Mouse Anatomy and Pathobiology PDF
Document Details
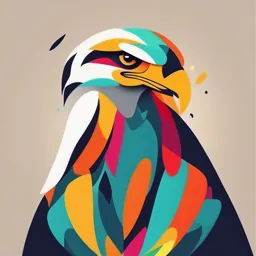
Uploaded by JoyfulComputerArt3149
Universitat Autònoma de Barcelona
2024
Joan Faus Camarena
Tags
Related
Summary
This module details mouse anatomy and pathobiology, focusing on morphological phenotyping. It examines organs like the pancreas and knee joints, and discusses the use of mice as models for human diseases. The module also integrates imaging and histology techniques.
Full Transcript
MODULE I: MOUSE ANATOMY AND PATHOBIOLOGY Universitat Autònoma de Barcelona Joan Faus Camarena 2023-2024 Erasmus Mundus Joint Master’s in Human Diseases Models Morphological Phenotyping (MorphoPHEN) MODULE I: MOUSE ANATOMY AND PATHOBIOLOGY...
MODULE I: MOUSE ANATOMY AND PATHOBIOLOGY Universitat Autònoma de Barcelona Joan Faus Camarena 2023-2024 Erasmus Mundus Joint Master’s in Human Diseases Models Morphological Phenotyping (MorphoPHEN) MODULE I: MOUSE ANATOMY AND PATHOBIOLOGY BLOCK 0: INTRODUCTION Universitat Autònoma de Barcelona Module I UAB MorphoPHEN UNIT 0.1: GENERAL CONCEPTS IN MORPHOLOGICAL MOUSE PHENOTYPING Jesús Ruberte Animal models, not just mice, are important to understand physiopathology and develop new treatments. Indeed, from the thousand drug candidates that are tested, only a few of them reach the clinical phase, and that is why the process is so costly ($1.8 billion average cost for successful discovery and development of a NME (New Molecular Entity) (Paul et al, 2010)). Besides, this is due in part because animal models represent imperfect facsimiles of human diseases, and can react differently to drugs than humans do. Mouse is the most used animal model. More than a third (34/100) of top drugs were enabled using mouse models (Nature Review, 2013). 1. Mouse relevance as a human disease model. Robert Boyle (1662)'s pioneering experiments laid the groundwork for the scientific method and experimental inquiry, which provided the foundation for later scientific research, including the use of animal models. Robert Boyle discovered oxygen. To do some experiments that demonstrated that, he developed a glass ball, where vacuum was produced inside. When they put the mice inside the glass container, the mouse died (there was no oxygen there). Joseph Wright (1768)'s artistic representations of scientific experiments, such as those involving animals in air pumps, highlight the curiosity and exploration of the natural world during the Enlightenment era, including the study of animals in experiments. The mouse, as a versatile animal model, has played a crucial role in scientific investigations, building on the principles of experimentation and scientific discovery pioneered by Boyle and represented in the works of artists like Wright. Mouse is a model good for studying human diseases because: We have similar genetics (95-99% of genetic homology). Similar anatomy and physiology. Similar pathology, and the response to some drugs is also similar (responsiveness to known drugs). A lot of Nobel prizes were awarded to discoveries that involved mice research: from penicillin in 1945 (Fleming), to Capecchi in 2007 with the description KO and transgenic mice, until E. Charpentier and A. Doudna in 2020 for the description of CRISPR/Cas9 to artificially modify DNA (it allows to do very fine things, changing just a base of the genome, allowing personalized medicine to flourish, for example generating avatars). 1 Module I UAB MorphoPHEN 2. MorphoPHEN approach. The intention of the master’s is to put together the knowledge of different departments to have a whole integrated idea of the anatomy and pathology of the mice. Example: The Pancreas When studying an organ like the pancreas, it's essential to begin with an exploration of its macroscopic anatomy. This initial step involves comprehending its orientation within the body, familiarizing ourselves with its various components, and gaining the ability to isolate it effectively. Once we've established this foundation, we can progress to more detailed examinations, including cross-sectional analyses that reveal differences compared to the human pancreas. For instance, it's noteworthy that in mice, the pancreas is in close proximity to the abdominal wall, a distinction from its human counterpart. Furthermore, we can employ various imaging techniques, such as MRI or echography, to visualize the pancreas from different angles and perspectives. This not only enhances our understanding of its structural features but also allows for topographical assessments that may prove valuable for in-vivo monitoring of certain conditions. 2 Module I UAB MorphoPHEN Moving beyond macroscopic anatomy, we delve into the intricacies of the pancreas, focusing on its histological characteristics. This involves the examination of both the endocrine and exocrine aspects, including the production of hormones. Techniques like immunohistochemistry enable us to specifically mark components of interest, such as insulin (typically indicated in green). Additionally, electron microscopy (EM) grants us access to high-resolution images, revealing electrodense and electro-lucid phases within the tissue. Let's illustrate the importance of mouse phenotyping with a practical example: a research group encountered a challenge when investigating a knockout (KO) mouse model for a pancreatic gene, specifically the Socs2 gene, in comparison to a wild-type (WT) mouse. Initially, at the macroscopic level, no discernible differences were apparent between the two groups. Undeterred, they embarked on a comprehensive phenotyping journey that would prove pivotal in unraveling the mysteries of this genetic variant. Their investigative path took them through multiple layers of scrutiny. Beginning at the microscopic level, they diligently examined specific markers within the pancreatic islets, focusing on insulin as a key indicator of function. Concurrently, they probed the vasculature using markers like Collagen IV (CoIIV marker) to gain insights into the microenvironment. 3 Module I UAB MorphoPHEN However, the pivotal breakthrough came when they turned to electron microscopy (EM) imaging. Here, they uncovered a critical divergence: a significant loss of mature insulin granules in the transgenic (Tg) mouse, a finding that signified a crucial discovery. Armed with this newfound knowledge, the research team embarked on a targeted quest to identify the enzyme responsible for granule maturation. Their journey led them to PC1, an enzyme intricately linked to granule maturation, and it became evident that this enzyme was indeed affected in the Socs2 KO mouse. This revelation not only illuminated the role of the Socs2 gene in pancreatic function but also underscored the profound value of mouse phenotyping in biomedical research. In essence, this example vividly demonstrates that mouse phenotyping, encompassing macroscopic, microscopic, and ultrastructural analyses, serves as an indispensable tool in uncovering intricate genetic and physiological nuances that hold the keys to breakthroughs in biomedical science. And that is what scientists are trying to do for example within the International Mouse Phenotyping Consortium (IMPC). 4 Module I UAB MorphoPHEN 3. The Crucial Integration of Imaging and Histology in Disease Research In the pursuit of a comprehensive understanding of human diseases and their manifestation in mouse models, the seamless integration of imaging and histological techniques emerges as an imperative approach. Consider, for instance, the examination of a knee joint – a complex structure that offers profound insights into the intricacies of disease progression. By combining both imaging and histological analyses, we can capture a holistic view of the knee joint, allowing us to juxtapose different scenarios within a single slide. This integration proves invaluable in our exploration of diseases like Type IVA Mucopolysaccharidosis (MPS IV-A), characterized by the accumulation of these molecules in lysosomes, ultimately leading to neurological, bone, and joint disorders. Let's focus on the femur, with its trochlea and condyles, as a prime example. In MPS IV-A, we observe anomalies such as the presence of liquid in the tibiae condyle, indicative of joint degeneration and loss. Turning our attention to histological analyses, we uncover telltale signs of cartilage alterations and the onset of arthritis. Utilizing specialized staining techniques like Safranin O staining, we discern a distinct contrast in chondrocyte textures between the affected mice and their wild-type counterparts. Zooming in further through electron microscopy (EM), we peer into the lysosomes, revealing them to be laden with MPS, underscoring the genetic underpinnings of the condition. The pursuit of such comprehensive phenotypic information is paramount. While compensatory mechanisms may come into play, they are seldom flawless. 5 Module I UAB MorphoPHEN In essence, this integrated approach marries the macroscopic and microscopic, offering a profound synergy that unravels the nuanced progression of diseases. By amalgamating imaging and histology, we fortify our ability to decipher the intricacies of human diseases as they manifest in mouse models, ultimately advancing our quest for therapeutic solutions. Advanced imaging techniques empower us to unveil the most minute anatomical intricacies in mice. One such example is the hyoid bone, nestled at the base of the cranium, and intimately involved in the process of swallowing (deglutition). Despite its diminutive size, comprising just a few thousand cells, modern imaging technology allows us to delve into levels of detail that are comparable to what we can observe in humans. The realm of mouse morphology holds a wealth of untapped knowledge, and the application of imaging techniques serves as a potent catalyst for fresh discoveries. Consider, for instance, an intriguing revelation: within the clitoridis, an organ that, from an imaging perspective, bore a striking resemblance to bone. This revelation challenges conventional wisdom, as it has long been established that the male mouse possesses a bone in its glans, but scientists did not know that females also had a bone. Shared embryonic origin of the clitoris and the penis: during the critical window of 18 days post-development, these structures exhibit remarkable similarity. This parallel is noteworthy as it underscores the complex interplay between anatomy and developmental pathways. Notably, the perineum, the anatomical region situated between the genital organs and the anus, becomes a pivotal focal point of examination. 6 Module I UAB MorphoPHEN But they are more than an homologous organ. Within the C57BL6/J mouse lineage, we uncover the intricacies of the preputial orifice of female and its accompanying cavity. Subsequent exploration reveals the presence of preputial ducts (four in number), each unveiling a unique facet of murine morphology. Upon venturing further, by carefully opening the preputial cavity, we unveil the urethral cavity ensconced within the corpus spongiosum. Notably, the dorsal vein of the clitoris, two dorsal arteries, and an intricate network of nerves come into view. The implications of these findings are profound, as they challenge conventional assumptions and underline the remarkable similarity in anatomical components between female and male mice. The unexpected parallels and complexities that emerge from this exploration serve as a testament to the wonders that lie within the realm of mouse morphology. Brace yourself for a journey of perpetual surprises as we continue to unravel the mysteries of the biological world. 7 Module I UAB MorphoPHEN UNIT 0.2: LABORATORY ANIMAL ASSOCIATIONS AND TRAINING P. Vergara - [email protected] In this lecture we are going to see how laboratory animal sciences people are organised, which organisations exist and how they can help to our professional development. Laboratory Animal Science Associations – LASA. These are National associations of laboratory animal science professionals (Vets, Facility Managers, Animal Welfare officers, Technicians, Scientists...). We can join these associations as individuals, it is a good way to be up to date of our topic of interest. Some examples are: European associations SECAL, GV-SOLAS, SPCAL, RusLASA, PolLASA, AISAL, LASA, NVP, which belong to FELASA, south American associations like SBCAL, AACyTAL, ASOCHICAL, and the north American association AALAS which is the most well-known, is huge and it has a lot of material and information that we can check out. The aim of these associations is to promote the 3Rs. These organisations are used by scientists as a network. For examples, in SECAL (the one in Spain) they have a mail list just in case someone has any doubt related to Laboratory Animal matters; or CompMed™ Community Platform from AALAS. All of the example organization that have been mentioned before, except for AALAS, later joined bigger regional organizations: FELASA for Europe, AFLAS for Asia, FESSACAL for South America, or FeSAHANCCCAL in Central America. As far as she knows, there is no regional associations in Africa. However, there are some LASA in Tunisia, Argelia, South Africa… In Nigeria there is an organization called ACURET that organises courses and is very active. 1. FELASA (Federation of European Laboratory Animal Science Associations) - www.felasa.eu. This organization includes 2 European organisation + Israel. The important points of this organization are: They have working groups that publish guidelines that are open access, they are written by experts in the area coming from different countries and positions, thus being consensus papers. For example, when we need to check some condition in our mice in our animal facility, we check FELASA paper about Health monitoring. And we can do the same for identification of animals, genetic monitoring in mice, severity, harm-benefit analysis, Continuous professional development (CPD)… In Europe, legislation in laboratory animal is quite tight, and FELASA is the reference to discuss legal procedures with the Council of Europe, European Commission or EFPIA (European Association of Pharmaceutical Industries and Associations), as it represent the point of view of people using animals and at the same time welfare and ethics professionals. As a big federation, FELASA has been able to make liaison with other organizations like AALAS, ICLAS, ESLAV… Education and Training. In Europe, you have to get a training if you want to work with laboratory animals. Education and training is a key aspect of FELASA's mission to support professional competence in personnel working with animals, the implementation of the 3Rs leading to the conduct of ethical and high quality science and the mobility of researchers within the EU. The FELASA accreditation scheme encourages and assists the development of high-quality educational programmes in laboratory animal science (LAS) throughout Europe and internationally. FELASA accreditation was introduced on 1st of January 2003 and is recognised as the premier accreditation scheme in Europe for LAS courses. 1 Module I UAB MorphoPHEN The scheme is in line with the EU Directive (EU Directive 2010/63, Article 23) and the EC guidelines on education (European Commission, 2014) requiring that education in LAS is to be mandatory in all EU countries and is regulated by Competent Authorities. In this EU Directive 2010/63 “Categories” of training were replaced by “Functions”. At the beginning of the 2000s FELASA defined what a FELASA course is, because everybody was using FELASA name to promote their courses, and now there are some rules to get an accreditation like that. Most courses of FELASA are in Europe, but there is also one in India and one in South Africa. 1.1. Laboratory Animals (www.lal.org.uk). FELASA, ESLAV and some National Associations use Laboratory Animals as their journal. They publish a new issue every 2 months and publish all of most of FELASA guidelines open access. They are kind of an NGO; they win money and use it for courses and other granted programmes. Unlike FELASA, AALAS (www.aalas.org) gives individual certifications if you follow their courses, I understood that you can do them online, and you don’t need to attend in person. 2. Veterinarians’ organizations. The organizations we have talked about are open for every scientist, but veterinarians have other organisations. In Europe we have ESLAV (European Society of Laboratory Animal Veterinarians) which every veterinarian can join and ECLAM (European College of Laboratory Animal Medicine) for diplomates that have passed a training and an exam. In America there are other organizations like this one. 3. ICLAS – International Council for Laboratory Animal Science. This global organization started in 1950s from the need to define and standardised the results that they were getting from laboratory animal experiments. It is somehow part of the UNESCO, and it has a very complex structure, which includes scientific organisation, institutional organisations, and country representatives. Patricia was a scientific representative there from the Spanish LAA SECAL. It is dedicated to advancing human and animal health and well-being by promoting the ethical care and use of animals in research worldwide. It is not possible to be part of ICLAS as an individual. It is easier to become part of it if you are an organization of scientists because you have to pay a fee. They work in different programs: laboratory animal quality network (it includes health monitoring and genetic monitoring programmes, the latter coordinated by her), and an educational programme totally regionalised (they put money in each region depending on the demands for courses). 4. OIE (Office International des Epizooties) – World Organisation for Animal Health. This Organization is a WHO for animals and published the Terrestrial Animal Health Code. This kind of directives include a chapter that was written by ICLAS people for the use of animals for research and education, and it is a good base to define the legislation for new centres and organisations in counties that they don’t have yet one of them. Most countries are members of this Organization. 2 Module I UAB MorphoPHEN 5. ETPLAS – Education and Training Platform for Laboratory Animal Science. When the current directive for courses was published in 2010, it was not possible to define that everybody needed to have a certification in every country to work with laboratory animals because education was not a competence of the European Union. Several steps were taken to harmonise that, and the European Commission created a structure to give information about courses, this being ETPLAS. EU member states, course organizers, and accreditation bodies must be there, but it is a loose structure. It is interesting for us because there are some free courses that give certifications. 6. Education and training requirements to work with laboratory animals in the EU. In Europe, to become “competent” to work with laboratory animals, there are some steps you have to take. Depending on the function you are going to exert, you’ll have to gain some competences or others. Apart from the theoretical training, some functions require a Work Under Supervision to become competent. This WUS only has to be done when you are working directly with the animals, for example the WUS is not necessary to design an experiment. The aim of the directive was not to define a syllabus, but to tell what a technician should know when they finish the training, it is like a learning outcome list. Functions that the Directive 2010/63/EU defines are: A: carrying out procedures in animals. B: designing procedures and projects. Related to research, but there is no need to touch the animals, so WUS is not necessary. C: taking care of animals. D: Killing animals (euthanasia). Designated Veterinarians with expertise in laboratory animal medicine (Article 25). Functions of people in charge of oversee the welfare and care of the animals (Article 24). The order of the letters is different in Spain, so always look at the definition of the function. 3 Module I UAB MorphoPHEN There are certain learning outcomes that are needed for every function, which are called Core Modules. Apart from that, every function has its own specific modules you have to know to get the accreditation. There are some modules that are specie specific and have to be taken if you want to work with a certain species. You may need to add species or functions to your curriculum, and this can be done because the courses are modular and can be checked in ETPLAS. Besides, there are also courses to update competences, because depending on the country and the function you have to be re-evaluated every some years. 4 Module I UAB MorphoPHEN Although all of the countries talk about harmonisation, there are actually a lot of difficulties in trying to recognise the courses that have been done in a country in another country. For instance, the Italian government does not recognise FELASA, so people that want to work with animal models there have to do a specific Italian course. In other countries they may not make you repeat the course but only to study the legislation part. The training can be achieved following the courses from universities which can be consulted in ETPLAS website or other FELASA accredited Courses. The Autonomous University of Barcelona (UAB) also offers a course. Training course in Laboratory Animal Science for scientists responsible for the design or conduct of animal experiments – Course for functions A, B, C and D. Species according to needs: mouse, rat, rabbit, pig, sheep, zebrafish, xenopus, cephalopods... Laboratory Animal Science and Welfare – A specialization program in Laboratory animal Science for veterinarians and other professionals. 5 Module I UAB MorphoPHEN UNIT 1: COMPARATIVE MOUSE vs HUMAN ANATOMY J. Ruberte Mouse is the ideal animal model because they have similar genetics, physiology and pathology and responsiveness to known drugs, but most importantly because we have a similar anatomy. Despite the fundamental difference in size between humans and mice, a remarkable similarity in our anatomical features becomes evident upon closer examination. This shared morphology suggests the possibility that these anatomical features may serve comparable functions. Consider, for instance, the case of the Lesser trochanter, a structure designed to facilitate the insertion of gluteal muscles. In mice, this anatomical feature exhibits a notable enlargement, reflecting their quadrupedal nature. It's a fundamental principle observed in all quadrupeds—the development of gluteal muscles is accentuated compared to their bipedal counterparts. It's important to recognize that these subtle changes in morphology are intrinsically tied to corresponding alterations in physiology. Interestingly, some similarities between species can be quite unexpected. Take, for instance, the clavicle—an anatomical structure absent in many animals, including cows. The clavicle plays a pivotal role in enhancing mobility in the thoracic region and facilitating arm movement. Remarkably, mice possess a notably large clavicle, featuring a body and two distinct ends. But what's the purpose behind this? Mice, it turns out, are avid climbers. Recent research, employing video recordings within cages to delve into their behaviour, sheds light on their unique eating habits. It appears that mice use their hands to consume food, equipped with specialized pads that enable them to manipulate and transport their meal. This illuminates the function of their clavicle—providing essential support for this distinctive feeding behaviour. However, despite these intriguing similarities, it's essential to remember that a mouse's anatomy diverges significantly from that of a human. Nowhere is this contrast more apparent than in the brain. In this context, the complexity of cortical folds stands out as a defining characteristic in humans. Mice, on the other hand, are considered lisencephalic, lacking the intricate cortical folding observed in our species. In essence, while we can uncover remarkable commonalities between different species, such as the intriguing adaptation of the clavicle in mice, it's equally essential to acknowledge the stark anatomical disparities that set us apart, particularly in complex structures like the brain. This recognition enhances our understanding of the rich tapestry of life's diversity. 1 Module I UAB MorphoPHEN 1. Temporal acquisition of anatomical knowledge. Over the course of history, our understanding of human anatomy has steadily expanded. Pioneering figures like Galenus in 130 A.D., Leonardo da Vinci in 1506, and Julen Bouglée in 1899 made significant contributions to this growing body of knowledge. However, it's important to note that this journey was far from linear, and we cannot claim to have reached the pinnacle of understanding even today, even though we know almost anything. Surprisingly, despite the long history of anatomical exploration, the first comprehensive anatomy book dedicated to mice didn't emerge until 1965 (Margaret J. Cook). What makes this fact even more intriguing is that it wasn't authored by an anatomist. Another book is “Anatomy of the Small Laboratory Animals” (P Popesko 1992) which have good drawings but sometimes are not based in the reality. Others useful books are “Comparative Anatomy and Histology. A mouse and Human Atlas” (PM Treuting, 2012 and 2018), and “Morphological Mouse Phenotyping: Anatomy, Histology and Imaging” (J Ruberte, 2017). In essence, we find ourselves in a fascinating juxtaposition—a contrast between two species characterized by similar anatomical complexity yet starkly different levels of anatomical understanding. 2. Anatomic variation. Anatomic variation, distinct from pathology, can be characterized as deviations from the established norm in individual anatomical form. Pathology, on the other hand, is directly linked to diseases and medical conditions. The concept of anatomic variation implies that the morphological patterns can differ not only across various species but also among individuals within the same species. While extensively studied in humans, this area of research remains relatively unexplored in mice. Consider the radial fossa, for instance. In mice, a supratrochlear foramen exists, facilitating particular functionalities. Interestingly, this foramen can also occur in some human individuals, with the highest incidence noted in India, particularly among women. What purpose does this foramen serve? It enables hyperextension. Importantly, the presence of this foramen in humans is not standard but rather a unique occurrence. The world of anatomy has often been perceived as an unquestionable realm. However, it's essential to recognize that anatomical knowledge is not without its own nuances, particularly when it comes to the methods employed in its acquisition. One such example is the bulbourethral glands, which play a role in seminal fluid production. An interesting historical journey sheds light on this. Initially, these glands were absent from descriptions by M. Cook (top drawing, 1965), only to resurface in the illustrations of the same women two decades later (bottom drawing, 1983). 2 Module I UAB MorphoPHEN This sparked a cascade, as subsequent authors began replicating these newfound depictions. Notably, Jackson's laboratory produced a remarkable book on laboratory mice in 1941, featuring illustrations by Fekete that included two bulbourethral glands and two diverticula. This illustration, too, was widely adopted. The year 2004 and 2012 brought no significant alterations to this representation. Even in 2011, Contastinescu maintained the depiction of two bulbourethral glands and two diverticula. 3 Module I UAB MorphoPHEN However, the reality, as revealed through anatomical dissection, differs. Precisely, there are two bulbourethral glands, with the diverticulum nestled within certain muscles. A comprehensive examination using specialized techniques unveiled a single substantial diverticulum, a finding corroborated by MRI. It's important to underscore that some anatomical references altogether omit any discussion of the bulbourethral gland. In essence, this narrative illuminates the dynamic nature of anatomical knowledge, emphasizing that challenging established assumptions and conducting rigorous anatomical dissections remain essential in our quest for a comprehensive understanding of the human body. If we don’t know the anatomy, we cannot do a diagnostic. 4 Module I UAB MorphoPHEN 3. Anatomical Nomenclature There is a difference between nomenclature and terminology. Nomenclature is “a normalized system of exactly defined terms arranged according to certain classification principles”, which are often devised by experts. Embracing nomenclature is essential for clear communication and mutual understanding. For instance, consider the arteries stemming from the aortic arch, as illustrated in MJ Cook's work from 1965. What humans commonly refer to as the "brachiocephalic trunk" takes on the name "Innominate artery" in the context of mouse anatomy. These distinct terms reflect the intricacies of nomenclature. In the early 20th century, there existed a staggering 50,000 anatomical terms, many of which were eponyms, named after their discoverers. This led to considerable confusion, with identical structures bearing various names. To resolve this naming tangle, anatomists of the era devised the "nomina anatomica," effectively streamlining the terminology to 7,635 terms for human anatomy and 6,500 terms for veterinary anatomy. While mice lack an equivalent "nomina anatomica," the Mouse Genomics Informatics, originating from Jackson's lab, introduced the Adult Mouse Anatomy Browser. It comprises approximately half the number of terms found in the Terminologia Anatomica, totalling 3,300 terms. To bridge the gap between mouse and human anatomy, they link each term with a corresponding number. However, the endeavour encounters challenges, as roughly half of these terms lack direct counterparts. However, people are reluctant to advance in this basic research, very important to make coherent conclusions in other research fields. Let's consider the case of the carpus: In the MGI database, the falciform carpal bone is included within the carpus. However, there are also sesamoid bones found within tendons, and in mice, there are two of them: the falciform carpal bone and the ulnar sesamoid bone. These sesamoid bones are not part of the carpus. The central carpal bone is typically fused to other bones in the carpus. Only 15% of mice have an independent central carpal bone, showing intrastrain variation. In 40% of cases, it is fused to the capitate bone, and in 45% of cases, it is fused to the scapholunate bone. Furthermore, the order of bone names in the MGI database does not follow the anatomical or morphological conventions. Names should be entered from proximal to distal and from medial to lateral (as Versalius already did). Thus, to close these gaps more in-depth mouse anatomical research is needed, and it will be necessary to extent and refine the current vocabulary of mouse anatomical terms. Considering all of these factors, it's important to acknowledge that MGI is the best database in the field of mouse research. Nevertheless, we must offer constructive criticism to help it improve. 5 Module I UAB MorphoPHEN 4. Introduction to Anatomy The anatomical position serves as a valuable reference point for different body parts. In mice, this position corresponds to the quadrupedal stance, with the head facing forward and the neck extended. Within this framework, we can establish three primary planes of reference: Sagittal/median planes: These planes encompass both the median (central) and paramedian (non-central) orientations. Subsequently, we can further categorize these into cranial (toward the head) and caudal (toward the tail). In human anatomy, cranial signifies superior, while caudal denotes inferior. Here, cranial signifies anterior and caudal, posterior. The median plane passes through the sagittal suture. Transverse/axial planes: These planes run perpendicular to the sagittal planes. They allow us to make distinctions between medial (closer to the median plane) and lateral. While many medical professionals describe transverse planes as horizontal, in the context of the mouse, they are actually vertical. Sometimes, transverse sections are also referred to as axial. Micro-CT image of the cranium. 6 Module I UAB MorphoPHEN Dorsal/coronal planes: These planes follow the dorsal part of the animal, known as the dorsum. In human anatomy, they are referred to as frontal or coronal planes due to their alignment with the coronal suture and the presence of the frontal bone in that area. Additionally, ventral and dorsal in humans are synonymous with anterior and posterior. Human Mouse Vertical. Vertical. Sagittal Planes Define cranial (superior) and caudal (inferior). Define cranial (anterior) and caudal (posterior). Also Axial. Vertical, perpendicular to the surface. Transverse Horizontal, parallel to the surface. Define medial and lateral. Planes Define medial and lateral. Also Coronal or frontal. Horizontal, parallel to the surface. Dorsal Planes Vertical, perpendicular to the surface. Define ventral (inferior) and dorsal Define ventral (anterior) and dorsal (posterior) (superior). 4.1. Directional terms and planes: Head: as everything in the head is already cranial, we use another terminology: o Dorsal and ventral: Referring to the superior part of the cranium (frontal bone, continuation of the dorsum) and inferior part of the cranium (mandibula), respectively. o Rostral/oral and caudal/aboral: referring to the more cranial and caudal parts. Extremities: o Proximal and distal: These terms describe the proximity of the structure to the trunk. 7 Module I UAB MorphoPHEN Hand: o During mouse development, the hand moves. Its parts can be described as dorsal or cranial (due to evolutionary movement from dorsal to cranial) and palmar (volar), ventral, or caudal. o The number of fingers also proceeds from medial to lateral. However, it's important to note that medial and lateral fingers differ between humans and mice: the thumb is always the 1st one, because is the more medial in quadrupedal species. o Digits in the foot are better developed than in the hand. There is an axis situated between the 3rd and 4th digits. Those facing the axis are referred to as axial, while those not facing it are considered abaxial. 8 Module I UAB MorphoPHEN UNIT 2. ANIMAL TRANSGENESIS: FROM THE CLASSICS TO THE CRISPR GENOME EDITING A. Pujol 1. Applications of animal models. Transgenic technologies play a vital role in biotechnology and biomedicine studies, offering various applications: 1. Animal Models for Disease Research: Animal models are indispensable for investigating the genetic contributions to pathological mechanisms and testing new diagnostic and treatment methods for diseases in both humans and animals. 2. Due to the lack of spontaneous mutant models for many diseases, genetically modified animals have been pivotal in creating animal models for conditions such as obesity, neurological diseases, and Parkinson's. 3. Development of Therapies: Gene Therapy: Transgenic animals are instrumental in demonstrating the viability of new therapies. For example, researchers have explored gene therapy for diabetes by engineering muscle cells to secrete insulin, regulating blood insulin levels. This concept was tested using a transgenic animal with a construct containing the insulin gene under the MLCp promoter, demonstrating the muscle's response to hyperglycemia. 4. Drug Production: Animals can serve as bioreactors to produce specific molecules, such as growth hormone (GH) or interleukines, which can be harvested from urine or milk. 5. Xenotransplantation: Transgenic animals have applications in xenotransplantation, where animal organs are transplanted into humans. Pigs are commonly used due to their similar organ size. Researchers are working to humanize pig organs by modifying cell surface molecules responsible for organ rejection. They are also used to do basic research, basically in studying Gene Function: Transgenic animals are essential for studying gene function, particularly for newly discovered sequences resulting from projects like the Human Genome Project. Finally, transgenic animals have also been used to do Animal Production Enhancement: Transgenic animals find application in improving livestock characteristics, making them more marketable by enhancing features like growth rate and milk quality. Although, many animal species have been modified, most transgenic animals until now are mice. They have specific features that make them more useful than other animal species: Life span relatively short (2.5 years), allowing to endure experiments during all the life of the animal. Short gestation (21 days), big litter size (8-12) and short generation time (3 months), which enables us to have a lot of animals in a short period of time, useful to plan experiments. We can work with several inbred and outbred strains for different experiments. Housing cost is cheap compared with other animal species. Apart from those logistical reasons, there are two more reasons that make mice the species of choice: Mice genome is over 90% identical to human, so results in mice can be translated more or less to humans. Classical transgenic technologies themselves have been more efficient in mice than in other animal species. Because of that, most of the animals that have been genetically modified have been mice. However, with genome editing technologies the genetic modification of other species is also successful. 1 Module I UAB MorphoPHEN 2. Tools used in animal model generation. Working with transgenic animals involves utilizing two essential tools: 1. DNA/mRNA: DNA and mRNA are fundamental molecules found within the nucleus of eukaryotic cells. They carry vital genetic information for both individual cells and the entire organism. Genes contained within DNA undergo transcription and translation processes to produce proteins, which are responsible for determining an organism's phenotype. The translation of genetic information from DNA to proteins is facilitated by the universal genetic code. This universality allows researchers to transfer genes from one species, such as a jellyfish, into another, like a mouse, with relative ease. 2. Preimplantation Embryos: Preimplantation embryos serve as the foundation for creating transgenic animal models. These embryos progress through various developmental stages, starting from a single-cell embryo, advancing to 2-cell, 4-cell, and 8-cell stages, and ultimately forming a morula before reaching the blastocyst stage. Throughout these stages, the embryos are enveloped by the zona pellucida, a protective layer safeguarding them from potential contaminants. After reaching the blastocyst stage, the embryo undergoes hatching and is ready for uterine implantation. Consequently, beyond the blastocyst stage, embryos cannot be sustained in culture, necessitating the use of a surrogate female for further development. 3. Definition of transgenic animal. Transgenic animals are genetically modified animals carrying experimentally introduced external DNA or mutations in all of their cells, including the germline. That is why we can produce a colony from a mouse already that contains the modification. Animal transgenesis either means: Transferring DNA into the animal or altering DNA already in the animal. We have to consider that there are other kind of transgenesis that do not fit in this definition. 2 Module I UAB MorphoPHEN 4. Type of transgenic animals and technologies. Considering the earlier definition, transgenic animals can be categorized into two distinct types: Addition of New Genes: o The primary technique employed for this purpose is Gene Addition, a classical method. This involves the microinjection of DNA constructs into 1-cell embryos. o These animals are referred to as Conventional Transgenic Animal Models and were first described in the 1980s, typically through microinjection into single-cell embryos. Gene Alteration: o Gene alteration can be achieved using two technologies: ▪ Mutagenesis in Embryonic Stem (ES) Cells: This method permits targeted genome modification and is known as Gene Targeting in ESC, another classical technique. It involves introducing genetic changes in ES cells, followed by the injection of these modified cells into blastocysts. ▪ Mutagenesis by Genome Editing: This approach utilizes tools such as ZFN, TALENS, or CRISPR/Cas9 to manipulate 1-cell embryos directly, resulting in the production of Knockout (KO) or Knock-in (KI) animal models. o Animals generated through these techniques are commonly referred to as KO/KI animal models. Remember that classical technologies are DNA microinjection in 1-cell embryo (which leads to conventional transgenic animals) and Gene Targeting in ESC (which leads to KO/KI animals). 3 Module I UAB MorphoPHEN 4.1. Gene Addition – DNA microinjection in one-cell embryos: Transgenic Animal Models. The first transgenic mouse, producing growth hormone (GH) in response to zinc exposure, was generated in 1982. A team led by Richard Palmiter and Ralph Brinster fuse elements of a gene that can be regulated by dietary zinc to a rat growth hormone gene, and inject it into fertilized mouse embryos. The resulting mice, when fed with extra zinc, grow to be huge, and the technique paves the way for a wave of genetic analysis using transgenic mice. One-cell embryo pronuclear microinjection: This technique involves injecting a solution of the construct into the pronuclei of a one-cell embryo, consisting of two parts: o Promoter: Specifies where (tissue) and how (regulated by hormones or diet) and when the gene will be expressed. o Coding sequence: Determines the product that will be produced. We obtain one-cell embryos and inject into the male pronucleus (coming from the spermatozoa) a solution containing the construct that we want to inject. With the injection pipette we go through the zona pellucida, plasmatic membrane and pronuclear membrane and inject the solution there (we see how it swells). After the microinjection, we transfer the embryos into surrogate females that will give birth to different animals. These animals will have the transgene inserted in their DNA. During the procedure, random insertion occurs. The location (single point) and number of copies of the inserted construct are random, as the construct solution contains various sequences with different copies. Since insertion usually happens before the first cell division (first embryo cleavage), the generated offspring (F1) will possess the construct in all body cells (somatic and germline). However, sometimes the insertion can be after the first embryo cleavage. In this situation, we obtain F0 mosaic individuals, that are not good to quantify the number of copies of the transgene have been inserted. In this case, we will have to mate the transgenic mouse with a WT mouse, obtaining a F1 of heterozygous non-mosaic mouse. Typically, only the founder animal is used to establish a colony. Applications: This technique is employed for: 1. Gene overexpression. 2. “de novo” gene expression. When we want to express a gene in a tissue, organisms or time where it is not usually expressed. 3. Knockdown: partial silencing of an endogenous gene expression. There are technologies that express transgenes that have product (proteins) that can degrade the mRNA of a gene, leading to reduced final production of the product. 4 Module I UAB MorphoPHEN Limitations: o Random insertion can result in positional effects and insertional mutagenesis. We must test the possibility of insertional mutagenesis in the haunters that we obtain. To do so, we will analyze the phenotype of the transgene from two different lines (colonies obtained from each of the F1 individuals – they will have different insertion points and number of copies of the transgene – by mating each of the F1 offspring with a WT mouse). If the phenotype coincides in both lines, then we can say that the phenotype is due to the expression of the transgene and not due to other possible mistakes like insertional mutagenesis. To do an experiment, you should use animals from line 1 and 2 separately and controls. In cases with a genetic hybrid background, Wild Type (WT) siblings are the best control group, so the genetic background it is not a variable that can bother us. o It does not achieve complete suppression of endogenous gene expression. Because of this, Gene Targeting in ESC was described. 4.2. Genetic alteration – Gene Targeting in ESC: KO/KI Animal Models. This method relies on Homologous Recombination (HR) between an external DNA vector and a specific endogenous locus. It enables the production of Knockout (KO) and Knock-in (KI) animals. Gene Targeting in ESC was described in 1986. Doctor Martin Evans investigated how to maintain ESC pluripotency (capacity of ESC to differentiate and produce all the somatic/germline cells in an organisms) in in vitro culture. Two other researchers, Mario Capecchi and Oliver Smithies were studying Homologous Recombination. This HR was described in bacteria, and they discovered its occurrence in eukaryotic cells as well. For this discovery they received the Nobel Prize in Medicine and Physiology in 2007. KO and KI Animals: KO animals result from silencing a specific genomic locus, achieved by deleting part of the DNA sequence or inserting irrelevant DNA sequence information to disrupt the expression of the gene. The targeting vector has homology arms (the piece of sequence marked in a X) that surround the Neo selection gene. When HR occur, the endogenous exons are eliminated from the genome and are replaced by the selection gene, producing a KO animal. KI animals involve altering the genomic locus of interest by a one-for-one substitution of DNA sequence information or by addition of sequence information not found in said genetic locus (gene reporters, transgenes, LoxP sequences, TAG signals…). A gene knock-in can be viewed as a gain-of-function mutation, while a gene knockout represents a loss-of-function mutation. However, a gene knock-in may also entail substituting a functional gene locus for a mutant phenotype, resulting in some loss of function. 5 Module I UAB MorphoPHEN We can produce two kinds of KO/KI animals: Constitutive: the gene is modified during all the life of the animal and in all the tissues of the animal. However, it has limitations: o Interference of the neo selection gene. The expression of this gene can also alter the expression of genes flanking the locus of interest, that finally ends up altering the phenotype of the individual. o Early lethal phenotypes. o Adaptation/compensation, sometimes we obtain the KO animal and has no visible phenotype because other gens have supplied the function of the modified genes. o Complex phenotypes, as the gene is altered in all the cells of the animal, and the same gene can have different functions in different cell types, we can have complex phenotypes. To address these limitations, researchers developed conditional, tissue-specific or inducible mice using recombinase systems. Conditional tissue specific/inducible: In these animals we decide in which tissue we want to alter a specific gene (tissue specific) or when in the life of the animal we want to alter the gene (inducible). In this way, we are able to suppress the expression of the gene in a specific cell type and in the moment of interest. Cre-LoxP system of recombinases is usually used to generate these animals. To do so, we have to generate two types of animals: - Constitutive KI animal (floxed): contains LoxP geneomic sequences (with around 30nt) in intronic sequences of the gene we want to silence, flanking an important exon of the gene. It contains the genetic modification in all the cells of the organism. However, as the modification is in intronic sequences, the expression of the gene is not altered (there are no exons affected). - Conditional animal: it will express Cre recombinase (recombines LoxP sequences) under a promotor that could be tissue specific (if we want to suppress the gene with exons flanked by LoxP sequence in a specific tissue) or inducible system (we can induce the expression of Cre whenever we want). When we mate both animals we will obtain a transgenic animal that has in all the cell of the organisms the gene of interest we want to KO with exons flanked with LoxP, but Cre will be produced only in the cells in which the chosen promoter of Cre is active or when we want to be active. In this situation, Cre protein will be expressed, travel to the nucleus, recombine LoxP sequences and the exon will be cleaved, generating a KO of that specific gene. 6 Module I UAB MorphoPHEN Gene Targeting in ESC technique HR in mammals is infrequent (1:1,000,000), making it challenging to find modifications in one embryo among many /it is like to find a needle in a haystack). Hence, ESCs are preferred as they can be cultured in large numbers. 1. The targeting vector (with selection genes: Neomycin resistance, and Thymidine Kinase – generates toxic product when exposed to ganciclovir) is introduced in a dish with millions of ESC (Embryonic Stem Cells). 2. The cells in which HR (Homologous Recombination) has occurred are selected using a drug (neomycin, + selection; ganciclovir, - selection). ESC with the introduced targeting vector become resistant to the drug. 3. Surviving cells (ESC that have experienced HR) are amplified through mitosis. 4. The selected and amplified ESC are then used for blastocyst injection. Blastocysts already have their own ESC without genetic modification (the inner mass cells). We have to go through the zona pellucida, the trophectoderm and reach the cavity of the blastocyst where the ESC with the knockout (KO) or knock-in (KI) modification are introduced. After injeciton, the blastocyst collapses and one hour later (in vitro culture) the cavity appears again, and the ESC appear together with the blastocyst’s inner cell mass cells. 5. This process results in chimeric animals, which are animals whose bodies contain two types of cells: those with and those without the genetic mutation (cells with different genetic backgrounds). 6. These chimeric mice are bred with Wild Type (WT) mice. If the modified ESC introduced in the chimeric mice colonize the germline cells (introduced gene is present in the germline), it results in heterozygous modified offspring. These offspring inherit the mutation from both the chimeric animal and the WT animal. If two heterozygous mutant mice are mated, homozygous mutant mice can be obtained. These are mice with two copies of the mutation. ESCs used in this process are from male (XY): when we inject male mutant cells into male blastocyst, we obtain fertile male modified chimeric animals. On the contrary, if we inject male ESC in female blastocyst other things can happen, and we have to check if the obtained females can transmit the modification to the offspring. 7 Module I UAB MorphoPHEN Challenges: Despite significant advancements in genetic engineering techniques, Embryonic Stem Cells (ESCs) continue to play a pivotal role in gene targeting. While gene targeting in ESCs has proven highly effective in mice, it faces challenges when applied to other species due to the difficulty of maintaining pluripotency in these ESCs. For these non-mouse species, an alternative technology known as Somatic Cell Nuclear Transfer (SCNT) is employed. SCNT, famously used in the creation of Dolly the sheep, involves the use of a somatic cell, typically a fibroblast, for introducing genetic modifications. The nucleus of this somatic cell is then transferred into an enucleated oocyte. Following oocyte activation, it develops into an embryo, which must subsequently be transferred into a surrogate mother for gestation. However, it's important to note that SCNT has a notably low efficiency rate, which significantly limits its practicality. Consequently, the generation of genetically modified animals, beyond mice, using this technique has been exceptionally rare. This limitation was overcome with genome editing technologies, that also allow to modify specific locus. 4.3. Genetic alteration – Genome editing; Endonuclease microinjection in one-cell embryos: KO/KI Animal Models. At the end of the 90s and the beginning of the XXI century the cite-specific nucleases were described. First meganucleases, Zinc-Finger nucleases, and TALENS, finally, at the end of 2013 CIRSPR/Cas9 was described. CRISPR/Cas9 can be designed to make specific double strand DNA breaks. That activates the natural DNA repair mechanism of the cells: Homologous Recombination, Homologous Direct Repair, and Non-Homologous End Joining. All of these natural DNA repair mechanisms of the cells can be used to do genome editing. Dr. Francis Mojica (Universitat d’Alacant) described this method in bacteria and called it Clustered Regularly Interspaced Short Palindromic Repeats and CRISPR-associated protein 9 (left photo). He described as an immune system for bacteria. It was Drs. Emmanuella Charpentier and Jennifer A. Doudna, together with Dr. Feng Zhang, who described the possibility to use this immune system of bacteria to edit the genome in mammalian cells (right photo). In 2020 Emmanuella Charpentier and Jennifer A. Doudna were awarded the Nobel Prize in Chemistry “for the development of a method for genome editing”. They demonstrated that Cas9 could be guided to specific target sequences in the larger and more complex mammalian genomes where, as in bacteria, it cleaved the DNA, and the repair mecanisms could be used for genome editing. 8 Module I UAB MorphoPHEN CRISPR/Cas most used system is a ribonucleoprotein consisting of a guide RNA (gRNA= crRNA+TracrRNA) and the endonuclease Cas9, derived from Streptococcus pyogenes. The specificity of CRISPR/Cas9 is determined by: - a 20b sequence (crRNA or sgRNA): guide RNA that will define the genomic locus where the cut will be produced. - the presence of a PAM sequence (Protospacer-Adjacent Motif) in the target DNA. Depending on the species of the nuclease, the PAM sequences changes. In the case of spCas9, the PAM is a trinuclotide 5' NGG 3' (N any nucleotide) and it is essential for the Cas9 target DNA binding. Cas9 is directed to the genic locus by its guide RNA (complementary to the genomic sequence) and makes a DNA double-strand break 3bp upstream of the PAM sequence. After that, the double-stranded DNA is broken and has to be repaired. It can be done by NHEJ or HR. NHEJ: the DNA polymerase adds random nucleotides, introducing a genetic alteration at that point and thus generating a KO. HR/HDR: a donor DNA can be used to repair the break. This donor DNA contains two homology arms with sequences complementary to the flanking regions of the double-strand break, and in between there is a sequence that we want to insert in the braking point. If HR occurs between the homology arms and the homologous sequencing the new DNA material will be inserted, generating a KI. The advantage of CRISPR/Cas9 compared to Gene targeting in ESC is that it can do double strand breaks that increase HR probability to occur to 1:10-100, and that allows us to work directly in one-cell embryos (zygotes). 9 Module I UAB MorphoPHEN Advantages of Genome Editing: High efficiency of genome edition vs gene targeting in mESC. Possibility to induce from small to big genomic deletions/insertions. Possibility to modify genes of the Y chomosome (not achieved by gene targeting in mESC). Possibility to modify multiple genes and in their two alleles (homzygous) at the same time using guides for different genes. It has been described the possibility to knock-out up to 6-7 genes in the same embryo. Donor DNA for CRISPR very simple vs targeting vector for gene targeting in ESC. Shorter homologous arms, not selection genes (neo, TK). Generation of KO/KI by pronuclear/cytoplasmic microinjection or electroporation of one cell embryos, avoiding ESC. With microinjection we have to manipulate the embryos one by one, while using electroporation we can manipulate a large number of embryos at the same time. Important reduction of time needed to generate heterozygous mutations (KO/KI). That turs out to be not true actually. Although vector constricution and ESC culture (gene targeting) takes a lot of time and the Cas9 preparation is quick, the mice (F0) that we obtain using CRISP/Cas9 are mosaics. After the F0 obtention, we have to segregate the mutation of interest and that takes more time. GT in ESC: 1 year CRISPR/Cas9 in 1-cell embryo: 4-6 months. The animals after 1-cell embryo injection are mosaics because the Cas9 is active until 8-cell embryo. The Cas9 can do different cuts in different chromosomes and blastomeres. We must mate our chimeric mice with WT mice and segregate the variations that it contains. Possibility of genome editing of all the animal species and cell lines. No limitation by genetic background, avoiding backcrossing. All of these species in the image were genetically modified using CRISPR/Cas9 system the year after the description of the technique. 10 Module I UAB MorphoPHEN All in all, CRISPR/Cas9 system allows us to generate BETTER animal models for human diseases, xenotransplants, and livestock production. For example, Mucopolysaccharidosis IIIA (MPSIIIA) is a rare disease due a mutation in heparan sulfatase gene. Before CRISPR, the mouse models that we generated for this disease were KO mouse models by deletion of exons. However, researchers realized that each patient had different point mutations. After CRISPR, we can produce KI mouse models and other animal species that contain the specific point mutation of each patient (avatars), allowing a more accurate study of each patient. Limitations of Genome Editing: Off-target activity induces mutations at sites other than the intended on-target site. For the generation of animal models, off-target mutations may be gradually eliminated after a couple of generations with selection of on-target mutations. The double-strand breaks in off-target sites are repaired by NHEJ and are very difficult to find. In addition, off-target activity of CRISPR/Cas is much lower in animal than in cultured cells, partially because Cas9 and sgRNA are injected as short-lived RNAs or as protein in embryos instead of as the longer expression plasmids usually used in cell lines. So, if we want to use the system for gene therapy, we have to take that into account. The only way to know that there are no off-target mutations in the cells we are working with is sequencing all the genome of the cell. Mosaicism: denotes the presence of two or more populations of cells with different genotypes in one individual who has developed from a single fertilized egg. It is due to the different Cas9 activity in the different blastomeres up to the 8-cell embryo. o Genome editing after the first embryo clevage. o HR and NHEJ occured in the same or in different blastomeres and in each allele of the locus of interest. o Off-target activity occurred at different time and genome sites. o Random genome insertion of donor DNA. When we obtain mosaic F0 with many genetic alterations in differnt cells, we have to mate them with WT animals to segregate in the F1 offspring each one of the mutations that the mosaic is carrying. We will chose in the F1 the animal with the mutation of interest. It is recommended to phenotype at least 2 different lines from two independent F0 mutants in order to eliminate the effects of possible off-target mutations. The reason to do that is because with the segregations we are selecting the animal for the on- target mutations, but we don’t know if we are still carrying some off-target mutations that can alter the phenotype. If we phenotype two lines separately and the phenotype coincides, we can say that there is no off-target mutation that is not affected our phenotype. Dependence of PAM sequences, whcih limits the number of possible genomic locus to be edited. 11 Module I UAB MorphoPHEN Other applications of CRISPR/Cas: CRISPR/Cas9 has also been engineered to be used as a tool for several other applications other than gene editing. Dead-Cas9 (dCas9) is a Cas9 that cannot do double-strand DNA breaks. However, it is still able to reach the target locus using the guide. Then we can use the dCas9 bound to other elements to transport these elements to specific points of the genome. - Gene regulation: a trans effector can be bound to the dCas9, we can inhibit or activate specific promoters, and this activate or inhibit the gene expression. - Epigenome editing: epigenetic modifiers can change the methylation or acetylation of specific areas of the genomes, and thus affect the gene expression. - Chromatin imaging: fluorescent proteins bound to dCas9. - Base editing: it allows the introduction of point mutations in the genome without the need for double-strand breaks. - RNA targeting: Cas13 is used in this application. It is very important for therapy, as the alteration will not be transmitted to the offspring (we are not modifying the DNA). - Chromatin topology: protein bound to dCas9 that can dimerise with another protein, changing the organization of the chromatin. 12 Module I UAB MorphoPHEN UNIT 3: 3R’S PRINCIPLES FOR ETHICAL USE OF MICE: REPLACEMENT, REDUCTION, AND REFINEMENT Anastasia Tsingotjidou 1. The laboratory mouse (Mus musculus). 1.1. Historical facts. Mus musculus, commonly known as the house mouse, typically has a lifespan ranging from 2 to 3 years. In terms of physical characteristics, males typically weigh between 20 to 30 grams, while females weigh around 35 grams. Historically, mice had a parasitic relationship with humans and were nearly obligatory commensals. They were introduced to Europe from Asia via ships. Their unique ability to be easily transported facilitated their widespread distribution, making them a ubiquitous presence. “Humans and mice were already in close contact at the end of the glacial period, at the time of the Neolithic revolution, i.e., about 12.000 years ago. Archaeological records have confirmed that by the Bronze Age (3.000 years BC) house mice were quasi-obligatory commensals of established farming communities, and as a consequence, they were transported almost everywhere the maritime trade could carry them at that time, i.e., essentially around the Mediterranean.” From “Of Mice and Men” (John Steinbeck). A proof of the presence of mice in old civilizations can be found in a Turkish Greek Temple. One of the ancient Greek gods, temple of Apollo/Zeus. Mice helped the people to defend the territories because they ate the leather clothing of their enemies. “The temple is dedicated to Apollo, who is known in this context as "Lord of Mice" Smintheus. The origin of this title comes from Greek mythology, specifically the Trojan War and the renowned seer and prophet, Calchas. According to the myth, during the Trojan War, the Greek army faced a series of setbacks, and Calchas, a priest and seer of Apollo, interpreted these misfortunes as a sign of the god's anger. Calchas believed that the reason for Apollo's wrath was a curse associated with mice. To appease Apollo, the Greeks built a temple dedicated to him at the location where the Apollo Smintheus Temple stands today. The term "Smintheus" is believed to be derived from "sminthos," which means "mouse" in ancient Greek. Therefore, Apollo was invoked as Smintheus, the god associated with mice, to seek his favor and end the plague of misfortune that had befallen the Greek forces. This association between Apollo and mice is depicted in the miniature statues placed on the temple's stairs, serving as a tribute to this intriguing mythological connection.” 1 Module I UAB MorphoPHEN 1.2. Mouse as Laboratory Animal. Mice and Humans are close Evolutionary speaking, and that makes mouse species very important. In the lab we have created a new species. Clarence C. Little in 1909 was investigating coat colors of mice and had begun inbreeding mice with coat colors of dilute (d), brown (b), and non-agouti (a). His dba strain became the DBA strain still popular today. He also developed C57BL/10, C57BR, C57BL/6, and C57L. Dr. Little established Jackson Labs in 1929 and was a recipient of The Nobel Prize in 1929. Another important person in the creation of the Laboratory Mouse was Leonell C. Strong (1919), a cancer geneticist, who was the originator of the inbred strains A, C, CBA, C3H, BRSUNT, CHI, F, I, JK, H, NH, STR, BDP, and SEC, that we still use now. If we go through the landmarks of history, we can see that the mouse genetics started when scientists started using Mus musculus as lab animals, and exploded with the mouse genome sequencing in the 2000s. 2 Module I UAB MorphoPHEN 2. Historical facts. The history of animal research has its origins with early pioneers like Aristotle (384 322 b.C.) and Erasistratus (304 258 b.C.), predating the Common Era. Galen (129-199), another notable figure, conducted physiology experiments on animals, including pigs, monkeys, and dogs. It's worth noting that in those times, anaesthesia was not employed during these procedures, as depicted in historical images of Galen using pig for educational reasons at 2nd century. FAristotle (left) and Erasistratus (right). Galene, a drawing of some of his physiological discoveries, and an image of an experiment conducted in alive pig. Ibn Zuhr (1162-1094), also known as Avenzoar, made significant contributions by performing tracheotomies on goats. Vesalius (1514-1564), considered the first anatomist, was pivotal in comparing human and animal anatomy. 3 Module I UAB MorphoPHEN Moving forward to the medieval era, Francis Bacon (1561-1626) stands out as a key figure. He developed a scientific method characterized by observation, questioning, hypothesis generation, experiment design, data collection, and conclusion drawing. Bacon is often regarded as the father of scientific methodology. There is a distinction between the scientific method before Bacon, which followed a deductive approach of hypothesizing (1st general principle) and then proving if the hypothesis was true (top-down), and the contemporary scientific method based on Bacon’s principles, which employs an inductive approach (bottom-up). Nowadays, researchers base their studies on hypotheses but first conduct experiments (1st observation → hypothesis) to test the validity of those hypotheses (if the hypothesis is true or wrong), allowing for a more flexible and evidence-based scientific approach. Similarities between the modern scientific method and Bacon's method. 3. The philosophical approach of using mouse as an animal model in the 19th century. The philosophical approach to using mice as animal models has a rich history, involving several influential thinkers. René Descartes (1596-1650) introduced the idea that animals could be utilized for human needs, perceiving them as machines. He not only practiced but also promoted vivisection. In his correspondence, Descartes suggested that understanding animals as mechanical entities absolved people of guilt for their use in various ways. He even developed a Cartesian Conception of an Animal, describing them as "reflex- driven machines" devoid of intellectual capacities. This mechanistic explanation was deemed sufficient for understanding sensation and perception aspects of animal behaviour, which are often associated with consciousness today. Immanuel Kant (1724-1804) advocated for treating animals ethically, even though he didn't believe in direct ethical duties to them. Kant argued that we should treat animals as we do humans, not because of direct ethical obligations but to avoid developing cruel habits that could harm other people. He posited that ethical duties applied exclusively to rational beings, excluding animals from this category. 4 Module I UAB MorphoPHEN Jeremy Bentham (1748-1832) introduced utilitarianism in his book "The Principles of Morals and Legislation" (1789), utilitarianism, which evaluates actions based upon their consequences. Pleasure, or happiness, is the only thing that has intrinsic value. Bentham emphasized using animals in a way that prevented suffering and promoted happiness. His moral philosophy, particularly the principle of utilitarianism, assessed actions based on their consequences. Actions were deemed right if they promoted happiness and wrong if they caused unhappiness. Bentham advocated for equal consideration of animals within a comprehensive, non-religious moral theory, endorsing the use of animals as long as pointless cruelty was avoided. Everyone's happiness counts equally. John Stuart Mill (1806-1873), who continued Bentham's philosophy, emphasized minimizing pain and distress. He believed that the right action in any situation was one that reduced suffering and maximized pleasure and happiness for all parties involved, including animals. Mill argued that the suffering, pain, pleasure, and happiness of animals should be factored into moral calculations. 4. The evolution of animal use in research. Opposition to animal experimentation began in the 19th century in the UK. The Society for the Prevention of Cruelty to Animals (SPCA) was established in 1824 and remains active today. While they advocate against the unreasonable use of animals, there are activists who employ less reasonable methods. Claude Bernard, in his work on experimental medicine, dissected his own dog, which is widely criticized today. However, Bernard also discussed the criteria for a sound scientific theory and the qualities that define a true discoverer. He is credited with early insights into the nature of significant discoveries and their relevance to humanity. In 1859, Charles Darwin published "The Origin of Species," marking a significant milestone. In the late 19th century, the British Cruelty to Animals Act was enacted to regulate animal The Lesson of Claude Bernard, or Session at the Vivisection Laboratory. Léon Augustin Lhermitte, experimentation. Subsequently, major medical 1889 breakthroughs occurred, including Pasteur's development of the rabies vaccine and the discovery of the tetanus antitoxin. 5 Module I UAB MorphoPHEN Tragedies like the sulfanilamide incident, where over 100 people died due to an antibiotic containing toxic diethylene glycol (DEG), highlighted the importance of animal testing. The absence of prior integral animal testing underscored the need for safety evaluations. In 1952, Jonas Salk introduced the polio vaccine, making it widely accessible and benefiting humanity as a whole. 5. Newer approaches. The Universities Federation for Animal Welfare (UFAW) emerged during a period of significant advancements in the field of medicine. In 1959, the organization commissioned Russel and Burch to write a groundbreaking book titled "The Principles of Humane Experimental Technique." This marked the introduction of the 3Rs concept to humanity: Replacement: This emphasizes methods that avoid or replace the use of animals in research. Reduction: It promotes the utilization of methods that allow researchers to obtain comparable levels of information while using fewer animals or obtaining more data from the same number of animals. Refinement: This focuses on employing methods that alleviate or minimize potential pain, suffering, or distress, thereby enhancing the welfare of animals used in research. During the late 1950s and early 1960s, a tragic event known as the Thalidomide disaster occurred, resulting in a birth defect crisis. Over 10,000 people were affected by the use of thalidomide during pregnancy, with approximately 40 percent of them not surviving or succumbing shortly after birth. Those who survived faced a range of severe health issues, including limb, eye, urinary tract, and heart defects. In response to this catastrophe, the U.S. Food and Drug Administration (FDA) recommended the testing of drugs in animals before human use to ensure safety. 6 Module I UAB MorphoPHEN Following the publication of the 3Rs, the Fund for the Replacement of Animals in Medical Experiments (FRAME) was established in the UK in 1969. Concurrently, Peter Singer's book "Animal Liberation" was published, becoming a seminal work for animal liberation organizations. Singer argued for the liberation of all animals, advocating for their freedom. 5.1. Directive 2010/63/EU. Since the Directive 2010/63/EU many things have changed in this area, because there is a law we have to follow. We cannot do any experiment unless it goes through the legal pathway. Directive 2010 /63 was amended in 2019 by Regulation (EU) 2019/1010 to incorporate ambitious goals for reporting and transparency. For example, the Greek legislation on laboratory animal science is based on: Directives and Decisions of the European Commission. The European Convention of the Council of Europe for the protection of vertebrate animals used for experimental and other scientific purposes of the Council of Europe, which offers the scientific and procedural background for the formation of the relevant Directives. 7 Module I UAB MorphoPHEN The key points of the directive 2010/63/EU: This directive introduces several significant elements to the field of animal experimentation. It underscores the importance of having veterinarian support, a vital aspect that was previously lacking. In the past, there were no such requirements, allowing anyone to conduct experiments without the guidance of veterinarians. However, the present directive emphasizes the necessity of implementing a health monitoring program to ensure the well-being of the animals involved. This aligns seamlessly with the principles of the 3Rs. Furthermore, the directive provides guidance on administering anesthesia or euthanasia, both of which are closely related to the principles of Replacement, Reduction, and Refinement (3Rs). Other special elements in the directive are: Designated veterinarian for each establishment Animal welfare body Named person for education and staff competence Improved transparency Ban of the use of Great Apes and Welfare of NHP Determination of method of killing Classification of severity of Procedures Re-use of animals Animal projects only allowed for licence holders Animal experiments are allowed only for special purposes Only when there is no alternative without the use of animals Only when using minimal numbers of animals Only when causing minimal discomfort !3 R’s: replacement, reduction, refinement –obligation of strategy 8 Module I UAB MorphoPHEN The UK 3Rs website holds great significance in the field of animal experimentation. One of its most pivotal statements is, "Scientific excellence and humane use of laboratory animals are inextricably linked." Similarly, the MDC institute in Berlin incorporates the 3R principles on its website, emphasizing their deep connection to animal use. The scientific community's commitment to the 3Rs is exemplified by the reissue of Russell and Burch's "The Principles of Humane Experimental Technique," now titled "The Three Rs and the Humanity Criterion" by the FRAME organization in 2007. The widespread acceptance of the 3Rs is evident on platforms like the Norecopa website, which serves as a platform for advancing these principles. Norecopa introduced the PREPARE checklist, which in turn led to the development of the ARRIVE checklist. Both the ARRIVE and PREPARE checklists are essential tools to ensure responsible animal use in experiments. Following these checklists diligently ensures clarity in experiment protocols, with adherence eliminating any room for criticism. 9 Module I UAB MorphoPHEN 5.2. Alternative approaches. Following the directive's acceptance by society, the support for the 3Rs has led to the development of alternative approaches: 1. Establishment of national laboratories dedicated to validating alternative methods, often with reference to ECVAM (The European Centre for the Validation of Alternative Methods). 2. Introduction of European Union Reference Laboratories tasked with evaluating the suitability of newly developed alternative approaches as substitutes for animal models. 3. Emphasis on avoiding data duplication and promoting the acceptance of alternative methods. These advancements include the creation of mini organs and mathematical models. Consequently, using animals, such as mice, now requires demonstrating to authorities that no other method can yield the same results. The European Commission has initiated a significant effort in promoting Non-animal Methods in Science and Regulation, aiming to phase out animal use by 2030. While this goal may seem ambitious, it underscores the importance of clearly justifying the use of mice and persuading others of its necessity for valid reasons. Several European associations, including the European Animal Research Association, and Understanding Animal Research, advocate for the responsible use of animals and mice in research. They offer valuable insights on presenting research effectively and ethically. And remember: 10 MODULE I: MOUSE ANATOMY AND PATHOBIOLOGY BLOCK 1: BASES OF MOUSE EMBRIOLOGY Universitat Autònoma de Barcelona Module I UAB MorphoPHEN UNIT 4: INTRODUCTION TO MOUSE DEVELOPMENT J. Ruberte In our exploration of mouse development, we first delve into the concepts of the Law of Muller and Haeckel. According to this law, ontogeny, or development, recapitulates phylogeny, or evolution. This means that as we examine the development of different species, such as mice, humans, sheep, and cows, we observe distinct shapes but shared underlying morphological similarities. Recapitulation is the idea that the development of an individual organism (ontogeny) mirrors the evolutionary history of its species (phylogeny); in other words, each stage in development corresponds to an ancestral stage in the evolutionary history of a species. During the course of development, species experience similar phases, particularly those close in evolutionary lineage, with differences typically manifesting towards the end of gestation. We can categorize ontogeny into three key periods: the germinal period, which pertains to early cell development; the embryonic period, where primary organs like somites that will eventually form dermis, vertebrae, muscles, and more, take shape; and finally, the fetal period, were the already formed organs start to mature. It's worth noting that the duration of gestation varies among species, with mice, for instance, having a gestation period of approximately 19.5 days. The formation of the vaginal plug, a result of coagulated seminal fluid, prevents sperm from escaping the vagina, thus increasing the efficiency of fertilization. This moment is referred to as stage E0.5 or 0.5 dpc (days post-coitum). After birth, we use the "P" for postnatal to count the days. To effectively compare embryos at the same developmental stage, we employ a process known as "staging." This involves assessing specific features defined in Theiler stages. 1 Module I UAB MorphoPHEN 1. Fertilization. The female germ cell is known as the oocyte, and it's important to clarify that in mammals, there is no structure called the ovule (haploid female germinal cell); instead, the process is better described as ovocytation. During ovulation, an oocyte is produced (it contains a nuclei 1n and a polar corpuscle 1n), and it is encased by a specialized membrane known as the Zona Pellucida. Surrounding this membrane are follicular cells, forming what is referred to as the corona radiata. 1. Sperm traverse the corona radiata by means of hyaluronidase which creates spaces between its conforming cells. Spermatozoa consist of three parts: the head (containing compacted DNA and a Golgi apparatus), the acrosome (which contains lytic enzymes), and the tail. 2. Adhesion of sperm to ZP proteins of the zona pellucida, especially ZP3. Zona Pellucida mainly consists of four glycoproteins (ZP 1 to ZP 4). ZP 2 and ZP 3 combine to form basic units that polymerize into long filaments. These filaments periodically connect through cross-bridges formed by molecules of ZP 1 and ZP 4. It is estimated that the zona pellucida of an unfertilized mouse egg contains over a billion copies of the ZP 3 protein. When this binding occurs, the sperm's acrosomal reaction is activated (Ca2+ ions entry and activation and release of lytic enzymes), releasing its enzymes that erode the zona pellucida, allowing penetration. When spermatozoa come into contact with the Zona Pellucida, it triggers the release of Ca2+ ions and activates proteases. These proteases degrade the zona pellucida, facilitating the fusion of both membranes. Importantly, ZP proteins are species-specific, ensuring that the correct spermatozoa are recognized. However, in some cases, like donkeys and horses, there can be recognition between spermatozoa of different species. 3. Sperm that have penetrated fuse their membranes with those of the oocyte. 4. Prevention of polyspermy is activated, meaning mechanisms are set in motion to prevent more sperm from entering and causing aneuploidies. Once the first sperm arrives, it closes off, and no more can penetrate. Upon the entry of the first spermatozoon, the cortical reaction takes place. It consists of two phases: o a rapid phase (1) involving the release of H+ ions from the oocyte's interior, causing an influx of Na+ ions to balance the charge. This phase shifts the oocyte membrane potential from -70 mV to +8 mV (depolarization), repelling other spermatozoa. Subsequently, H+ ions re-enter, restoring the membrane potential. o Then, a slower phase (2) ensues, during which cortical granules containing ovastacin near the plasma membrane of the oocyte are released through exocytosis. This creates a new membrane layer between the plasma membrane and the Zona Pellucida, preventing other spermatozoa from entering. The cortical granules content bind to the ZP and hydrolyze it, preventing binding with sperm. In birds, polyspermy, or fertilization by multiple sperm, is normal. Metabolic activation of the oocyte. 2 Module I UAB MorphoPHEN 5. Decondensation of the sperm nucleus, forming the male pronucleus. 6. Completion of the oocyte's second meiosis (previously arrested at metaphase II), resulting in the formation of the female pronucleus. Following these events, the zygote emerges as a cell containing both male and female pronuclei. The second polar body, representing the other haploid set of chromosomes from the germline cell, is still present. The fusion of the male and female pronuclei, called anfimixis, results in a diploid cell (2n), marking the commencement of development. Moles It's essential to distinguish between a mole and an embryo. When two spermatozoa enter the oocyte, and their male pronuclei fuse, an incorrect gestation occurs that is incompatible with life. This is due to a phenomenon called imprinting, where IGF2, a gene, is methylated on the male chromosome, rendering it inactive. If both sets of chromosomes come from the male, the mole forms due to the absence of IGF2 activity. Moles are cystic dilations of chorionic villi. These occur when a zygote is formed with two paternal genomes (two sperm fertilize an ovum without a nucleus), resulting in a zygote with 46 chromosomes of paternal origin, or in diandric triploids, where fetal development is almost nonexistent, and the placenta forms abnormally, creating a mass that occupies the entire uterus and even invades it like a tumor. 2. Germinal period. 2.1. Segmentation: Once the fertilization has occurred, the one-cell egg will start dividing by mitosis. The zygote will travel through the oviduct while it is dividing (generating blastomeres, each “visually-independent” cell inside the zygote). Blastomers divide so quickly that the resulting cells are each time smaller. The mitochondria in the blastomeres are the one from the mother because all the cytoplasm comes from the oocyte. 3 Module I UAB MorphoPHEN The 4-cell egg and 8-cell egg (morula) will form, those cells are independent, there are no cell contacts (each blastomere can give rise to a full individual). Compaction occurs between blastomeres when ovomorulin (a cadherin) allow more contact between cells, triggering also the formation of gap junctions, that allow communication between cells. Morula is the name of the embryo composed of blastomeres from 8 cells on. The compaction of the morula determines two cellular populations: An outer one, beneath the zona pellucida: the outer cell mass (OCM), comprised of polarized cells (organized in a way where we can distinguish what is apical and what is basal) expressing cdx-2. They are connected to each other through tight junctions, giving them an almost epithelial-like configuration and form through symmetric division. The other "inner" population is the inner cell mass (ICM), consisting of non-polarized cells (like a pool of balls) expressing oct-4. They are connected through gap junctions, and form through asymmetric division. 2.2. Blastocyst formation (blastulation). Blastomeres start to introduce Na+ through Na+/H+ and Na+/K+ pumps, triggering the water to inside by osmosis and a new cavity appears (blastocele), while the division rate also increases. This is a blastula or blastocyst, not a morula anymore and happens when the zygote enters the uterus (E3.5). Now we can differentiate two regions: cells around the zygote will form the extraembryonic tissue and it is called trophoblast (outer cell mass) and the inner cellular mass will form the embryoblast. 4 Module I UAB MorphoPHEN 2.3. Bilaminar embryo formation Implantation. In this phase, the cells from the inner cellular mass or embryoblast differentiate into two layers: hypoblast or primitive endoderm (ventral, yellow) Blastocele Trophoblast Blastocele as a thin layer of cells in contact with the blastocele cavity, and epiblast (dorsal, blue). The blastocyst is now free in the uterine cavity and has to adhere to the endometrium. For the implantation to occur, the zygote has to lose the ZP. This phenomenon is called hatching, and it is produced by proteases secreted by the trophoblast cells. The blastocyst is free now and will adhere and go really deep inside the uterus mucosa. Oviduct epithelia have cilia that moves in the same direction. 2.4. Gastrulation (Egg cylinder). After the blastulaiton, the 3rd and final phase of the germinal period is the gastrulation, the formation of the egg cylinder. The gastrula forms from the inner cell mass (smaller cells with