Myocardial Strain Imaging: Theory, Practice, and Future PDF
Document Details
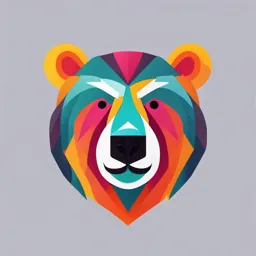
Uploaded by RobustMiracle
Tags
Summary
This article provides a review of myocardial strain imaging, covering its theoretical background, current clinical applications, and future prospects. The paper discusses various imaging techniques like echocardiography and cardiac MRI, emphasizing their importance in diagnosing and managing cardiac diseases. The article also explores the historical context of strain imaging, including its evolution from research tools to widely used clinical measures.
Full Transcript
## Abstract Myocardial strain imaging by echocardiography or cardiac magnetic resonance (CMR) is a powerful method to diagnose cardiac disease. Strain imaging provides measures of myocardial shortening, thickening, and lengthening and can be applied to any cardiac chamber. Left ventricular (LV) glob...
## Abstract Myocardial strain imaging by echocardiography or cardiac magnetic resonance (CMR) is a powerful method to diagnose cardiac disease. Strain imaging provides measures of myocardial shortening, thickening, and lengthening and can be applied to any cardiac chamber. Left ventricular (LV) global longitudinal strain by speckle-tracking echocardiography is the most widely used clinical strain parameter. Several CMR-based modalities are available and are ready to be implemented clinically. Clinical applications of strain include global longitudinal strain as a more sensitive method than ejection fraction for diagnosing mild systolic dysfunction. This applies to patients suspected of having heart failure with normal LV ejection fraction, to early systolic dysfunction in valvular disease, and when monitoring myocardial function during cancer chemotherapy. Segmental LV strain maps provide diagnostic clues in specific cardiomyopathies, when evaluating LV dyssynchrony and ischemic dysfunction. Strain imaging is a promising modality to quantify right ventricular function. Left atrial strain may be used to evaluate LV diastolic function and filling pressure. ## Definition _Strain_ is a term that originates from the field of continuum mechanics and may be used to describe deformations of any structure, including the heart. In cardiac imaging, the term _strain_ is used to describe myocardial shortening and thickening, which are the fundamental features of myocardial fiber function. [Figure 1A](https://www.sciencedirect.com/science/article/pii/S1936878X24003012#fig1) illustrates the most commonly measured myocardial strains. These include left ventricular (LV) longitudinal and circumferential shortening strains and radial thickening strain. Both longitudinal and circumferential strains contribute to LV wall thickening. In addition to these orthogonal normal strains, a complete characterization of LV deformation includes 3 shear strains that are less commonly measured: circumferential-longitudinal shear, or so-called twist, results from 2 short-axis planes rotating relative to each other, as illustrated in [Figure 1B](https://www.sciencedirect.com/science/article/pii/S1936878X24003012#fig1); circumferential-radial shear can be described as the subendocardium is rotating more or less than the epicardium; and radial-longitudinal shear involves the subendocardium is moving more or less longitudinally than the epicardium. Figure 1. Definition of Myocardial Strain Myocardial strain imaging quantifies cardiac deformation. (A) The most widely used left ventricular (LV) strain measures are myocardial shortening in the longitudinal and circumferential directions and thickening in the radial direction (normal strains). (B) Illustration of LV circumferential-longitudinal shear or so-called twist, calculated as the difference between rotations at the apex and base. ECG = electrocardiogram. When calculating myocardial strain, it is most common to use a Lagrangian description that uses the end-diastolic length as a reference (L0 and T0 in [Figure 1](https://www.sciencedirect.com/science/article/pii/S1936878X24003012#fig1)) and expresses the change in length as a percentage of this reference length. A Eulerian description uses the deformed configuration as a reference. Historically, this was the first type of strain that was introduced in echocardiographic software, as it was calculated by integrating strain rate from tissue velocity measurements, but it is less used today. When strain based on the Eulerian (εE) and Lagrangian approach (εL) are expressed as fractions, they are related as εE = ln(1 + εL), where ln is the natural logarithm. Strain imaging provides complementary information to LV ejection fraction (LVEF), as it allows the quantification of segmental as well as global function and can be used to assess both systolic and diastolic function. Whereas myocardial strain imaging has traditionally been applied to study the LV, it is currently implemented also as a tool for the quantification of right ventricular (RV) and left atrial (LA) function. In this paper we review the theory behind strain as a measure of myocardial function, current clinical applications of strain imaging, and prospects for the technology. The [Central Illustration](https://www.sciencedirect.com/science/article/pii/S1936878X24003012#fig26) summarizes the current strain technologies and applications. Central Illustration. Strain Imaging: Technologies and Clinical Applications GLS = global longitudinal strain; HCM = hypertrophic cardiomyopathy; HFpEF = heart failure with preserved ejection fraction; LA = left atrium; LV = left ventricle/ventricular; RV = right ventricle. ## Myocardial Strain: History of the Technology The quantification of myocardial deformation has been available for a long time in cardiac physiology using implanted ultrasonic dimension crystals(https://www.sciencedirect.com/science/article/pii/S1936878X24003012#bib1) ([Figure 2](https://www.sciencedirect.com/science/article/pii/S1936878X24003012#fig2)) or radiopaque markers implanted in a grid pattern so their 3-dimensional (3D) movement could be tracked by high-speed biplane cineradiography to derive the deformations.(https://www.sciencedirect.com/science/article/pii/S1936878X24003012#bib2) Myocardial strain imaging as a clinical modality is rooted in cardiac magnetic resonance (CMR). The earliest CMR methods used radiofrequency pulses and multiple saturation planes, which resulted in distinct lines in the myocardium that could be used as a means of tissue tagging, as they follow myocardial deformation throughout the cardiac cycle.(https://www.sciencedirect.com/science/article/pii/S1936878X24003012#bib3) By the late 1980s, this had been refined to produce a 3D grid of tags known as spatial modulation of magnetization,(https://www.sciencedirect.com/science/article/pii/S1936878X24003012#bib4) and it was applied to interrogate myocardial velocities in 1991.(https://www.sciencedirect.com/science/article/pii/S1936878X24003012#bib5) This method enabled characterizations of segmental strains in both healthy and diseased myocardium, with early studies focusing mainly on the infarcted or hypertrophied myocardium.(https://www.sciencedirect.com/science/article/pii/S1936878X24003012#bib4),(https://www.sciencedirect.com/science/article/pii/S1936878X24003012#bib6),(https://www.sciencedirect.com/science/article/pii/S1936878X24003012#bib7) This method, which is primarily a research tool, is considered the clinical gold standard for the validation of new strain methods such as those based on echocardiography. Contemporaneously with the development of spatial modulation of magnetization tagging methods, CMR tissue phase velocity mapping methods for strain imaging also surfaced.(https://www.sciencedirect.com/science/article/pii/S1936878X24003012#bib8) This technique in general has not been adopted widely, because of lower temporal resolution. This was overcome by the development of navigated high–temporal resolution sequences by 2006,(https://www.sciencedirect.com/science/article/pii/S1936878X24003012#bib9) but long acquisition times have limited its use. Figure 2. History of Myocardial Strain Technologies Graphical illustration of the history of strain imaging. (A) Data from an experimental study in which sonomicrometry was used to measure myocardial percentage shortening and thickening. Adapted with permission from Bugge-Asperheim et al.(https://www.sciencedirect.com/science/article/pii/S1936878X24003012#bib1) (B) Illustration of left ventricular (LV) strain measured by cardiac magnetic resonance (CMR) with tissue tagging. Adapted under CC BY-NC-ND 4.0 license from Shehata et al.(https://www.sciencedirect.com/science/article/pii/S1936878X24003012#bib234) (C) Illustration of LV strain measured using tissue Doppler echocardiography. (D) Illustration of LV strain measured using speckle-tracking echocardiography. (E) Illustration of strain by CMR feature tracking. Adapted under CC BY 4.0 license from Backhaus et al.(https://www.sciencedirect.com/science/article/pii/S1936878X24003012#bib235) (F) Displacement encoding with stimulated echoes (DENSE) imaging CMR is an emerging clinical method for strain measurement. Adapted under CC BY-NC-ND 4.0 license from Auger et al.(https://www.sciencedirect.com/science/article/pii/S1936878X24003012#bib236) 4CH = 4-chamber view; AVC = aortic valve closure. Strain rate and strain by echocardiography were first introduced as a 1-dimensional tissue Doppler imaging–based method.(https://www.sciencedirect.com/science/article/pii/S1936878X24003012#bib10),(https://www.sciencedirect.com/science/article/pii/S1936878X24003012#bib11) For routine clinical use, this method was later superseded by 2-dimensional (2D) speckle-tracking echocardiography (STE), which provides multidirectional strains.(https://www.sciencedirect.com/science/article/pii/S1936878X24003012#bib12), (https://www.sciencedirect.com/science/article/pii/S1936878X24003012#bib13), (https://www.sciencedirect.com/science/article/pii/S1936878X24003012#bib14), (https://www.sciencedirect.com/science/article/pii/S1936878X24003012#bib15) Currently, strain by STE is the most commonly used method. The next phase of CMR strain imaging built on this echocardiography approach, which was applied to standard CMR cine images as feature tracking (FT). Despite greater variability and some discrepancy from the earlier “gold standard” tagging methods, as a result of the simplicity of postprocessing of standard cine images FT CMR is now the dominant strain method used by the CMR community. More recently, other methods have emerged, including 3D FT and techniques such as displacement encoding with stimulated echoes and strain-encoded magnetic resonance imaging. The latter has superior spatial and temporal resolution compared with tagging(https://www.sciencedirect.com/science/article/pii/S1936878X24003012#bib16) and affords the opportunity of real-time imaging. STE has also been applied to 3D data sets, theoretically overcoming the problem of through-plane motion of 2D speckle tracking and allows a comprehensive description of the deformation of the entire ventricle. Nevertheless, challenging data acquisition as well as the lower temporal and spatial resolution of 3D STE limit its clinical use. Interestingly, a recent study showed that the analysis of myocardial deformation is also feasible on time-resolved 3D cardiac computed tomographic images.(https://www.sciencedirect.com/science/article/pii/S1936878X24003012#bib17) The method is based on the calculations of global strain based entirely on the 3D displacement of the segmental myocardial volumes over the cardiac cycle using a deformable image registration–based tracking approach. The proposed method was reported to allow automatic and robust tracking of the LV myocardium using clinical computed tomographic image sequences, but data on clinical applications are still lacking. ## Methodology: CMR A wide range of CMR methods are available to assess myocardial strain. These methods offer various approaches to quantify strain, enabling clinicians and researchers to comprehensively analyze cardiac mechanics. Brief descriptions of the main approaches (myocardial tagging and FT) are provided in the following sections, with the main focus on imaging of the LV. More detailed information can be found elsewhere.(https://www.sciencedirect.com/science/article/pii/S1936878X24003012#bib18),(https://www.sciencedirect.com/science/article/pii/S1936878X24003012#bib19) ### Myocardial tagging With this method, perturbing the magnetization with radiofrequency saturation pulses creates a grid of visible markers (“tags”) in the tissue ([Figure 3](https://www.sciencedirect.com/science/article/pii/S1936878X24003012#fig3)). Myocardial strain is measured by tracking these markers during the cardiac cycle ([Video 1](https://www.sciencedirect.com/science/article/pii/S1936878X24003012#mmc1)). Tags are typically applied at end-diastole, with imaging being performed across the whole cardiac cycle. At lower heart rates, the tags can fade during early diastole, which limits diastolic functional assessment. Higher field strength 3-T magnets can result in longer tag duration throughout the cardiac cycle. Figure 3. Strain by Myocardial Tissue Tagging Image showing myocardial tagging: a grid of dark tag lines applied across a short-axis image. ### CMR tagging sequences All CMR tagging sequences consist of 2 main parts, the tagging part and the image acquisition part. It is the differences in how tagging and data acquisition are performed that make each of the techniques unique. The simplest and most commonly used method for tagging is called spatial modulation of magnetization. Its tagging part consists of 2 nonselective radiofrequency pulses (usually 90°), separated by a tagging gradient ([Figure 4](https://www.sciencedirect.com/science/article/pii/S1936878X24003012#fig4)). After the first pulse, the tagging gradient disperses the excited spins, creating a modulation on the basis of incremental phase shifts. The second pulse then stores the modulated spins in the longitudinal plane, and a spoiler gradient is then applied to remove any remaining transverse magnetization. Spatial modulation of magnetization uses conventional data acquisition for imaging. Figure 4. A Representative Tagging Sequence RF = radiofrequency pulse. More advanced CMR tagging techniques use a combination of modulation and demodulation gradients to better store strain information. One example is the displacement encoding with stimulated echoes technique, which uses a stimulated echo acquisition mode sequence for tagging ([Figure 5](https://www.sciencedirect.com/science/article/pii/S1936878X24003012#fig5)). Stimulated echo acquisition mode consists of 3 90° pulses to generate a stimulated echo. Equal-magnitude displacement encoding (modulation) and decoding (demodulation) gradients are added after the first and third pulses of the stimulated echo acquisition mode sequence, respectively. To eliminate any remaining transverse magnetization, crusher gradients are applied during the mixing period in between modulation and demodulation. Similar to spatial modulation of magnetization, the acquisition part uses conventional imaging readout. Displacement encoding with stimulated echoes provides higher spatial resolution and uses pixel-by-pixel displacement, making it possible to draw representative displacement vectors, with vector magnitude and direction representing the displacement value and orientation. [Figure 6](https://www.sciencedirect.com/science/article/pii/S1936878X24003012#fig6) shows an example of displacement encoding with stimulated echoes imaging CMR. Figure 5. A Representative Displacement Encoding With Stimulated Echoes Sequence The time between the first and second radiofrequency pulses (RFs; α1 and α2) is equal to the time between the third RF pulse (α3) and data acquisition (echo time \[TE\]/2). TM = mixing time. Figure 6. CMR Displacement Encoding With Stimulated Echoes Imaging Reproduced with permission under CC BY-NC-ND 4.0 license from Auger et al.(https://www.sciencedirect.com/science/article/pii/S1936878X24003012#bib236) Ant. = anterior; Inf. = inferior; other abbreviation as in [Figure 2](https://www.sciencedirect.com/science/article/pii/S1936878X24003012#fig2). Another example of advanced tagging sequence is strain-encoded imaging, which uses similar tagging part as spatial modulation of magnetization, but in this case, the tagging gradients are applied parallel to and inside the imaging plane to capture through-plane strain ([Figure 7](https://www.sciencedirect.com/science/article/pii/S1936878X24003012#fig7)). During the imaging part of strain-encoded magnetic resonance imaging, further demodulation (tuning) gradients are applied between the section selection and data readout gradients to reduce scan time. Two sets of demodulated images are ultimately acquired. The low-tuning images represents static tissue, and the high-tuning images represent contracting tissue, exhibiting a high modulation frequency caused by tissue contraction. By comparing the image pixel by pixel and comparing the low-tuning and high-tuning images, myocardial strain can be quantified into a strain map. Figure 7. A Representative Strain-Encoding Sequence RF = radiofrequency pulse. Another CMR tagging technique is called tissue phase mapping. It uses velocity-encoded bipolar gradients to encode the tissue velocity in the phase of the signal. This allows the calculation of the velocities of different points within the myocardium. Myocardial velocities are then integrated in 3 directions (and over time) to show myocardial displacement and allow the evaluation of regional strains in the longitudinal, circumferential, and radial directions. As this method involves long imaging times, which can result in motion artifacts, strain derivation is thus potentially less accurate, and 3D velocity data are typically reported rather than the strain ([Figure 8](https://www.sciencedirect.com/science/article/pii/S1936878X24003012#fig8)). Figure 8. CMR Tissue Phase Mapping (A) Magnitude (i) and phase (ii) images from the tissue phase mapping sequence. (B) Velocity (i) and displacement (ii) maps. (C) Velocity maps in the radial, circumferential, and longitudinal directions obtained. V = velocity; other abbreviation as in [Figure 2](https://www.sciencedirect.com/science/article/pii/S1936878X24003012#fig2). ### FT CMR FT is a postprocessing method for quantifying tissue deformation that uses routinely acquired contrast-free CMR cine images ([Figure 9](https://www.sciencedirect.com/science/article/pii/S1936878X24003012#fig9)). It is based on tracking small image features (eg, details of the endocardial contour) from frame to frame. Combining information on the direction and magnitude of the displacement of several features makes it possible to calculate tissue deformation in all directions within the image plane. When considering frame rate in addition, the method provides information on velocity and strain rate. FT CMR requires high-quality image acquisition with adequate temporal and spatial resolution. Figure 9. CMR Feature Tracking: Circumferential Strain The top panels show the feature points that are tracked, and the bottom panels show resultant displacement by colored strain maps (below) across the cardiac cycle. Abbreviation as in [Figure 2](https://www.sciencedirect.com/science/article/pii/S1936878X24003012#fig2). FT postprocessing begins with defining a region of interest (ROI), usually comprising the endocardial and epicardial borders on short- or long-axis cine images ([Figure 9](https://www.sciencedirect.com/science/article/pii/S1936878X24003012#fig9)). In the following step, the software detects and tracks features and produces a 2D strain map. Myocardial deformation can then be visualized through graphical markers on the image or quantified via strain curves. Three-dimensional data sets allow the simultaneous estimation of radial, circumferential, and longitudinal strain parameters. ## Methodology: Echocardiography ### Tissue Doppler vs speckle tracking Echocardiography is currently the method of choice for strain assessment in clinical practice. Measurements of strain are obtained by STE using dedicated speckle-tracking software during postprocessing of regular image loops. Strain based on tissue Doppler imaging is also feasible in clinical settings but is not widely used, as it requires dedicated acquisitions, is dependent on the angle of insonation, and is more time consuming when analysis in multiple directions and image planes is required. Nevertheless, tissue Doppler imaging enables higher temporal resolution than STE, allows a more reliable assessment of strain rate and a better recognition of short-lived events, and can be faster for a focal analysis of a certain myocardial region. STE is based on tracking bright and dark dots (“speckles”) in the myocardium. Speckles do not represent tissue structure but represent interference patterns that have been shown to move together with the tissue for a limited time and distance so that they can be used as features for tracking.(https://www.sciencedirect.com/science/article/pii/S1936878X24003012#bib20),(https://www.sciencedirect.com/science/article/pii/S1936878X24003012#bib21) In contrast, CMR FT tracks predominantly the endocardial border, which explains some of the differences in results found in direct comparisons of STE and CMR FT ([Table 1](https://www.sciencedirect.com/science/article/pii/S1936878X24003012#tbl1)).(https://www.sciencedirect.com/science/article/pii/S1936878X24003012#bib22) Table 1. Summary of Technical Characteristics of Clinically Used Strain Methods | Empty Cell | CMR FT | 2D STE | 3D STE | | --- | --- | --- | --- | | Dominant features used for tracking | Endocardial borders | Myocardial speckles and contour | Myocardial speckles and contour | | Signal-to-noise ratio | High | Moderate | Low | | Temporal resolution | 30 phases per heart cycle | 40-80 frames/s | 34-50 frames/s | | Spatial resolution | Reasonable (1-2 mm in plane and 8-10 mm through plane) | Good (submillimeter radial; lateral depends on number of beams and image depth) | Poor (at least 3-4 times