Molecules Derived From Amino Acids PDF
Document Details
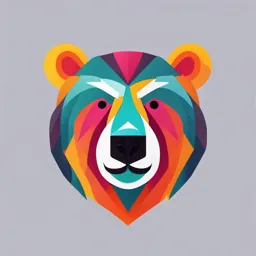
Uploaded by DiversifiedMelodica6625
Tags
Related
- Biosynthesis Of Nonessential Amino Acids PDF
- BIOCHEMISTRY AMINO ACIDS: METABOLISM OF CARBON SKELETON (Part 2) PDF
- Biochemistry Amino Acids Metabolism of Carbon Skeletons Part 2 PDF
- Lecture 8_Biosynthesis of Nucleotides and Amino Acids_PDF
- Harper's Biochemistry Chapter 27 - Biosynthesis of the Nutritionally Nonessential Amino Acids PDF
- Lippincott's Biochemistry Chapter 21 - Amino Acids Conversion to Specialized Products PDF
Summary
This document discusses the molecules derived from amino acids. It details the pathways of amino acid biosynthesis, grouping them into families based on their metabolic precursors. Key molecules like glutamate, glutamine, and porphyrins are also highlighted.
Full Transcript
Contents 678 FUNDAMENTALS OF BIOCHEMISTRY Table 27–1. Essential and nonessential amino acids and the number of enzymes required for their synthesis Nutritionally essential amino acids Nutritionally nonessential amino acids...
Contents 678 FUNDAMENTALS OF BIOCHEMISTRY Table 27–1. Essential and nonessential amino acids and the number of enzymes required for their synthesis Nutritionally essential amino acids Nutritionally nonessential amino acids a Arginine*, 7 Alanine 1 b Histidine* 6 Asparagine 1 Isoleucine 8 (6 shared) Aspartic acid 1 Leucine 3 (7 shared) Cysteined 2 Lysine 8 Glutamic acid 1 a Methionine 5 (4 shared) Glutamine 1 c Phenylalanine 1 (9 shared) Glycine 1 a Threonine 6 Proline 3 Tryptophan 5 (8 shared) Serine 3 Valine +1 (7 shared) Tyrosine +10 50 24 * Arginine and histidine, though belonging to the nutritionally essential category, are sometimes classified as nutritionally semiessential amino acids because they may be synthesized in tissues at rates inadequate to support growth of children. a b c d 2− From glutamic acid From aspartic acid From serine From serine plus S amino acids, which must therefore be supplied by the diet, are termed nutritionally essential amino acids. It may be emphasized that a given amino acid may be nutritionally essential for one form of life but nutritionally nonessential for another. These differences reflect their different genetic heritages. Confusion may arise because nutritionists frequently refer to the nutritionally essential amino acids as ‘essential’ or ‘indispensable’ amino acids and the nutritionally nonessential amino acids as ‘nonessential’ or ‘dispensable’ amino acids. Although in a nutritional context these terms are correct, it is unfortunate that they obscure the biologically essential nature of all the 20 amino acids. A perusal of the Table 27–1 indicates that the number of enzymes required by cells to synthesize the nutritionally essential amino acids is large in relation to the number of enzymes required to synthesize the nutritionally nonessential amino acids. This suggests that there is a positive survival advantage in retaining the ability to manufacture ‘easy’ amino acids while losing the ability to make ‘difficult’ amino acids. Metabolic Precursors of Amino Acids The pathways for the biosynthesis of amino acids are diverse. However, they have an important common feature: their carbon skeletons come from intermediates in glycolysis, tricarboxylic acid cycle or pentose phosphate pathway (Fig. 27–3). The nonessential amino acids are synthesized by quite simple reactions, whereas the pathways for the formation of essential amino acids are quite complex. Ten of the amino acids are only one or a few enzymatic steps removed from their precursors. The pathways for others, such as the aromatic amino acids, are more complex. A useful way to organize the amino acid biosynthetic pathways is to group them into families corresponding to the metabolic precursor of each amino acid (Table 27-2). Contents BIOSYNTHESIS OF AMINO ACIDS 679 Glucose Glusose–6–phosphate Ribose–5–phosphate Histidine Erythrose–4–phosphate 3–phosphoglycerate Serine Glycine Cysteine Phosphoenolpyruvate Pyruvate Alanine Valine Tryptophan Leucine Citrate Phenylalanine Tyrosine Oxaloacetate a–ketoglutarate Aspartate Glutamate Asparagine Glutamine Methionine Proline Threonine Arginine Lysine Isoleucine Fig. 27-3. An overview of amino acid biosynthesis Precursors from glycolysis, the citric acid cycle and the pentose phosphate pathway have been shown enclosed in single-line, double-line and dashed-line rectangles, respectively. Table 27–2. Six biosynthetic families of amino acids, based on different metabolic precursors (shown in boldface) α-ketoglutarate Oxaloacetate Phosphoenolpyruvate and erythrose-4-phosphate Glutamate Aspartate Phenylalanine* Glutamine Asparagine Tyrosine Proline Methionine* Tryptophan* Arginine† Threonine* Isoleucine* Lysine* 3-phosphoglycerate Pyruvate Ribose-5-phosphate Serine Alanine Histidine* Cysteine Valine* Glycine Leucine* * Essential amino acids; rest are nonessential. † Although essential in young, growing animals but not in adults, the status of arginine is rather ambiguous since the entire arginine molecule can be synthesized by human and other animals. Contents 680 FUNDAMENTALS OF BIOCHEMISTRY In addition to these, there is a notable intermediate that recurs in several pathways: phosphoribosyl pyrophosphate (PRPP). PRPP is synthesized from ribose-5-phosphate derived from the pentose phosphate pathway, in a reaction catalyzed by ribose phosphate pyrophosphokinase: Ribose-5-phosphate + ATP l 5-phosphoribosyl-1-pyrophosphate + AMP Ribose phosphate pyrophosphokinase is allosterically regulated by many of the biomolecules for which PRPP is a precursor. PRPP is an intermediate in tryptophan and histidine biosyntheses, with the ribose ring contributing many of its carbons to the final structure of these amino acids. It is also of fundamental importance in the biosynthesis of nucleotides. AN OVERVIEW OF NITROGEN METABOLISM The pathways leading to the biosynthesis of amino acids and nucleotides both share a requirement for nitrogen. But as soluble and biologically-useful nitrogen compounds are scarce in natural environment, therefore ammonia, amino acids and nucleotides are used economically by most organisms. Nitrogen from the environment is introduced into biological systems by many pathways which are described below. Nitrogen Cycle The atmospheric air contains 79% (i.e., about four-fifths) molecular nitrogen (N2). However, only a relatively few species can convert atmospheric nitrogen into forms useful to living organisms. Therefore, different organisms display an interdependent manner to salvage and reuse biologically available nitrogen in a vast nitrogen cycle (Fig. 27–4). Fig. 27–4. The nitrogen cycle The total amount of nitrogen fixed annually in the biosphere exceeds 1011 kg. The first step in nitrogen cycle is nitrogen fixation, i.e., reduction of N2 to ammonia (NH3 + or NH4 ) which is accomplished only by nitrogen-fixing bacteria. Plants cannot reduce but live symbiotically with the bacteria (usually Rhizobium) and thereby enrich the nitrogen content of the soil. Thus, the symbiotic and photosynthetic N2-fixing systems fix a major amount of the total of Contents BIOSYNTHESIS OF AMINO ACIDS 681 8 approximately 10 tons of N2 fixed annually. On the contrary, the free-living and nonphotosynthetic bacteria (Azotobacter, Klebsiella, Clostridium) contribute relatively little to the total. Although ammonia can be used by most living organisms, the soil bacteria, which are so – – abundant and active, oxidize soil ammonia first to NO2 and then to NO3, a process called – nitrification. Plants and many bacteria can easily reduce NO3 to ammonia by the action of nitrate reductases. Ammonia so formed can be synthesized into amino acids by plants which are then used up by animals as source of amino acids, both essential and nonessential, to built animal proteins. When organisms die, the microbes degrade their body proteins to ammonia in the soil, where nitrifying bacteria convert it into nitrite and nitrate again. Certain denitrifying bacteria, however, convert nitrate to N2 under anaerobic conditions, so that a balance is maintained between fixed nitrogen and atmospheric nitrogen. In this process of – denitrification, these soil bacteria utilize NO3, rather than O2, as the ultimate electron acceptor. (a) Electrons from reduced ferredoxin N2 DINITROGENASE DINITROGENASE REDUCTASE REDUCTION (Mo-Fe protein) + (Fe protein) NH4 ATP ADP (b) Fig. 27–5. The 3-dimensional structure of nitrogenase complex (a) Ribbon diagram. Nitrogen is an essential component of many biochemical building blocks. This enzyme complex, shown here, converts nitrogen gas, an abundant but inert compound, into a form that can be used for synthesizing amino acids, nucleotides, and other biochemicals. The dinitrogenase subunits are shown in grey and pink, the dinitrogenase reductase subunits in blue and green. Bound ADP is shown in red. Note the 4Fe-4S complex (Fe and S atoms orange and yellow, respectively), and the Fe-Mo cofactor (Mo Black, homocitrate (light grey). The P clusters (bridged pairs of the 4Fe-4S complexes) are also shown. (b) Schematic diagram. The dinitrogenase reductase component dissociates from the + nitrogenase component before N2 is converted into NH4. Contents BIOSYNTHESIS OF AMINO ACIDS 685 FIXATION OF AMMONIA INTO AMINO ACIDS Ammonia, of whatever origin, can be combined into organic linkage by 3 major reactions that occur in all organisms. These reactions result in the formation of glutamate, glutamine and carbamoyl phosphate. Utilization of the nitrogen of carbamoyl phosphate is limited to two pathways, one contributing a single nitrogen atom in the synthesis of pyrimidines and the other donating one nitrogen atom to the synthesis of arginine. Essentially all other nitrogen atoms of amino acids (or of other organic compounds) are derived directly or indirectly from glutamate or the amide group of glutamine. Although NH3 can be utilized in place of glutamine in some enzymic reactions, glutamine is preferred in most cases. The α amino group of most amino acids is derived from the α amino group of glutamate by a process called transamination. Glutamine, the other major nitrogen donor, contributes its side-chain nitrogen in the biosynthesis of a wide range of compounds. Glutamate is synthesized from NH4 and α-ketoglutarate, a tricarboxylic acid cycle intermediate, + by the action of L-glutamate dehydrogenase which is present in all organisms. This reaction, which is termed reductive amination, has already been dealt with in the catabolism of amino acids. NADPH acts as a reductant in glutamate biosynthesis, whereas acts as an oxidant in its degradation. α-ketoglutarate + NH4 + NADPH Ö + + L-glutamate + NADP + H2O Glutamate dehydrogenase from Escherichia coli (MW = 50,000) is a hexamer of 6 identical subunits. In eukaryotic cells, L-glutamate dehydrogenase is located in the mitochondrial matrix. + The equilibrium for the reaction favours reactants and the Km for NH4 (~ 1 mM) is so high that a modest NH4+ assimilation takes place. Soil bacteria and plants rarely have sufficiently high concentrations of NH4+ and as such not enough glutamate is formed and these organisms have to generally rely on the two-enzyme pathway, described below. The pathway for the conversion (or assimilation) of NH4+ into glutamate requires 2 reactions. + First, glutamate and NH4 react to produce glutamine by the action of glutamine synthetase, which is found in all organisms. Glutamate + NH4+ + ATP → Glutamine + ADP + Pi + H+ In fact, this is a two-step reaction, with enzyme-bound γ-glutamyl phosphate as an intermediate. Glutamate + ATP Ö γ-glutamyl phosphate + ADP γ-glutamyl phosphate + + + NH4 ÖGlutamine + Pi + H Besides its importance for NH4+ assimilation in bacteria, this is a central reaction in amino acid metabolism. It is the main pathway for converting toxic free ammonia into the nontoxic glutamine for transport in the blood. Glutamine synthetase (Fig 27–8), (MW from that of E. coli = 6,00,000), consists of 12 identical subunits and is inactivated by the transfer from ATP of a 5′-adenylyl group to form a phosphodiester linkage with the phenolic hydroxyl group of a specific tyrosine residue of each subunit. At least 6 end products of glutamine metabolism plus alanine and glycine are allosteric inhibitors of the enzyme (Fig. 27–9) and each subunit (MW = 50,000) has binding sites for all 8 inhibitors as well as an active site for catalysis. Each inhibitor alone gives only partial inhibition. The effects of the different inhibitors, however, are more than additive, and all 8 together virtually shut down the enzyme. Thus, glutamine synthetase is a primary regulatory point in nitrogen metabolism. Contents BIOSYNTHESIS OF AMINO ACIDS 707 Fig. 27–24. Control of the activity of glutamine synthetase by reversible covalent modification The activity of glutamine synthetase is also regulated by reversible covalent modification — the attachment of an AMP unit by a phosphodiester bond to the hydroxyl group of a specific tyrosine residue in each subunit. There are two forms of glutamine synthetase; the more active deadenylated form and the less active adenylated form. The adenylated form is more susceptible to cumulative feedback inhibition than the deadenylated form. The covalently-attached AMP unit can be removed from the adenylated enzyme by phosphorolysis. Adenylation and deadenylation both are catalyzed by the same enzyme, adenylyl transferase (AT). It has been observed that the specificity of adenylyl transferase is controlled by a regulatory protein (designated P), which can exist in 2 forms, PA and PD and (Fig. 27–24). Adenylation is catalyzed when the enzyme AT forms a complex with one form (PA) of the regulatory protein. The same enzyme (AT) catalyzes deadenylation when it is complexed with the other form (PD) of the regulatory protein. MOLECULES DERIVED FROM AMINO ACIDS Amino acids are the building blocks of peptides and proteins. They also serve as precursors of specialized biomolecules, such as hormones, coenzymes, nucleotides, porphyrins, alkaloids, antibiotics, pigments, neurotransmitters etc. Some of the leading biosynthetic pathways are discussed below. 1. Biosynthesis of Porphyrins Glycine acts as a major precursor of porphyrins, which are constituents of hemoglobin, the cytochromes, and chlorophyll. The porphyrins are formed from four moles of the monopyrrole derivative, porphobilinogen (Fig. 27–25). In the first reaction, glycine reacts with succinyl-CoA to yield α-amino-β-ketoadipate, which is then decarboxylated to produce δ-aminolevulinate. This reaction is catalyzed by δ-aminolevulinate synthase, a PLP enzyme in mitochondria. Two moles of δ-aminolevulinate condense to form porphobilinogen, the next intermediate. This dehydration reaction is catalyzed by α-aminolevulinate dehydrase. Four moles of porphobilinogen Porphyrias are inherited as acquired disorders come together to form protoporphyrin, through caused by a deficiency of an enzyme in the heme a series of complex enzymatic reactions. The biosynthetic pathway. About 15% of heme iron atom is incorporated after the synthesis occurs in the liver and nearly all of the protoporphyrin has been assembled. remainder occurs in erythrocyte precursors. In humans, genetic defects of certain Hence, the porphyrias are separated into hepatic enzymes of this pathway lead to the or erythropoietic types. All types of porphyrias, accumulation of specific porphyrin precursors except a few hepatic ones, are inherited. Those in body fluids and in the liver. These genetic in which biochemical defect appears to be known include erythropoietic porphyria, acute intermittent diseases are known as porphyrias. In porphyria, hereditary porphyria, porphyria cutanea congenital erythropoietic porphyria, which tarda and lead poisoning. affects mainly erythrocytes, there is an Contents 708 FUNDAMENTALS OF BIOCHEMISTRY accumulation of uroporphyrinogen I, the useless symmetric isomer of a precursor of protoporphyrin. It stains the urine red and causes the teeth to fluoresce strongly in UV light and the skin to be abnormally sensitive to light. Because insufficient heme is synthesized, patients with this disease are anemic, shy away from sunlight and have a propensity to drink blood. This condition may have given rise to the vampire myths in medieval folk legend. The disease is inherited as an autosomal dominant. – Two molecules of COO d-aminolevulinate — — — CH2 2 DEHYDRATION CH2 Succinyl-CoA – – C—S-CoA OOC CH2—COO — — — — O CH2 CH2 + C —— C Porphobilinogen + CH2—NH3 — – —C CH COO Glycine — — CH2 N H — +NH CONDENSATION 1 CoA-SH 3 Four molecules of – porphobilinogen COO — — — — — 3 4 NH+4 CH2 CH2 a-amino- b-ketoadipate 4 C O + 4 CO2 CH—NH3 5 – COO 4 CO2 6 DECARBOXYLATION 1 CO2 CH3 CH CH2 — — — — – C COO C — — — — — CH2 HC—C C CH — — N C C CH3 CH2 CH3—C—C — — C O NH HN — CH2—C—C CH2 — — C C CH CH2 CH2 N — — — — +NH 3 – CH—C C CH COO — — — — d-aminolevulinate C C CH2 CH3 CH2 – COO Protoporphyrin IX Fig. 27–25. Biosynthesis of protoporphyrin IX, the porphyrin of hemoglobin and myoglobin The atoms furnished by glycine are shown in boldface. The remaining carbon atoms are derived from the succinyl group of succinyl-CoA. The enzymes involved in the numbered reactions are : 1 δ- aminolevulinate synthase, 2 porphobilinogen synthase, 3 uroporphyrinogen synthase, 4 uroporphyrinogen III cosynthase 5 uroporphyrinogen decarboxylase, and 6 coproporphyrinogen oxidase. Contents BIOSYNTHESIS OF AMINO ACIDS 709 Acute intermittent porphyria is a quiet different disease. The liver, rather than the red cells, is affected and the skin is not typically photosensitive. Symptoms rarely occur before puberty; they include in order of frequency intermittent abdominal pain, vomitting, constipation, paralysis and psychological symptoms. The urine of the afflicted may have a port wine colour from photooxidation of the porphobilinogen excreted, together with 5-aminolevulinate, in large amounts. As its name implies, the disease is episodic in its clinical expression. The disease is inherited as an autosomal dominant. 2. Biosynthesis of Bile Pigments The normal human erythrocyte has a life span of about 120 days. Old cells are removed from the circulation and degraded by the spleen. The apoprotein of hemoglobin is hydrolyzed to its constituent amino acids. The first step in the degradation of the heme group to bilirubin (Fig. 27–26) is the cleavage of its α-methene bridge to form biliverdin, a linear tetrapyrrole. This reaction is catalyzed by heme oxygenase. The central methene group of biliverdin is then reduced by biliverdin reductase to form bilirubin. Bilirubin binds to serum albumin and is transported to the liver, where it is transformed into the bile pigment bilirubin glucuronide. Bilirubin glucuronide is sufficiently water-soluble to be secreted with other components of bile into the small intestine. Impaired liver function or blocked bile function causes bilirubin to leak into the blood. This results in a yellowing of the skin and white of the eye, a general condition called jaundice. Fig. 27–26. Biosynthesis of billrubin from heme group of porphyrin ( = Degradation of heme to billirubin) 3. Biosynthesis of Creatine and Glutathione Phosphocreatine (PC) is derived from creatine (Cr) and acts as an important energy reservoir in skeletal muscle. Creatine is derived from glycine and arginine, and methionine (as S-adenosylmethionine) plays an important role as donor of a methyl group (Fig. 27–27). Contents 710 FUNDAMENTALS OF BIOCHEMISTRY + NH2 NH3 — — — — — Glycine + CH2 C NH2 COO – NH Arginine (CH2)3 — + CH—NH3 — Amidinotransferase – COO Ornithine NH2 — — — + C NH2 NH Guanidinoacetate CH2 — – COO adoMet Methionine Methyltransferase adoHcy NH2 — — — — + C NH2 N—CH3 Creatine CH2 – COO ATP Creatine kinase ADP – O — — — — — — – O P—O NH + C NH2 N—CH3 CH2 – COO Phosphocreatine Fig. 27–27. Biosynthesis of creatine and phosphocreatine Note that creatine is made from 3 amino acids : glycine, arginine and methionine. This pathway shows the versatility of amino acids as precursors in the biosynthesis of other nitrogenous biomolecules. Contents BIOSYNTHESIS OF AMINO ACIDS 711 Glutathione (GSH) is a tripeptide containing glycine, glutamate and cysteine (Fig. 27–28). The first step in its synthesis is the formation of a peptide linkage between the γ-carboxyl group of glutamate and the α-amino group of cysteine, in a reaction catalyzed by γ-glutamylcysteine synthase. Formation of this peptide bond requires activation of the γ-carboxyl group, which is achieved by ATP. The resulting acyl phosphate intermediate is then attacked by cysteine amino group. This reaction is feedback-inhibited by glutathione. In the second step, which is catalyzed by glutathione synthetase, ATP activates the carboxyl group of cysteine to enable it to condense with the amino group of glycine. Glutathione is a highly distinctive amino acid derivative with several important functions. Glutathione is present in virtually all cells, often at high levels (~ 5 mM), and serves as a sulfhydryl buffer. It cycles between a reduced thiol form (GSSG) in which 2 tripeptides are linked by a disulfide bond. GSSG is reduced to GSH by glutathione reductase. The ratio of GSH to GSSG in most cells is more than 500. Glutathione also plays a key role in detoxification by reacting with H2O2 and organic peroxides, the harmful byproducts of organic life. Glutathione peroxidase 2GSH + R—O—OH → GSSG + H2O + R—OH Glutathione peroxidase, the enzyme catalyzing this reaction, is unique in possessing a covalently- attached selenium (Se) atom. Cysteine Glycine ATP ADP + Pi ATP ADP + Pi Glutamate g-Glu–Cys g-Glu–Cys–Gly g-glutamyl cysteine Glutathione synthase Glutathione (GSH) synthase g-Glu Cys Gly + NH3 O O — – – OOC—CH—CH2—CH2—C—N—CH—C—N—CH2—COO — — — — H CH2 H SH Glutathione (GSH) (reduced) g-Glu–Cys–Gly g-Glu–Cys–Gly Glutathione (GSSG) (oxidized) Fig. 27–28. Biosynthesis and structure of glutathione Also shown is the oxidized form of glutathione (GSSG), which contains 2 moles of glutathione linked by a disulfide bond. 4. Biosynthesis of Neurotransmitters The small diffusible molecules which communicate nerve impulses across most synapses are called neurotransmitters. Many important The word catecholamine is derived neurotransmitters are primary or secondary amines, derived from catechol, which is a fairly recent from amino acids in simple pathways. Besides, certain shortening of the trivial name polyamines, that are conjugated with DNA, are derived pyrocatechol or 1,2-dihydroxybenzene from the nonprotein amino acid, ornithine. (catechol was a more complex The synthesis of some neurotransmitters is illustrated aromatic compound from which in Fig. 27–29. Tyrosine gives rise to a family of pyrocatechol was obtained by heating). catecholamines that comprises dopamine, norepinephrine The nomenclature is unfortunate, but and epinephrine. Levels of catecholamines are correlated it is in common usage. Contents 712 FUNDAMENTALS OF BIOCHEMISTRY with changes in blood pressure in animals. Parkinson’s disease, a neurological disorder, is associated with an underproduction of dopamine. The disease is cured by administering L-dopa. An overproduction of dopamine in the brain leads to psychological disorders such as schizophrenia. An inhibitory neurotransmitter called γ-aminobutyrate (GABA) is produced from glutamate on decarboxylation. An underproduction of GABA leads to epilepsy. GABA is used in the treatment of epileptic seizures and hypertension. Tryptophan, in a 2-step pathway, produces serotonin (= 5- hydroxytryptamine), another important neurotransmitter. + NH3 + NH3 — — – HO— —CH2—CH—COO – OOC—CH2—CH2—CH—COO – Tyrosine Glutamate Tetrahydrobiopterin O2 Glutamate PLP Tyrosine decarboxylase CO2 hydroxylase H 2O + Dihydrobiopterin NH3 — + – HO NH3 OOC—CH2—CH2—CH2 — — – g-aminobutyrate HO— —CH2—CH—COO (GABA) Dopa Aromatic PLP amino acid + NH3 decarboxylase CO2 — – CH2—CH—COO + HO NH3 N NH — Histidine — HO— —CH2—CH2 Dopamine Histidine PLP decarboxylase CO2 Ascorbate Dopamine O2 + b-hydroxylase NH3 — H 2O Dehydroascorbate CH2—CH2 + NH HO NH3 N — — HO— —CH—CH2 Histamine — OH Norepinephrine adoMet Phenylethanolamine N-methyltransferase adoHcy + HO H2N—CH3 — — HO— —CH—CH2 — OH Epinephrine Fig. 27–29. (Cont’d) Contents BIOSYNTHESIS OF AMINO ACIDS 713 + NH3 — – CH2—CH—COO N Tryptophan H Tetrahydrobiopterin O2 Tryptophan hydroxylase H 2O Dihydrobiopterin + NH3 — – CH2—CH—COO HO N 5-hydroxytryptophan H Aromatic PLP amino acid decarboxylase CO2 + NH3 — CH2—CH2 HO N H Serotonin Fig. 27–29. Biosynthesis of some neutrotransmitters derived from amino acids Note that the key biosynthetic step is the same in each case : a PLP-dependent decarboxylation (shaded). Histidine, upon decarboxylation, produces histamine which is a powerful vasodilator found in animal tissues. In allergic responses, histamine is produced in large amounts. It also stimulates acid secretion in the stomach. 5. Formation of Nitric Oxide from Arginine Nitric Oxide (NO), a short-lived signal molecule, is produced endogenously from arginine in a complex reaction that is catalyzed by nitric oxide synthase (NOS); citrulline is the other product. Nitric oxide, a free-radical gas, is an important messenger in many vertebrate signal transduction processes. It diffuses freely across membranes but has a short life span, less than a few seconds, Contents 714 FUNDAMENTALS OF BIOCHEMISTRY because it is highly reactive. Hence, nitric oxide is well suited to serving as a transient signal molecule within cells and between adjacent cells. 6. Biosynthesis of Lignin, Tannin and Auxin The aromatic amino acids, phenylalanine, tyrosine and tryptophan are precursors of many plant substances. Lignin, a complex rigid polymer, is derived from phenylalanine and tyrosine. It is second only to cellulose in abundance in plant tissues. Phenylalanine and tyrosine also give rise to many natural products such as tannins that inhibit oxidation in wines. Tryptophan gives rise to plant growth hormone, indole-3-acetate (IAA) or auxin (Fig. 27–30). This molecule regulates some important physiological processes. Fig. 27–30. Biosyntheis of indole-3-acetate 7. Biosynthesis of Papaverine from Tyrosine The metabolism of most alkaloids is poorly known. Their chemical structure in many cases The term ‘alkaloid’ (which means alkali-like), first proposed by a pharmacist, C.F.W. Meissner in 1819, was originally applied to all organic bases isolated from plants. As this definition included unnecessarily an extraordinary wide variety of compounds, so the definition changed. Later, Landenburg defined alkaloids as natural plant compounds having a basic character and containing at least one nitrogen atom in a heterocyclic ring. His definition noticeably excluded any synthetic compounds and any compounds obtained from animal sources. Some authors specify those alkaloids obtained from plants as plant alkaloids or vegetable alkaloids. They are very poisonous, but are used medicinally in very small quantities. Thus, plant alkaloids are characterized by their basic properties, (usually) complex structures, specific physiological actions and their plant origin. In fact, they represent another group of secondary metabolites, parallel to terpenes or terpenoids. About 4,500 alkaloids have so far been isolated from more than 4,000 species of plants, mostly from dicot families such as Papaveraceae, Apocynaceae, Papilionaceae, Ranunculaceae, Rubiaceae, Rutaceae and Solanaceae : the first one being unusual in that almost all its members contain alkaloids. Among monocot families, they are found in Liliaceae and Gramineae (=Poaceae). Alkaloids are generally found in the roots, bark, stem, leaves and seeds of these plants and are stored in the vacuoles (hence, they do not appear in young cells until they are vacuolated) as salts of various plant organic acids, such as acetic, citric, oxalic, tartaric etc. The alkaloids are usually colourless, crystalline, nonvolatile solids, which are insoluble in water but are soluble in ethanol, ether, chloroform etc. Some alkaloids are liquid which are soluble in water (e.g., coniine and nicotine) and a few are coloured (e.g., berberine is yellow). Most alkaloids have a bitter taste and are optically active (levorotatory). Alkaloids form insoluble precipitates with solutions of phosphotungstic acid, phosphomolybdic acid, picric acid etc; many of these have definite crystalline shapes, hence may be used to help in their identification. The alkaloids are stored in sites other than the site of their synthesis. For example, the alkaloid nicotine is synthesized in tobacco roots but is transported and stored in the leaves. Some alkaloids are even modified at the storage sites. Basically, the alkaloids are synthesized from various amino acids (ornithine, lysine, tyrosine, phenylalanine, asparagine, tryptophan, proline, glutamic acid) or their derivatives. Because of their physiological effects on human systems, alkaloids have been used in pharmacy since time immemorial. Coniine is principle alkaloid of hemlock (Conium masculatum) which was responsible for the death of the great Greek philosopher Socrates in 399 B.C. when he drank the cup of poison– hemlock oil. They act as nitrogen excretory products. They also help in maintaining ionic balance. Besides, they act as growth regulators, most probably germination inhibitors because they have chelating power.