
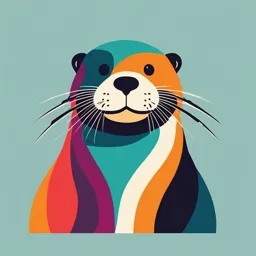
Uploaded by LovingLobster
Full Transcript
• What is the main function of our nervous system? Control and co-ordination –Fast –Generally short-lived responses –Electrically mediated Neurons and glial cells are cellular elements of nervous tissue. Glial cells outnumber neurons between 3:1 to 10:1• Several subtypes of glial cells Role of glial...
• What is the main function of our nervous system? Control and co-ordination –Fast –Generally short-lived responses –Electrically mediated Neurons and glial cells are cellular elements of nervous tissue. Glial cells outnumber neurons between 3:1 to 10:1• Several subtypes of glial cells Role of glial cells is to nurture neurons Types of Glial cells (Neuroglia) • Ependymal cells –e.g. line ventricles, assist in production of CSF • Microglia –remove debris, waste by phagocytosis • Astrocytes –BBB, absorb/recycle neurotransmitters • Oligodendrocytes –myelinate CNS axons Neurons Functional units of the Nervous System • Main function is to Process Information • Three regions – 1. Cell body and dendrites (Inputs) 2. Axon (Transmission) 3. Axon terminals (Outputs) • All these functions are mediated by neuronal membrane functions Neurons are very diverse Neurons are classified based on their structure; Divergence • spread of information to several neurons • e.g. significant divergence of sensory information reaching different parts of CNS Convergence • several neurons synapse on a single neuron • e.g. voluntary breathing vs. breath-holding Serial processing • stepwise processing of information • nociceptive pathways Parallel processing • several neurons receive the same information (divergence must occur) • stepping on pin –foot moves, weight moves, sense of pain Reverberation • positive feedback loop • maintenance of wakefulness • Nervous tissue composed of neurons and glia • Several subtypes of glia –now thought to be more than passive • Neurons function because of their membrane properties • Integrative action of neurons is essential –neuronal pools CNS consists of brain and spinal cord. Protecting your brain- bone, meninges, cerebrospinal fluid Divisions of the brain • Medulla • Pons • Midbrain • Limbic system • Diencephalon • Cerebrum • Cerebellum Brainstem –medulla, pons, midbrain • Evolutionary very old • ‘Basic’ functions –respiratory rhythm, blood pressure regulation, vomiting • Concept of centres • Origin of cranial nerves • Midbrain constitutes part of the reticular formation –maintenance of wakefulness, aspects of visual and auditory processing Cranial Nerves • Organisation of the brainstem showing the cranial nerves • Twelve cranial nerves, I-XII • All innervate head & neck except Vagus nerve Thalamus • final relay point for sensory information that will be transmitted to the cortex –acts as a filter• Hypothalamus • Controls and integrates activity of the ANS • Controls the pituitary gland (release hormones) and releases its own hormones • Regulates emotional and behavioural patterns e.g. eating and drinking • Controls body temperature • Subconscious control of skeletal muscle in relation to behavioural patterns e.g. rage, aggression, pain • Coordination between voluntary and autonomic functions e.g. increased HR when thinking about dangerous situations • Contains the suprachiasmatic nucleus which controls circadian rhythms. Cerebellum • Lies below the cerebrum and behind the brainstem • Divided into 2 lobes, thence into 9 lobules • Consists of outer grey matter and internal white matter • Connected to the brainstem by three pairs of nerve fibres –cerebellar peduncles • Adjusts postural muscles • By modifying motor centres in the brainstem • Programming/Fine tuning conscious and subconscious movements • compares proprioceptive information with motor commands Inferior cerebellar peduncle Cerebellum –medulla: receive inputs from proprioceptors Middle cerebellar peduncle Cerebellum –pons: voluntary motor activity initiated in motor cortex Superior cerebellar peduncle Cerebellum –midbrain: fibres from cerebellum to motor cortex via thalamus What does the cerebellum do? • Electrical stimulation = no loss of sensation or movement • However, loss of cerebellum = significant motor abnormalities • Overall, role in controlling postural muscles and rapid muscular activity e.g., typing, playing a musical instrument • Inputs –motor cortex, vestibular system, proprioceptors • Outputs –via thalamus to motor cortex and premotor cortex and to vestibular nuclei and reticular formation Supplement motor activity – particularly control of posture and correcting rapid movements initiated in motor cortex (comparator) • Motor learning • Much of what we know comes from damage such as a staggering walk and difficulty standing –cerebellar ataxia and loss of coordination (asynergia) and muscle tone (hypotonia) Cerebrum • Separated into two cerebral hemispheres by the longitudinal fissure, each hemisphere divided into four lobes • Hemispheres connected by the corpus callosum • Outermost layer of each hemisphere is the cortex, below which is the white matter. • The cortex enlarges at a greater rate than the underlying white matter – hence its convoluted appearance. Peaks are called Gyri, troughs are called Sulci • Cortex divided into several functional areas – –motor –sensory –association –integrative Sensory Cortex • Mainly the posterior half of both cerebral hemispheres, association areas adjacent to these regions • Primary Somatosensory Area (Area 1,2,3) -located directly posterior to the central sulcus • Primary visual area (Area 17) -posterior tip of occipital lobe • Primary Auditory Area (Area 41, 42) –superior part of temporal lobe Association Areas • Interpret incoming information (or coordinate motor responses) • Somatosensory Association Area (5,7) integrates and interprets sensations e.g., determining shape and texture • Visual Association Area (18,19) • Premotor Area (6) Integrative Centres • Coordinate information from several association areas (and direct complex movements) • Also undertake complicated analytical functions e.g. abstract thought –General interpretive area –Wernicke’s area –integrates sensory info and oversees access to visual and auditory memories –damage limits the ability to interpret what is seen or heard Motor Activity • Electrical stimulation of areas of the cortex resulted in movements of specific contralateral areas • How we generate voluntary movement not clearly understood –But possible to record electrical activity in the pre-motor cortex, almost a second before movement=readiness potential Spinal Cord Gross Anatomy • Cylindrical in shape -~45cm long, 2cm diameter • Spinal cord is shorter than the vertebral column • Covered by meninges and enclosed by the vertebral column • Filum terminale anchors the spinal cord to the coccyx Spinal Nerves • Arising from the spinal cord are 31 pairs of spinal nerves (these branch extensively and constitute the peripheral nervous system) • Points of attachment to the cord are called Roots • Posterior (dorsal) root - afferent (sensory) • Anterior (ventral) root- efferent (motor) Functions of the Spinal Cord • A link between the brain and the rest of the body -a function of the white matter • Participation in neural responses -SPINAL REFLEXES -a function of the grey matter Sensory and Motor Tracts • White matter divided into tracts • Links between the brain and the periphery • Ascending (Sensory) tracts convey impulses to the brain • Descending (Motor) tracts convey impulses from the brain Spinothalamic tract –cross in spinal cord. Crude touch, pain, temperature sensation to brain. Dorsal Column Pathway – cross in medulla. Information about joint sense and fine discriminatory touch Spinal Reflexes • Gray matter serves as an integrating centre for spinal reflexes • Reflexes are fast, predictable automatic responses to stimuli in the environment • Somatic reflexes, which result in muscle movement are the most obvious type Components of a reflex • Receptor • Afferent Neuron • Integrating Centre • Efferent Neuron • Effector The Stretch Reflex • A simple monosynaptic reflex • Muscle stretch is detected by muscle spindles • Afferent neurons synapse directly with motor neurons. • Motor neurons are activated resulting in muscle contract which returns it back to its original length • Simple spinal reflexes (a)Stretch reflex e.g. knee jerk. This is a monosynaptic reflex (b) Withdrawal reflex –polysynaptic reflex Crossed Extensor Reflex • Polysynaptic, contralateral, intersegmental reflex • Stepping on a pin • Flexor muscles of the thigh contract and withdraw the leg from the painful stimulus • At the same time, the extensor muscles on the other leg contract allowing all the weight of the body to be supported on one leg • The autonomic nervous system (ANS) is responsible for the regulation of visceral functions and maintenance of homeostasis of the internal environment. It is not under conscious control. Divisions of the Autonomic Nervous System • Sympathetic Division (SANS) • Parasympathetic Division (PANS) • Enteric Division General Organisation of the ANS •Consists of a two-neuron chain • First neuron -cell body in the central nervous system (Preganglionic Neuron) • Synapse in ganglia where second order neurons originate from, and which innervate effectors (Postganglionic Neuron) Organisation of the SANS • Preganglionic neurons lie in the intermediolateral cell column between T1-L2 (i.e. thoracolumbar outflow) • Preganglionic fibres synapse in paired paravertebral ganglia (22 of these on either side of the spinal cord extending from cervical to sacral region) • Preganglionic fibres may pass up/down the paravertebral ganglia before synapsing Exceptions • Some preganglionic neurons pass through the paravertebral ganglia without synapsing - These preganglionic neurons eventually synapse with postganglionic neurons in the prevertebral ganglia -These postganglionic neurons innervate the glands, blood vessels and smooth muscles of the abdominal and pelvic viscera • Some preganglionic fibres directly innervate the adrenal gland -Adrenal gland may be a specialized sympathetic ganglion -The effect of stimulation is to release adrenaline and nor adrenaline into the blood resulting in a diffuse, wide ranging sympathetic response SANS Neurotransmitters • Between pre and postganglionic neurons the neurotransmitter is acetylcholine -the receptor subtype is nicotinic (cholinergic) • Between postganglionic neuron and effector the neurotransmitter is noradrenalin -the receptor subtype is adrenergic Organisation of the PANS • Cell bodies of preganglionic neurons lie in the brainstem and intermediolateral cell column of the sacral spinal cord (Craniosacral outflow) • The ganglia of the PANS are in close proximity to the effector structure. In some case the ganglia (and postganglionic neuron) are part of the effector structure PANS Neurotransmitters • Between pre and postganglionic neurons the neurotransmitter is acetylcholine -the receptor subtype is nicotinic • Between postganglionic and effector, the neurotransmitter is acetylcholine -the receptors Summary of some SANS and PANS effects Patterns of Innervation • Dual antagonistic innervation • Single innervation • Dual nonantagonistic innervation ANS – Higher Brain Centres • Visceral afferents enter spinal cord via dorsal horn • Second order neurons terminate in various brainstem nuclei and thalamus and hypothalamus • Activation of these afferents generates autonomic reflexes e.g., baroreceptors (afferents reach brainstem via vagus) • In response to danger –defence reaction –normal reflex control overridden, increases in HR, BP, CO • ANS controls visceral homeostasis • Various subdivisions • Range of patterns of innervation and transmitters • There is overarching central control of ANS The Action Potential • Communication of information • Membrane phenomenon • Transitory reversal of the membrane potential (1ms) • Conduction of information along nerve cells –‘frequency coding of information Intracellular recording of an AP in a neuron • Depolarization –Decrease in membrane potential i.e. membrane potential becomes less negative • Repolarization –Increase in membrane potential i.e. membrane potential becomes more negative • Hyperpolarization –Membrane potential returning to RMP following an AP What is the ionic basis of the action potential? • Increased Na+ permeability – Remove Na+ from the ECF – no AP • That the peak of the AP is almost the same vale as the Na+ electrochemical equilibrium potential for Na+ (defined by Nernst) indicates increased Na+ permeability is essential • Na+ moves because voltage gated Na+ channels open What triggers the AP? • Depolarisation to threshold by local currents. This must occur before an AP can be generated – Inputs from other neurons – Sensory activation • This initial depolarization has to bring the membrane at the axon hillock to threshold – generally 15-30mV above RMP The AP peaks • Reversal of membrane potential from –60mv to +40mV • Inactivation of sodium channels – voltage regulated gates begin to close when membrane potential approaches +30mv. Cannot open until membrane repolarised • At this point voltage regulated potassium channels are opening The AP returns to rest • Potassium ions move out of the cell along the electrochemical gradient. – Passive movement. – Repolarisation. • Membrane potential begins to return to resting level. • Brief period of hyperpolarisation • Potassium channels close • Sodium – potassium pump restores intracellular and extracellular ion concentrations to prestimulation levels How soon can a second AP be generated? • Mentioned earlier that neural information is frequency coded – number of APs/sec ‘defines’ information • Is there a limit on the frequency? • How soon can a second AP be generated after the first – concept of the refractory period What is the Refractory Period (RP) • Period of time after an initial AP has been generated where it is impossible or difficult to generate a second AP • Absolute RP -Absolute RP – from time sodium channels open at threshold until sodium channels inactivated – membrane totally inexcitable -Absolute RP lasts 0.4 – 1.0 msec • Relative RP -Relative RP begins when sodium channels regain their normal resting condition and lasts until membrane potential stabilises at resting levels. -Membrane can be excited if stronger than threshold stimulus is applied – membrane is repolarizing/hyperpolarized – inward flow of +ve charge has to win AP Propagation • The nerve impulse is propagated from axon hillock body to axon terminals (Orthodromic propagation) • Does this by local currents – each region of membrane depolarizing the region in front of it • In myelinated axons this only happens at nodes of Ranvier (salutatory conduction) • Retrograde (antidromic propagation) prevented by the RP • AP due to Na+ influx followed by K+ efflux – both due to voltage gated channels • AP frequency limited by RP • Local currents permit orthodromic propagation of AP Resting Membrane Potential • Why the inside of a neuron is negatively charged with respect to the outside • Resting = the cell is doing nothing – the membrane potential is stable • Membrane potentials are measured as potential difference (voltage) • Many cells exhibit a potential difference across their cell membrane – ΔVm • In neurons the ΔVm is termed the RMP • Typical values -70 mV to -90 mV Neurons • Neurons like any other cell are bounded by a plasma membrane • Composition of the ECF and ICF are quite different • The sodium pump Na+/K+ ATPase is electrogenic What happens next? • K+ efflux leads to the development of a voltage across the membrane • With each ion leaving it becomes increasingly difficult for the next to leave (the voltage is establishing an inward electrical gradient) • At some point then, for every K+ leaving down the concentration gradient, one K+ is forced back in down the electrical gradient • The two gradients are equal and opposite –electrochemical equilibrium • The voltage at which this occurs is called the electrochemical equilibrium potential • If we know the concentration of K+ in the ICF and ECF we can predict this voltage –Nernst Equation Nernst Equation • Assumes that only one ion is involved –it is we have chosen to ignore all the others (for the time being) • Assumes that the membrane separating the two K+ solutions is permeable to K+ - there are K+ channels • [K+]o= 4mM, [K+]i = 150mM • EK ~97mV • That’s nearly what we measure –K+ efflux is the major determinant of RMP • Use the Nernst equation for all the other ions described in the earlier table –none come close to RMP! • There are more than just K+ ions but remember the Nernst equation assumes the membrane is fully permeable to an ion • Lets assume that Na+ permeability is about 1/100th that of K+ permeability • Some Na+ ions would enter the neuron –lots of it on the outside, and neurons are negatively charged on the inside • That would bring +ve charge into the cell • The value of -90mV due to K+ alone would become less negative –and move towards the value we measure • RMP is primarily due to K+ efflux plus a small Na+ influx Goldman Constant Field Equation • This is a derivation of the Nernst equation – it considers the concentrations of all ions and their permeability (relative to K+ permeability) • Neurons are ‘special’ • Membrane properties account for what we know of them • RMP is primarily K+ efflux with small Na+ influx Electrical Synapses • Gap junction • Cells are said to be “electrically coupled” • Pre- and post-synaptic membranes held together at gap junctions • 2-4nm gap between cells • Channels between cells – Connexon- formed by six connexins – Cells are said to be “electrically coupled” • Proteins contain pores which allow ion movement between cells -effectively two neurons ‘share a membrane’-a high conductance pathway • Present in both PNS and CNS but rare – vestibular nuclei, the eye (ciliary ganglia) Chemical Synapses • Synaptic gap between cells • Neurotransmitters • Pre and post-synaptic cells not directly coupled • Most widely studied is the NMJ, but also now chromaffin cells of adrenal medulla, synaptosomes and brain slices • Big differences between CNS and PNS: –NMJ –arrival of a single AP results in fast (200μs) release of NT –CNS much slower, 10-100msec, several APs may be required for the release of a single vesicle Varieties of CNS Synapses: • Axodendritic: Axon to dendrite – most common kind • Axosomatic: Axon to cell body • Axoaxonic: Axon to axon • Dendrodendritic: Dendrite to dendrite – most rare kind Synaptic vesicles release Neurotransmitters, process is dependent on Calcium Ions Ca2+ • Evidence for this: –Removal of extracellular Ca2+ –Ca chelators e.g. EGTA –Non-specific blockade of Ca channels –Fluorescence imaging e.g. injection of aequorin into synapses Synaptic Transmission – sequence of events • AP = Increase in Ca2+ permeability • Ca2+ activates Ca2+ / Calmodulin dependent kinases, phosphorylating synapsin proteins, triggering vesicle fusion and exocytosis • Transmitter binding to post synaptic neuron • Initiates change in postsynaptic cell (hyperpolarisation or a depolarisation) • Degradation of transmitter Proteins involved in Neurotransmission • Bewildering array of proteins are thought to be involved: • Vesicular Proteins: -Synapsin -Synaptophysin • Plasma membrane SNARE proteins: -Syntaxin -SNAP-25 • Synapsin attaches vesicles to the cytoskeleton. Phosphorylation results in release. • Syntaxin, SNAP25 involved in priming the vesicle prior to fusion and release of NT (targets for the protease toxins of Clostridium) • (?) Synaptophysin and physophilin form a fusion pore. Neurotransmitter Degradation • Diffusion: Away from the synapse • Reuptake: Neurotransmitter re-enters presynaptic axon terminal (e.g. serotonin (5-HT)) • Enzymatic destruction: -inside terminal cytosol -or synaptic cleft (e.g. acetylcholinesterase) Neurons may release more than one neurotransmitter • Dales ‘Law’ stated that a single synapse released only a single neurotransmitter–not true! • Now known a single synapse may release >1 substance -Some of these substances may alter pre and post-synaptic responses to neurotransmitters and are called NEUROMODULATORS e.g. neuropeptides e.g. endogenous opioids inhibit the release of SubP -Neuromodulators are slow to act, have long term responses, may involve intermediary compounds, may have pre and post synaptic actions -Possible that some neurons may also release multiple primary neurotransmitter • Vasoactive Intestinal Polypeptide (VIP) binding to Ach receptors enhances saliva secretion Excitatory Transmission • Excitatory neurotransmitters act on the postsynaptic membrane to produce depolarisation -favouring AP firing • The postsynaptic electrical response is a brief depolarisation followed by a slower decline to the resting potential -an excitatory postsynaptic potential (EPSP) • The EPSP differs from the AP in that it is –a) not conducted over the cell –b) it is a graded response not ‘all or nothing’ • The larger the number of excitatory synapses stimulated, the larger the EPSP –This is called spatial summation -the effects of the excitatory transmitter release at different synapses adds together to give a larger total response • Similarly, repeated stimulation of a single excitatory synapse may increase the peak of the EPSP • This is temporal summation • If summation leads to a large enough depolarisation (i.e. to threshold) the postsynaptic neuron will generate an AP which then travels along its axon Inhibitory Transmission – Postsynaptic Inhibition • The release of an inhibitory transmitter at an inhibitory synapse result in a hyperpolarisation of the postsynaptic membrane -an inhibitory postsynaptic potential (IPSP) • This moves the membrane further from threshold and makes it less likely that an AP will be generated in response to other excitatory stimuli Synaptic Integration • Process by which multiple synaptic potentials combine within one postsynaptic neuron • Integration: EPSPs added together to produce significant postsynaptic depolarization • Spatial: EPSP generated simultaneously in different spaces • Temporal: EPSP generated at same synapse in rapid succession • Spatial and temporal summation of IPSP’s is also possible • IPSP’s and EPSP’s from different synapses acting on a given neuron summate algebraically • Signals from many different inputs to a cell are integrated in this way allowing for neurons to perform sophisticated computations • An AP will only be generated if the postsynaptic membrane in the region of the axon (axon hillock) reaches threshold • The AP frequency reflects the balance between excitatory and inhibitory influences at any time Ionic Mechanisms of EPSP’s and IPSP’s • Neurotransmitters produce changes in postsynaptic potential by opening receptor operated ion channels • EPSP’s -the excitatory transmitter opens Na+ channels (leads to depolarisation) • IPSP’s -the inhibitory transmitter opens K+ or Cl- (leads to hyperpolarisation) • Chemical neurotransmission very common • Calcium mediated exocytosis • Vast array of transmitters and modulators • Co-transmission more common than we thought • Integration of PSPs decides whether an AP is generated or not