Chapter 4: A Tour of the Cell PDF
Document Details
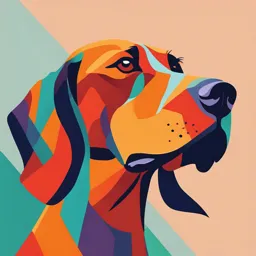
Uploaded by QuietSolarSystem1595
BITS Pilani Hyderabad Campus
Tags
Summary
This document describes the structure and function of cells, the differences and similarities between prokaryotic and eukaryotic cells, and the role of cells in biological processes. The relationship between antibiotics and cellular structures as well as the impact of these relationships is discussed. The chapter explores membrane structure and functions.
Full Transcript
# A Tour of the Cell ## Chapter Contents - The Microscopic World of Cells - Membrane Structure - The Nucleus and Ribosomes: Genetic Control of the Cell - The Endomembrane System: Manufacturing and Distributing Cellular Products - Energy Transformations: Chloroplasts and Mitochondria - The Cytoskel...
# A Tour of the Cell ## Chapter Contents - The Microscopic World of Cells - Membrane Structure - The Nucleus and Ribosomes: Genetic Control of the Cell - The Endomembrane System: Manufacturing and Distributing Cellular Products - Energy Transformations: Chloroplasts and Mitochondria - The Cytoskeleton: Cell Shape and Movement ## Humans Versus Bacteria ### Biology and Society #### Antibiotics: Drugs That Target Bacterial Cells Antibiotics-drugs that disable or kill infectious bacteria-are a marvel of modern medicine. The first antibiotic to be discovered was penicillin in 1920. A revolution in human health rapidly followed. Fatality rates of many diseases (such as bacterial pneumonia and surgical infections) plummeted, saving millions of lives. In fact, human health care improved so quickly and so profoundly that some doctors in the early 1900s predicted the end of infectious diseases altogether. Alas, this did not come to pass (see the Evolution Connection section in Chapter 13 for a discussion of why infectious diseases were not so easily defeated). The goal of antibiotic treatment is to knock out invading bacteria while doing no damage to the human host. So, how does an antibiotic zero in on its target among trillions of human cells? Most antibiotics are so precise because they bind to structures found only in bacterial cells. For example, the common antibiotics erythromycin and streptomycin bind to the bacterial ribosome, a vital cellular structure responsible for the production of proteins. The ribosomes of humans are different enough from those of bacteria that the antibiotics bind only to bacterial ribosomes, leaving human ribosomes unaffected. Ciprofloxacin (commonly referred to as Cipro) is the antibiotic of choice to combat anthrax-causing bacteria. This drug targets an enzyme that bacteria need to maintain their chromosome structure. Your cells can survive just fine in the presence of Cipro because human chromosomes have a sufficiently different makeup than bacterial chromosomes. Other drugs, such as penicillin, ampicillin, and bacitracin, disrupt the synthesis of cell walls, a structure found in most bacteria that is absent from the cells of humans and other animals. This discussion of how various antibiotics target bacteria underscores the main point of this chapter: To understand how life works-whether in bacteria or in your own body-you first need to learn about cells. On the scale of biological organization, cells occupy a special place: They are the simplest objects that can be alive. Nothing smaller than a cell is capable of displaying all of life's properties. In this chapter, we'll explore the microscopic structure and function of cells. Along the way, we'll further consider how the ongoing battle between humans and infectious bacteria is affected by the cellular structures present on both sides. ### The Microscopic World of Cells Each cell in your body is a miniature marvel. If the world's most sophisticated jumbo jet was reduced to microscopic size, its intricacy would pale next to a living cell. Organisms are either single-celled, such as most prokaryotes and protists, or multicelled, such as plants, animals, and most fungi. Your own body is a cooperative society of trillions of cells of many specialized types. As you read this page, muscle cells allow you to scan your eye across the words, while sensory cells in your eye gather information and send it to brain cells, which interpret the words. Everything you do-every action and every thought-is possible because of processes that occur at the cellular level. Figure 4.1 shows the size range of cells compared with objects both larger and smaller. Notice that the scale along the left side of the figure increases by powers of 10 to accommodate the range of sizes shown. Starting at the top with 10 meters (m), each subsequent mark represents a tenfold decrease in length. Most cells are between 1 and 100 µm in diameter (yellow region of the figure) and are therefore visible only with a microscope. There are some interesting exceptions: an ostrich egg is a single cell about 6 inches across and weighing about 3 pounds; nerve cells in your body can stretch several feet long; and nerve cells in giant squid can be more than 30 feet long! How do new living cells arise? First formulated in the 1800s, the cell theory states that all living things are composed of cells and that all cells come from earlier cells. So every cell in your body (and in every other living organism on Earth) was formed by division of a previously living cell. (That raises an obvious question: how did the first cell evolve? This fascinating topic will be addressed in Chapter 15.) With that introduction, let's begin to explore the variety of cells found among life on Earth. ## Table 4.1 Comparing Prokaryotic and Eukaryotic Cells | Property | Prokaryotic Cells | Eukaryotic Cells | |---|---|---| | Cytoplasm | Occupies entire interior of cell | Occupies the region between the nucleus and the plasma membrane | | Chromosomes | Single circular chromosome in nucleoid region | One or more linear chromosomes in nucleus | | First Evolved | Approximately 3.5 billion years ago | Approximately 2.1 billion years ago | | Size | Smaller, simpler | Larger, more complex | | Organelles | No membrane-bound organelles | Membrane-bound organelles (for example, nucleus. ER) | | Cell Walls | Most are surrounded by cell walls, some have capsules, pili, and/or flagella | Plant cells surrounded by cell walls; animal cells surrounded by extracellular matric | ## The Two Major Categories of Cells The countless cells that exist on Earth can be placed into two basic categories: prokaryotic cells and eukaryotic cells (Table 4.1). Prokaryotic cells are found in organisms of the domains Bacteria and Archaea, known as prokaryotes (see Figure 1.7). Organisms of the domain Eukarya-including protists, plants, fungi, and animals are composed of eukaryotic cells and are called eukaryotes. All cells, whether prokaryotic or eukaryotic, have several features in common. They are all bounded by a barrier called a plasma membrane, which regulates the traffic of molecules between the cell and its surroundings. Inside all cells is a thick, jellylike fluid called the cytosol, in which cellular components are suspended. All cells have one or more chromosomes carrying genes made of DNA. And all cells have ribosomes that build proteins according to instructions from the genes. Because of structural differences between bacteria and eukaryotes, as mentioned in the Biology and Society section at the beginning of the chapter, some antibiotics-such as streptomycin-target prokaryotic ribosomes, crippling protein synthesis in the bacterial invaders but not in the eukaryotic host (you). Although they have many similarities, prokaryotic and eukaryotic cells differ in several important ways. Fossil evidence shows that prokary-otes were the first life on Earth, appearing more than 3.5 billion years ago. In contrast, the first eukaryotes did not appear until around 2.1 billion years ago. Prokary-otic cells are usually much smaller about one-tenth the length of a typical eukaryotic cell—and are simpler in structure. Think of a prokaryotic cell as being like a bicycle, whereas a eukaryotic cell is like a sports utility vehicle. Both a bike and an SUV get you from place to place, but one is much smaller and contains many fewer parts than the other. Similarly, prokaryotic cells and eukaryotic cells perform similar functions, but prokaryotes cells are much smaller and less complex. The most significant structural difference between the two types of cells is that eukaryotic cells have organelles (“little organs”), membrane-enclosed structures that perform specific functions, and prokary-otic cells do not. The most important organelle is the nucleus, which houses most of a eukaryotic cell's DNA. The nucleus is surrounded by a double membrane. A prokaryotic cell lacks a nucleus; its DNA is coiled into a "nucleus-like" region called the nucleoid, which is not partitioned from the rest of the cell by membranes. Consider this analogy: A eukaryotic cell is like an office building that is separated into cubicles. Within each cubicle, a specific function is performed, thus di-viding the labor among many internal compartments. One cubicle may hold the accounting department, for example, while another is home to the sales force. The "cubicle walls" within eukaryotic cells are made from membranes that help maintain a unique chemical envi-ronment inside each cubicle. In contrast, the interior of a prokaryotic cell is like an open warehouse. The spaces for specific tasks within a “prokaryotic warehouse" are distinct but they are not separated by physical barriers. ## Membrane Structure Before we enter the cell to explore the organelles, let's make a quick stop at the surface of this microscopic world: the plasma membrane. To help you understand the structure and function of the cell membrane, imagine you want to create a new homestead in the wilderness. You will probably want to start by fencing your property to protect it from the outside world. Similarly, the plasma membrane is the boundary that separates the living cell from its nonliving surroundings. The plasma membrane is a remarkable film, so thin that you would have to stack 8,000 of these membranes to equal the thickness of one piece of paper. Yet the plasma membrane can regulate the traffic of chemicals into and out of the cell. As with all things biological, the structure of the plasma membrane correlates with its function. ### The Plasma Membrane The plasma membrane and other membranes of the cell are composed mostly of phospholipids. The structure of phospholipid molecules is well suited to their function as a major constituent of biological membranes. Each phospholipid is composed of two distinct regions-a "head" with a negatively charged phosphate group and two nonpolar fatty acid "tails." Phospholipids group together to form a two-layer sheet called a phospholipid bilayer. As you can see in Figure 4.4a, the phospholipids' hydrophilic ("water-loving") heads are arranged to face outward, exposed to the aqueous solutions on both sides of a membrane. Their hydrophobic ("water-fearing") tails are arranged inward, mingling with each other and shielded from water. Suspended in the phospholipid bilayer of most membranes are proteins that help regulate traffic across the membrane; these proteins also perform other functions (Figure 4.4b). (You'll learn more about membrane proteins in Chapter 5.) Membranes are not static sheets of molecules locked rigidly in place, however. In fact, the texture of a cellular membrane is similar to salad oil. The phospholipids and most of the proteins can therefore drift about within the membrane. Thus, a membrane is a fluid mosaic-fluid because the molecules can move freely past one another and mosaic because of the diversity of proteins that float like icebergs in the phospholipid sea. Next, we'll see how some bacteria can cause illness by piercing the plasma membrane. ### The Nucleus and Ribosomes: Genetic Control of the Cell If you think of the cell as a factory, then the nucleus is its control center. Here, the master plans are stored, orders are given, changes are made in response to external factors, and the process of making new factories is initiated. The factory supervisors are the genes, the inherited DNA molecules that direct almost all the business of the cell. Each gene is a stretch of DNA that stores the information necessary to produce a particular protein. Proteins can be likened to workers on the factory floor because they do most of the actual work of the cell. ### The Nucleus The nucleus is separated from the cytoplasm by a double membrane called the nuclear envelope (Figure 4.6). Each membrane of the nuclear envelope is similar in structure to the plasma membrane: a phospholipid bilayer with associated proteins. Pores in the envelope allow certain materials to pass between the nucleus and the surrounding cytoplasm. (As you'll soon see, among the most important materials that passes between the nucleus and cytoplasm through the nuclear pores are molecules of RNA that carry instructions for building proteins.) Within the nucleus, long DNA molecules and associated proteins form fibers called chromatin. Each long chromatin fiber constitutes one chromosome (Figure 4.7). The number of chromosomes in a cell depends on the species; for example, each human body cell has 46 chromosomes, whereas rice cells have 24 and dog cells have 78 (see Figure 8.2 for more examples). The nucleolus (shown in Figure 4.6), a prominent structure within the nucleus, is the site where the components of ribosomes are made. We'll examine ribosomes next. ### Ribosomes The small blue dots in the cells in Figure 4.3 and outside the nucleus in Figure 4.6 represent the ribosomes. Ribosomes are responsible for protein synthesis (Figure 4.8). In eukaryotic cells, the components of ribosomes are made in the nucleus and then transported through the pores of the nuclear envelope into the cytoplasm. It is in the cytoplasm that the ribosomes begin their work. Some ribosomes are suspended in the cytosol, making proteins that remain within the fluid of the cell. Other ribosomes are attached to the outside of the nucleus or an organelle called the endoplasmic reticulum (Figure 4.9), making proteins that are incorporated into membranes or secreted by the cell. Free and bound ribosomes are structurally identical, and ribosomes can switch locations, moving between the endoplasmic reticulum and the cytosol. Cells that make a lot of proteins have a large number of ribosomes. For example, each cell in your pancreas that produces digestive enzymes may contain a few million ribosomes. ### How DNA Directs Protein Production Like a company executive, the DNA doesn't actually do any of the work of the cell. Instead, the DNA "executive" issues orders that result in work being done by the protein "workers." Figure 4.10 shows the sequence of events during protein production in a eukaryotic cell (with the DNA and other structures being shown disproportionately large in relation to the nucleus). 1) DNA transfers its coded information to a molecule called messenger RNA (mRNA). Like a middle manager, the mRNA molecule carries the order to "build this type of protein." 2) The mRNA exits the nucleus through pores in the nuclear envelope and travels to the cytoplasm, where it binds to a ribosome. 3) The ribosome moves along the mRNA, translating the genetic message into a protein with a specific amino acid sequence. (You'll learn how the message is translated in Chapter 10.) In this way, information carried by the DNA can direct the work of the entire cell without the DNA ever leaving the protective confines of the nucleus. ## The Endomembrane System: Manufacturing and Distributing Cellular Products Like an office partitioned into cubicles, the cytoplasm of a eukaryotic cell is partitioned by organelle membranes (see Figure 4.3). Some of the membranes are physically connected, and others are linked by vesicles (sacs made of membrane) that transfer membrane segments between organelles. Together, these organelles form the endomembrane system. This system includes the nuclear envelope, the endoplasmic reticulum, the Golgi apparatus, lysosomes, and vacuoles. ### The Endoplasmic Reticulum The endoplasmic reticulum (ER) is one of the main manufacturing facilities within a cell. It produces an enormous variety of molecules. Connected to the nuclear envelope, the ER forms an extensive labyrinth of tubes and sacs running throughout the cytoplasm (Figure 4.11). A membrane separates the internal ER compartment from the cytosol. There are two components that make up the ER: rough ER and smooth ER. These two types of ER are physically connected but differ in structure and function. #### Rough ER The "rough" in rough ER refers to ribosomes that stud the outside of its membrane. One of the functions of rough ER is to make more membrane. Phospholipids made by enzymes of the rough ER are inserted into the ER membrane. In this way, the ER membrane grows, and portions of it can bubble off and be transferred to other parts of the cell. The ribosomes attached to the rough ER produce proteins that will be inserted into the growing ER membrane, transported to other organelles, and eventually exported. Cells that secrete a lot of protein-such as the cells of your salivary glands, which secrete enzymes into your mouth-are especially rich in rough ER. As shown in Figure 4.12, 1) some products manufactured by rough ER are 2) chemically modified and then 3) packaged into transport vesicles, sacs made of membrane that bud off from the rough ER. The transport vesicles may then be 4) dispatched to other locations in the cell. #### Smooth ER The "smooth" in smooth ER refers to the fact that this organelle lacks the ribosomes that populate the surface of rough ER (see Figure 4.11). A diversity of enzymes built into the smooth ER membrane enables this organelle to perform many functions. One is the synthesis of lipids, including steroids (see Figure 3.13). For example, the cells in ovaries or testes that produce the steroid sex hormones are enriched with smooth ER. In liver cells, enzymes of the smooth ER detoxify circulating drugs such as barbiturates, amphetamines, and some antibiotics (which is why antibiotics don't persist in the bloodstream after combating an infection). As liver cells are exposed to a drug, the amounts of smooth ER and its detoxifying enzymes increase. This can strengthen the body's tolerance of the drug, meaning that higher doses will be required in the future to achieve the desired effect. The growth of smooth ER in response to one drug can also increase tolerance of other drugs. For example, barbiturate use, such as frequently taking sleeping pills, may make certain antibiotics less effective by accelerating their breakdown in the liver. ### The Golgi Apparatus Working in close partnership with the ER, the Golgi apparatus, an organelle named for its discoverer (Italian scientist Camillo Golgi), who first described this structure in 1898, receives, refines, stores, and distributes chemical products of the cell (Figure 4.13). You can think of the Golgi apparatus as a detailing facility that receives shipments of newly manufactured cars (proteins), puts on the finishing touches, stores the completed cars, and then ships them out when needed. Products made in the ER reach the Golgi apparatus in transport vesicles. The Golgi apparatus consists of a stack of membrane plates, looking much like a pile of pita bread. 1) One side of a Golgi stack serves as a receiving dock for vesicles from the ER. 2) Proteins within a vesicle are usually modified by enzymes during their transit from the receiving to the shipping side of the Golgi apparatus. For example, molecular identification tags may be added that serve to mark and sort protein molecules into different batches for different destinations. 3) The shipping side of a Golgi stack is a depot from which finished products can be carried in transport vesicles to other organelles or to the plasma membrane. Vesicles that bind with the plasma membrane transfer proteins to it or secrete finished products to the outside of the cell. ### Lysosomes A lysosome is a membrane-enclosed sac of digestive enzymes found in animal cells. Most plant cells don't contain lysosomes. Lysosomes develop from vesicles that bud off from the Golgi apparatus. Enzymes within a lysosome can break down large molecules such as proteins, polysaccharides, fats, and nucleic acids. The lysosome provides a compartment where the cell can digest these molecules safely, without unleashing the digestive enzymes on the cell itself. Lysosomes have several types of digestive functions. Many single-celled protists engulf nutrients into tiny cytoplasmic sacs called food vacuoles. Lysosomes fuse with the food vacuoles, exposing the food to digestive enzymes (Figure 4.14a). Small molecules that result from this digestion, such as amino acids, leave the lysosome and nourish the cell. Lysosomes also help destroy harmful bacteria. For example, your white blood cells ingest bacteria into vacuoles, and lysosomal enzymes that are emptied into these vacuoles rupture the bacterial cell walls. In addition, without harming the cell, a lysosome can engulf and digest parts of another organelle, essentially recycling it by making its molecules available for the construction of new organelles (Figure 4.14b). With the help of lysosomes, a cell can thereby continually renew itself. Lysosomes also have sculpting functions in embryonic development. In an early human embryo, lysosomes release enzymes that digest webbing between fingers of the developing hand. The importance of lysosomes to cell function and human health is made clear by hereditary disorders called lysosomal storage diseases. A person with such a disease is missing one or more of the digestive enzymes normally found within lysosomes. The abnormal lysosomes become engorged with indigestible substances, and this eventually interferes with other cellular functions. Most of these diseases are fatal in early childhood. In Tay-Sachs disease, for example, lysosomes lack a lipid-digesting enzyme. As a result, nerve cells die as they accumulate excess lipids, ravaging the nervous system. Fortunately, lysosomal storage diseases are rare. ### Vacuoles Vacuoles are large sacs made of membrane that bud off from the ER or Golgi apparatus. Vacuoles have a variety of functions. For example, Figure 4.14a shows a food vacuole budding from the plasma membrane. Certain freshwater protists have contractile vacuoles that pump out excess water that flows into the cell (Figure 4.15a). Another type of vacuole is a central vacuole, a versatile compartment that can account for more than half the volume of a mature plant cell (Figure 4.15b). A central vacuole stores organic nutrients, such as proteins stockpiled in the vacuoles of seed cells. It also contributes to plant growth by absorbing water and causing cells to expand. In the cells of flower petals, central vacuoles may contain pigments that attract pollinating insects. Central vacuoles may also contain poisons that protect against plant-eating animals. Some important crop plants produce and store large amounts of toxic chemicals-harmful to animals that might graze on the plant but useful to us-such as tobacco plants (which store nicotine) and coffee and tea plants (which store caffeine). ### The Cytoskeleton: Cell Shape and Movement If someone asked you to describe a house, you would most likely mention the various rooms and their locations. You probably would not think to mention the foundation and beams that support the house. Yet these structures perform an extremely important function. Similarly, cells have an infrastructure called the cytoskeleton, a network of protein fibers extending throughout the cytoplasm. The cytoskeleton serves as both skeleton and "muscles" for the cell, functioning in support and movement. #### Maintaining Cell Shape One function of the cytoskeleton is to give mechanical support to the cell and maintain its shape. This is especially important for animal cells, which lack rigid cell walls. The cytoskeleton contains several types of fibers made from different types of protein. One important type of fiber forms microtubules, hollow tubes of protein (Figure 4.19a). The other kinds of cytoskeletal fibers, called intermediate filaments and microfilaments, are thinner and solid. Just as the bony skeleton of your body helps fix the positions of your organs, the cytoskeleton provides anchorage and reinforcement for many organelles in a cell. For instance, the nucleus is held in place by a "cage" of cytoskeletal filaments. Other organelles use the cytoskeleton for movement. For example, a lysosome might reach a food vacuole by gliding along a microtubule track. Microtubules also guide the movement of chromosomes when cells divide (via the mitotic spindle-see Chapter 8). A cell's cytoskeleton is dynamic: It can be quickly dismantled in one part of the cell by removing protein subunits and re-formed in a new location by reattaching the subunits. Such rearrangement can provide rigidity in a new location, change the shape of the cell, or even cause the whole cell or some of its parts to move. This process contributes to the amoeboid (crawling) movements of the protist Amoeba (Figure 4.19b) and movement of some of our white blood cells. ### Cilia and Flagella In some eukaryotic cells, microtubules are arranged into structures called flagella and cilia, extensions from a cell that aid in movement. Eukaryotic flagella (singular, flagellum) propel cells with an undulating, whiplike motion. They often occur singly, such as in human sperm cells (Figure 4.20a), but may also appear in groups on the outer surface of protists. Cilia (singular, cilium) are generally shorter and more numerous than flagella and move in a coordinated back-and-forth motion, like the rhythmic oars of a crew team. Both cilia and flagella propel various protists through water (Figure 4.20b). Though different in length, number per cell, and beating pattern, cilia and flagella have the same basic architecture. Not all animals have cilia or flagella-many do not-and they are almost never found on plant cells. Some cilia extend from nonmoving cells that are part of a tissue layer. There, they move fluid over the tissue's surface. For example, cilia lining your wind-pipe clean your respiratory system by sweeping mucus with trapped debris out of your lungs (Figure 4.20c). Tobacco smoke can inhibit or destroy these cilia, interfering with the normal cleansing mechanisms and allowing more toxin-laden smoke particles to reach the lungs. Frequent coughing-common in heavy smokers-then becomes the body's attempt to cleanse the respiratory system. Because human sperm rely on flagella for movement, it's easy to understand why problems with flagella can lead to male infertility. Interestingly, some men with a type of hereditary sterility also suffer from respiratory problems. Because of a defect in the structure of their flagella and cilia, the sperm of men afflicted with this disorder cannot swim normally within the female reproductive tract to fertilize an egg (causing sterility), and their cilia do not sweep mucus out of their lungs (causing recurrent respiratory infections). ## Humans Versus Bacteria ### Evolution Connection #### The Evolution of Bacterial Resistance in Humans Individuals with variations that make them better suited for the local environment will survive and reproduce more often (on average) than those who lack such variations. When the advantageous variations have a genetic basis, the offspring of individuals with the variations will more often also have the favorable adaptions, giving them a survival and reproductive advantage. In this way, repeated over many generations, natural selection promotes evolution of the population. Within a human population, the persistent presence of a disease can provide a new basis for measuring those individuals who are best suited for survival in the local environment. For example, a recent evolutionary study examined people living in Bangladesh. This population has been exposed to the disease cholera-caused by an infectious bacterium-for millennia (Figure 4.21). After cholera bacteria enter a victim's digestive tract (usually through contaminated drinking water), the bacteria produce a toxin that binds to intestinal cells. There, the toxin alters proteins in the plasma membrane, causing the cells to excrete fluid. The resulting diarrhea, which spreads the bacteria by shedding it back into the environment, can cause severe dehydration and death if untreated. Because Bangladeshis have lived for so long in an environment that teems with cholera bacteria, one might expect that natural selection would favor those individuals who have some resistance to the bacteria. Indeed, recent studies of people from Bangladesh revealed mutations in several genes that appear to confer an increased resistance to cholera. Researchers discovered one mutation in a gene that encodes for the plasma membrane proteins that are the targets of the cholera bacteria. Although the mechanism is not yet understood, these genes appear to offer a survival advantage by making the proteins somewhat more resistant to attack by the cholera toxin. Because such genes offer a survival advantage within this population, they have slowly spread through the Bangladeshi population over the past 30,000 years. In other words, the Bangladeshi population is evolving increased resistance to cholera. In addition to providing insight into the recent evolutionary past, data from this study reveal potential ways that humans might thwart the cholera bacterium. Perhaps pharmaceutical companies can exploit the proteins produced by the identified mutations to create a new generation of antibiotics. If so, this will represent another way that biologists have applied lessons learned from our understanding of evolution to improve human health. It also reminds us that we humans, like all life on Earth, are shaped by evolution due to changes in our environment, including the presence of infectious microorganisms that live around us. ### Energy Transformations #### Chloroplasts and Mitochondria One of the central themes of biology is the transformation of energy: how it enters living systems, is converted from one form to another, and is eventually given off as heat. To follow the energy through living systems, we must consider the two organelles that act as cellular power stations: chloroplasts and mitochondria. #### Chloroplasts Most of the living world runs on the energy provided by photosynthesis, the conversion of light energy from the sun to the chemical energy of sugar and other organic molecules. Chloroplasts, which are unique to the photosynthetic cells of plants and algae, are the organelles that perform photosynthesis. A chloroplast is partitioned into compartments by two membranes, one inside the other (Figure 4.17). The stroma is a thick fluid found inside the innermost membrane. Suspended in that fluid, the interior of a network of membrane-enclosed disks and tubes forms another compartment. Notice in Figure 4.17 that the disks occur in interconnected stacks called grana (singular, granum) that resemble stacks of poker chips. The grana are a chloroplast's solar power packs, the structures that trap light energy and convert it to chemical energy (as detailed in Chapter 7). #### Mitochondria In contrast to chloroplasts (found only in plant cells), mitochondria are found in almost all eukaryotic cells, including those of plants and animals. Mitochondria (singular, mitochondrion) are the organelles in which cellular respiration takes place; during cellular respiration, energy is harvested from sugars and transformed into another form of chemical energy called ATP (adenosine triphosphate). Cells use molecules of ATP as a direct energy source. An envelope of two membranes encloses the mitochondrion, and the inner membrane encloses a thick fluid called the mitochondrial matrix (Figure 4.18). The inner membrane of the envelope has numerous infoldings called cristae. The cristae create a large surface area in which many of the enzymes and other molecules that function in cellular respiration are embedded, thereby maximizing ATP output. (You'll learn more about how mitochondria convert food energy to ATP energy in Chapter 6.) Besides their ability to provide cellular energy, mitochondria and chloroplasts share another feature: They contain their own DNA that encodes some of their own proteins made by their own ribosomes. Each chloroplast and mitochondrion contains a single circular DNA chromosome that resembles a prokaryotic chromosome. In fact, mitochondria and chloroplasts can grow and pinch in two, reproducing themselves. This is evidence that mitochondria and chloroplasts evolved from ancient free-living prokaryotes that established residence within other, larger host prokaryotes. This phenomenon, where one species lives inside a host species, is a special type of symbiosis (see Chapter 16 for further information). Over time, mitochondria and chloroplasts likely became increasingly interdependent with the host prokaryote, eventually evolving into a single organism with inseparable parts. The DNA found within mitochondria and chloroplasts is therefore likely remnants of this ancient evolutionary event. ## The Cytoskeleton: Cell Shape and Movement If someone asked you to describe a house, you would most likely mention the various rooms and their locations. You probably would not think to mention the foundation and beams that support the house. Yet these structures perform an extremely important function. Similarly, cells have an infrastructure called the cytoskeleton, a network of protein fibers extending throughout the cytoplasm. The cytoskeleton serves as both skeleton and "muscles" for the cell, functioning in support and movement. #### Maintaining Cell Shape One function of the cytoskeleton is to give mechanical support to the cell and maintain its shape. This is especially important for animal cells, which lack rigid cell walls. The cytoskeleton contains several types of fibers made from different types of protein. One important type of fiber forms microtubules, hollow tubes of protein (Figure 4.19a). The other kinds of cytoskeletal fibers, called intermediate filaments and microfilaments, are thinner and solid. Just as the bony skeleton of your body helps fix the positions of your organs, the cytoskeleton provides anchorage and reinforcement for many organelles in a cell. For instance, the nucleus is held in place by a "cage" of cytoskeletal filaments. Other organelles use the cytoskeleton for movement. For example, a lysosome might reach a food vacuole by gliding along a microtubule track. Microtubules also guide the movement of chromosomes when cells divide (via the mitotic spindle-see Chapter 8). A cell's cytoskeleton is dynamic: It can be quickly dismantled in one part of the cell by removing protein subunits and re-formed in a new location by reattaching the subunits. Such rearrangement can provide rigidity in a new location, change the shape of the cell, or even cause the whole cell or some of its parts to move. This process contributes to the amoeboid (crawling) movements of the protist Amoeba (Figure 4.19b) and movement of some of our white blood cells.