MMD Lecture 11 DNA Replication Transcription and Translation Fall 2023 (1).ppt
Document Details
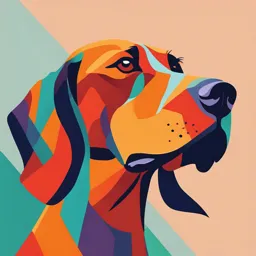
Uploaded by FlatterLogic
Nassau University Medical Center
Full Transcript
DNA Replication and Transcription, Protein Translation Criteria for Genetic Material To serve as genetic/inheritable material, molecule must be able to: Replicate Store information Express information Allow variation by mutation Central Dogma of Molecular Biology Protein as Genetic Material In...
DNA Replication and Transcription, Protein Translation Criteria for Genetic Material To serve as genetic/inheritable material, molecule must be able to: Replicate Store information Express information Allow variation by mutation Central Dogma of Molecular Biology Protein as Genetic Material In the 1940s, geneticists favored proteins as genetic material • Proteins and nucleic acids were major candidates for genetic material • Proteins were diverse and abundant in cells Evidence Favoring DNA as the Genetic Material Was First Obtained during the Study of Bacteria and Bacteriophages Avery, MacLeod, and McCarty (1940s) Avery, MacLeod, and McCarty: First direct experimental proof that DNA is the biomolecule responsible for heredity Griffith’s Transformation Experiment Griffith (1927) Provided foundation for Avery, MacLeod, and McCarty’s research Showed avirulent strains of Streptococcus pneumoniae could be transformed to virulence Speculated transforming principle could be part of polysaccharide capsule or compound required for capsule synthesis Griffith’s experiment demonstrating genetic transformation. RECOMBINATION Living encapsulated bacteria injected into mouse. Mouse died. Colonies of encapsulated bacteria were isolated from dead mouse. Living nonencapsulated bacteria injected into mouse. Mouse remained healthy. A few colonies of nonencapsulated bacteria were isolated from mouse; phagocytes destroyed nonencapsulated bacteria. Heat-killed encapsulated bacteria injected into mouse. Mouse remained healthy. No colonies were isolated from mouse. Living nonencapsulated and heatkilled encapsulated bacteria injected into mouse. Mouse died. Colonies of encapsulated bacteria were isolated from dead mouse. Transforming Principle is DNA Avery, MacLeod, and McCarty: • 1944 published results of their experiment DNase (deoxyribonuclease) utilized to destroy transforming activity • Demonstrated transforming principle was DNA, not protein Hershey and Chase Hershey and Chase (1952) • Used Escherichia coli and bacteriophage T2 • Demonstrated that DNA, not protein, is the genetic material • Used radioisotopes 32P and 35S • Demonstrated DNA enters bacterial cell during infection and directs viral reproduction Complimentary base pairing Adenine base pairs with Thymine via 2 Hydrogen Bonds Cytosine base pairs with Guanine via 3 Hydrogen bonds Uracil will base pair with Adenine in RNA. (Thymine is not present in RNA) The Nitrogenous Bases are attached to a sugar molecule Ribose sugar is used in RNA The Deoxyribose sugar is used in DNA The difference between the 2 sugars is the presences of a hydroxyl group on the #2 Carbon of the ring. OH is present on #2 Carbon in The Carbons of the sugar molecules are numbered as below Notice the #1 Carbon. This is where the nitrogenous base will attach to the sugar. The #5 Carbon is not a part of the ring structure. The #3 Carbon is a part of the ring. • . The Numbered Carbons give us a sense of direction when describing a DNA or RNA Strand The #5 Carbon of one sugar will form a phosphodiester bond with the #3 Carbon of the sugar above it. Riboses attach to other Riboses Deoxyriboses attach to other Deoxyriboses This forms a sugar backbone for a string of Nucleic Phospohodiester Bonds between the 3’ and 5’ Carbons There is a Phosphoester linkage at the #3 Carbon of the molecule above and a phosphoester linkage at the #5 Carbon below. Again, this will result in a chain of nucleic acids with a 5’ end and a 3’ end. Nucleic Acid Chains are antiparallel to each other Adenine binds to Thymine (for DNA) or Uracil (for RNA) Guanine Binds to Cytosine. 2 Complimentary chains associate with each other via the hydrogen bonding of the base pairs. The chains are antiparallel. One chain runs from 3’ to 5’, the complimentary . Eukaryotic DNA Organization Eukaryotic Chromatin consists of the DNA double helix complexed to small basic proteins containing arginine and lysine called histones. Eight histones form an Octamer which is wrapped with 147 base pairs of DNA, forming a Nucleosome. Octamers are connected to each other via a protein called Histone H1. Octamers linked Packing of DNA Octamers form solenoid structures, then coils furthermore into the highly compact and condensed chromosomes. Histone modifications Complex topic • Histones can be modified • Acetylation, methylation, phosphorylation are all possible modifications • In general acetylation is correlated with active sites of transcription Some transcription coactivators have been shown to be histone acetylases Transcription is increased by removing higher order chromatin structure that would prevent transcription Histone Modification and Chromatin Structure Mitochondrial DNA (mtDNA) • The mitochondria has specific Protein subunits of the Electron Transport Chain • Specific ribosomal RNA and transfer RNA’s • These are encoded by a double stranded circular DNA called mitochondrial DNA. • Mitochondrial DNA has matrilineal inheritance as it is originally derived from the ovum. Chromoso mes Chromosomes Strands of DNA that contain all of the genes an organism needs to survive and reproduce Genes Segments of DNA that specify how to build a protein •genes may specify more than one protein in Chromosome maps eukaryotes are used to show the locus (location) of genes on a chromosome The E. Coli genome includes approximately 4,000 ≈4.6 million base pairs genes DNA Replication Replication of DNA will during occur the S (Synthesis) cell replication. phase of The DNA bases on each act as a template to strand complementary synthesize a strands. The process is semiconservative. The new double-stranded DNA template contains and one one old strand new from the synthesized complementary strand. DNA Replication The process is bidirectional. The replication begins at an origin site and simultaneously moves out in both directions. Prokaryotes have one origin site which is AT rich called OriC. DNA Eukaryotes have multiple sites of origin on each chromosome. Replication DNA polymerase adds new nucleotides. DNA ligase joins the DNA segments together. This allows for replication of the much larger eukaryotic genome to occur much more quickly. I. Two rules for DNA Polymerase DNA Polymerase only reads in the 3’ to 5’ direction. II. DNA Polymerase can only add to a new strand already present and do so in the 5’ to 3’ direction. • The Single Template Strand must therefore have a short segment of RNA primer attached to it. This will serve as a starting point for DNA polymerase to attach new nucleotides. This RNA Primer is attached by Primase. Initiation DNA Replication: Initiation •Primase (a type of RNA polymerase) builds an RNA primer (5-10 ribonucleotides long) •DNA polymerase attaches onto the 3’ end of the RNA primer • Initiation: The Fork InReplication Prokaryotes, DnaA binds upstream of OriC and unwinds it in preparation for helicase. Helicase and Topoisomerase Helicases unwind the helix. • • The Single stranded binding proteins keep the strands from associating together again. • Topoisomerase prevent extreme supercoiling of the helix that would result from the unwinding. Topoisomerase: Cleaves and Reseals Topoisomerase I acts to relieve supercoiling stress on the molecule by cutting ONE of the strands on the double helix at the phosphodiester bond. Topoisomerase II acts to relieve supercoiling stress by cutting BOTH strands. This protein uses ATP to do this. DNA Gyrase is a Type II Topoisomerase used in prokaryotes. Ciprofloxacin inactivates DNA Gyrase DNA Polymerases DNA Polymerases differ between Prokaryotes and Eukaryotes. I. Prokaryote Types •pol I- Removes the RNA Primer and fills in the resulting gaps on lagging strand •pol II- DNA replication and repair •pol III- MAJOR replicative enzyme II. Eukaryote Types •DNA Polymerase α- Contains Primase and does replication of DNA on lagging strand (no proof reading) •DNA Polymerase β- DNA repair function (no proof reading) •DNA Polymerase γ- replicates mitochondrial DNA •DNA Polymerase δ- Elongates Okazaki fragments, main Poly on the leading strand •DNA Polymerase ε – DNA elongation and repair functions on leading strand DNA Polymerase The DNA Polymerase enzyme catalyzes the covalent bond between the phosphate of one nucleotide and the deoxyribose (sugar) of the next nucleotide DNA Polymerization DNA Replication: Elongation 3’ end has a free deoxyribose 5’ end has a free phosphate DNA Polymerase READS DNA in the 3’ to 5’ direction to build a new strand. The new strand ELONGATES in the 5’ to 3’ direction. DNA Replication: Elongation Elongation •DNA polymerase reads (3’ to 5’) EACH strand and elongates EACH new complimentary strand (5’ to 3’) DNA Replication at the Lagging Strand REPLICATION Proteins stabilize the unwound parental DNA. The leading strand is synthesized continuously by DNA polymerase. 3' DNA polymerase 5' Enzymes unwind the 1 parental double helix. Replication fork RNA primer Insert Fig 8.5 Primase 5' DNA polymerase 3' Parental strand Okazaki fragment DNA polymerase The lagging strand is synthesized discontinuously. Primase, an RNA polymerase, synthesizes a short RNA primer, which is then extended by DNA polymerase. DNA ligase 3' 5' DNA polymerase digests RNA primer and replaces it with DNA. DNA ligase joins the discontinuous fragments of the lagging strand. • Okazaki Fragment As more and more of the helix is unwound, synthesis on the lagging strand begins from another primer by DNA Polymerase δ • These short fragments formed in this process are called Okazaki fragments. • The RNA Primer will be removed by the nuclease RNase H • The gaps will be filled in by DNA Polymerase δ • The resulting Okazaki fragment will be joined together by DNA Ligase DNA Proof Read by 3’ to 5’ Exonuclease activity • DNA Polymerase III, Polymerase epsilon and delta also have intrinsic 3’ to 5’ exonuclease activity. • If an improperly placed base pair show up, the slight bulge in the chain will activate this feature. • The DNA Polymerase is able to back up the synthesized chain and can hydrolytically remove the incorrect base. • The intrinsic 5’-3’ synthesis activity DNA Mutations High fidelity of replication: about 1 mistake • in 1 billion. • The exonuclease function of the Polymerase enzyme ensures this high fidelity. • Changes not corrected can cause mutations, forever altering the base sequence in daughter DNA. Base substitutions. Parental DNA Replication During DNA replication, a thymine is incorporated opposite guanine by mistake. Daughter DNA Daughter DNA Daughter DNA In the next round of replication, adenine pairs with the new thymine, yielding an AT pair in place of the original GC pair. Replication Insert Fig 8.17 Granddaughter DNA Transcription When mRNA is transcribed from the DNA containing this substitution, a codon is produced that, during translation, encodes a different amino acid: tyrosine instead of cysteine. mRNA Translation Amino acids Cysteine Tyrosine Cysteine Cysteine DNA Damage and Repair Despite proofreading by intrinsic exonuclease activity by DNA Polymerase, errors can still occur. Repairing these errors is essentially 3 steps: I.Removal of the mismatched bases or damaged region II.Filling of the gap by DNA Polymerase III.Ligation of the synthesized segment to the DNA Repair: Repair of UV damage • UV light can covalently join two adjacent thymines on a chain producing a thymine dimer. • The dimerized thymine does not allow the DNA Polymerase to move past it, effectively halting replication. DNA Repair: Repair of UV damage • UV specific endonuclease recognize thymine dimers and cleaves the strand on both sides of the dimer. • Nucleotide excision repair (NER) • The gap is filled in by a DNA Polymerase and sealed up by DNA ligase Xeroderma Pigmentosum • Autosomal recessive inheritance • Malfunctioning NER system • XP-A gene for example • UV radiation and accumulation of error over time • Deficiency in at least one of the DNA repair enzymes • Accumulation of DNA damage • Cancers • Early death The Ends of Linear Chromosome Are Problematic during Replication • At the very end of the linear DNA molecule, (at the 5’ end of the lagging strand), the gap can not be filled in by DNA • Remember, there MUST be something to attach other nucleotides. • Within the chain this is no problem. There are multiple RNA primer segments. • But at the end of the chain there is NOTHING! • CONCLUSION: DNA will gradually shorten during the lifetime of replicating cells Figure 14.21 48 Copyright © 2017 McGraw-Hill Education. All rights reserved. No reproduction or distribution without the prior written consent of McGraw-Hill Education. The Ends of Linear Chromosome Are Problematic during Replication • The DNA has complexes of non-coding DNA on the ENDS of the linear chain called a telomere. • The telomere is a sort of buffer region that provides a lifetime of slack DNA for this inevitable shortening. • Eventually, even the telomere DNA repeats will be consumed, and subsequent DNA replication cycles will start to shorten coding DNA segments. • Senescent: The cell will eventually stop dividing. Telomere Diagram: As cells age Telomere maintenance Telomeres composed of short repeated sequences of DNA Telomerase – enzyme makes telomere section of lagging strand using an internal RNA template (not the DNA itself) Leading strand can be replicated to the end Relationship between senescence and telomere length Activity of Telomerase • High during embryonic development and childhood development. • Lower in adult somatic cells • Except lymphocytes • Evidence: seen in cell culture • Mice model for up to six generations without telomerase activity and eventually no viable offspring • Cancer cells generally show activation of telomerase • Telome re Function: to prevent loss of integrity of the linear DNA Strand. • The telomere is essentially a CA strand and GT complimentary strand tacked on to the end of the linear dsDNA molecule • The GT complimentary strand is longer than the CA strand. • ssDNA a few hundred nucleotide in length at the 3’-end. • Telomer ase Functions as a reverse Transcriptase to preserve the Telomere • Telomerase is a protein that has a CA rich RNA template that will bind to the longer GT rich tail of the telomere. • Telomerase has Reverse transcriptase component that can read itself and synthesize a GT rich DNA fragment (in the 5’-3’ direction) to lengthen the telomere. • The Telomerase will then shift down to the end of the DNA strand it just made and repeat the process to further lengthen the GT rich telomere. • The CA rich telomere strand will also be lengthened by the Primase-RNA Primer- DNA polymerase mechanism as normal. 55 Copyright © 2017 McGraw-Hill Education. All rights reserved. No reproduction or distribution without the prior written consent of McGraw-Hill Education. • Reverse Transcriptase Telomerase is an example of a reverse transcriptase. • It reads RNA and makes DNA (in the 5’ to 3’ direction). • Reverse Transcriptases are seen in Virology. • Examples include the retrovirus HIV which uses reverse transcriptase to transcribe their own ssRNA genome in viral DNA. Retrovirus use reverse transcriptase • Retroviruses such as HIV carries it’s genome as ssRNA. • It uses reverse transcriptase to produce viral DNA (in the 5’ to 3’) direction. • The viral DNA is then incorporated into the host genome. • As the host’s DNA is transcribed, viral RNA is produced. • Eventually yielding new viral proteins. Retrovirus Inhibition • This is an enormous area of research. • Retroviral therapy has many therapeutic targets. • Use of Nucleoside analogs A Modified nucleoside that has had its OH group removed from the #3 Carbon of the deoxyribose ring results in 2’,3’ dideoxyinosine (didanosine). Suppresses the replication of the human HIV reverse-transcriptase inhibitor class. Use of Nucleoside analogs • The modified nucleoside Thymidine has had its OH group removed from the #3 Carbon of the deoxyribose ring and replaced with N3 results in the drug AZT (Zidovudine) Use of Nucleoside Analogs • Chain growth of DNA transcribed by reverse transcriptase can be inhibited by incorporation of these modified nucleoside analogs. • The non-functional nucleoside analogs compete with the naturally occurring nucleotides for incorporation into the viral DNA strand. • The incorporation of non-functional nucleoside analogs stops chain elongation due to its inability to bind RNA •Messenger RNA (mRNA)- is the transcription product of nuclear DNA •Transfer RNA (tRNA)associated with protein synthesis and the translation of mRNA •Ribosomal RNA (rRNA)constitutes part of the machinery of the ribosome RNA is different from DNA • Ribose forms the backbone of the nucleic acid strand for RNA • Uracil is used as one of the nitrogenous bases • RNA is generally single stranded (most of the time) • RNA strand will base pair with itself to give secondary structures • RNA will recognize DNA by base pairing RNA: Extensive base pairing, Secondary and Tertiary Structure…. RNA is different from DNA RNA can act as a catalyst: •Ribozyme activity of small nuclear RNA removes introns and splices the free ends (spliceosomes with small nuclear ribonucleotide proteins) •Ribonuclease activity of some RNA can cleave other RNA molecules (miRNA, antisense RNA, siRNA) •Peptidyl Transferase is a ribozyme which catalyzes the formation of the peptide bond in protein translation • Messenger RNA mRNA is transcribed from DNA. It will serve as a blue- print for generating proteins in the ribosome. • In EUKARYOTES mRNA contains a cap structure on the 5’ end. • This cap is a methylated Guanine Triphosphate attached at the 5’ terminal end of the mRNA. • This cap protects the mRNA from degradation • The 3’ end of mRNA has a Poly adenylated tail (AAAAA……) that also protects the mRNA from degradation in eukaryotes mRNA Ribosomal RNA • rRNA with various proteins forms the ribosome subunits. • rRNA has ribozyme activity in the process of protein synthesis. • rRNA contains loops and exhibits extensive base pairing with itself. • rRNA’s differ between prokaryotes and eukaryotes in terms of their size. • Transfer RNA tRNA carries the amino acid to the ribosome for protein synthesis activity. • tRNA’s are small molecules with 80 nucleotides. • tRNA’s assume a cloverleaf shape RNA Synthesis • Protein synthesis occurs in two primary steps • mRNA (messenger RNA) copy of a gene is synthesized • Occurs in the cytoplasm of Prokaryotes • Synthesis of RNA DNA is transcribed by yet another Polymerase called RNA Polymerase. • RNA Polymerase is responsible for synthesizing a new RNA chain from the information provided by the DNA. • Unlike DNA Polymerase, RNA Polymerase does not need a primer. • Like DNA Polymerase, RNA Polymerase reads the template strand in the 3’ to 5’ direction and Synthesis of RNA DNA used dNTP’s (deoxyribose nucleoside triphosphates) as the precursors for the DNA chain. RNA uses rNTP’s (ribose nucleosides triphosphates) as the precursors for the RNA chain. • Synthesis of RNA in Prokaryotes Prokaryotic RNA Polymerase has 4 core subunits alpha2/beta/beta’ and a fifth unit called sigma factor. • Sigma factor will bind to specific promoter regions on DNA which essentially mark the location of specific genes. • Promoter regions contain the sequence TATAAT box about 10 bases before the start of gene transcription on DNA. • Synthesis of RNA in RNA polymerase recognizes the Prokaryotes promoter and binds • The RNA Polymerase moves along the strand transcribing the DNA Template in the 3’ to 5’ direction. The RNA chain grows in the 5’ to 3’ direction. • The RNA pol. grows the chain by catalyzing the phosphodiester bond • Synthesis of RNA in Prokaryotes RNA Polymerase transcribes only one strand. • This eliminates the need for Okazaki fragments or discontinuous copying mechanisms. • The non-coding strand is the DNA strand that is transcribed. • The coding strand is the strand that is not transcribed. • Supercoiling is avoided by topoisomerases. RNA Synthesis Diagram • Synthesis of RNA in Prokaryotes As the RNA polymerase slides up the DNA template strand, the DNA strand reassociates with its previous analogous DNA strand behind it. • Termination of transcription will occur when the assembled RNA molecule becomes tail heavy. • The end of the RNA molecule will contain a region of repeating GC residues followed by UUUU (4 U’s). • RNA can sometimes base pair with itself, the GC’s do this and form a hairpin loop. • The stress of this configuration is sufficient to destabilize the association of the RNA molecule from the active site of RNA Polymerase. • Alternatively a protein called rho factor disrupts the RNA-DNA association near the 3’ end of the RNA Antibioti cs • Some antibiotics target the RNA polymerase of bacteria preventing adequate catalysis of phosphodiester bond formation between ribose molecules. Eg: Rifampin and quinolones • Inhibits bacterial DNA-dependent RNA polymerase • Inhibits bacterial ligase capacities RNA Synthesis in Eukaryotes mRNA is transcribed from DNA mRNA (messenger RNA) copy of a gene is synthesized Nucleus of eukaryotes RNA Synthesis in Eukaryotic Cells • Is more complicated than in Prokaryotes. • There are separate Polymerases for synthesis of rRNA, mRNA and tRNA. • The Polymerases themselves require various specific and exclusive transcription factors for the RNA Polymerase to become active. • The transcription factors also bind Nuclear RNA Polymerases in Eukaryotes • RNA Polymerase I: involved in synthesis of rRNA in the nucleolus • RNA Polymerase II: involved in the synthesis of mRNA. • RNA Polymerase III: involved in the synthesis of tRNA Transcript ion Initiation mRNA Synthesis 1) INITIATION RNA polymerase binds to a region on DNA known as the promoter, which signals the start of a gene Promoters are specific to genes RNA polymerase does not need a primer Transcription factors assemble at the promoter forming a transcription initiation complex – activator proteins help stabilize the complex Gene expression can be regulated (turned on/off or up/down) by controlling the amount of each transcription factor (eukaryot es) • Promoters and Transcription Factors of RNA Polymerase II II has a The typical promoter for RNA polymerase short initiator sequence, consisting mostly of pyrimidines and usually a TATA box about 25 bases upstream from the start point. • RNA Polymerase II does not recognize the promoter sequence by itself. 1. TFIID finds and binds to the TATA box. 2. TFIIF brings the RNA Polymerase II to the promoter site 3. TFIIH opens the DNA double strand. 4. After an activation step requiring ATP-dependent phosphorylation of the RNA polymerase molecule, the polymerase can initiate transcription at the start point. •Transcription Elongation mRNA Synthesis 1) INITIATION RNA polymerase unwinds the DNA and breaks the H-bonds between the bases of the two strands, separating them from one another Base pairing occurs between incoming RNA nucleotides and the DNA nucleotides of the gene (template) •recall RNA uses uracil instead of thymine AGTC AT UCA GUA • Transcription Termination mRNA 1) Synthesis INITIATION A region on DNA known as the terminator signals the stop of a gene RNA polymerase disengages the mRNA and the DNA Posttranscriptional Modification of • In EUKARYOTES mRNA contains a cap structure mRNA on the 5’ end of the transcript. • This cap is a methylated Guanine Triphosphate attached to the hydroxyl of the 5’ terminal end of the mRNA. • This cap protects the mRNA from degradation • The 3’ end of mRNA has a Poly adenylated tail (AAAAA……) • protects the mRNA from degradation. 5’ Cap and Poly A Tail mRN A Posttranscriptional Modification of mRNA Newly synthesized mRNA will contains unwanted base sequences and is referred to as heterogeneous nuclear RNA (hnRNA) . • The unwanted sequences are called introns. • They are removed from the transcript by a spliceosome protein. • This leaves behind coding sequences called exons. • The remaining exons are linked together. • This process is called splicing. • Splicing occurs before • • Importance of Splicing 15% of genetic diseases are caused by improper splicing. • Improperly spliced mRNA transcripts may leave behind unwanted introns or exclude needed exons. • Sometimes a single mRNA transcript can be responsible for multiple protein products. • The reason this is possible is due to alternative splicing. • Alternative splicing may remove or include some exons or introns to produce Posttranscriptional Regulation • Control of gene expression usually involves the control of transcription initiation • Gene expression can be controlled after transcription with • Small RNAs • miRNA and siRNA • mRNA degradation • Alternative splicing Small RNAs • lin-4 mutant alters developmental timing in Caenorhabditis (C. elegans) • lin-4 does not encode a protein product, instead, it encodes two small RNA molecules • lin-4 RNA acts as a transcriptional repressor of an mRNA • First discovered miRNA; many more found subsequently – currently 1881 known human miRNA sequences • miRNA 1-5% of the human genome • Regulates approximately 30% protein coding genes Micro RNA or miRNA #1 • miRNA production begins with RNA pol II producing a transcript called the pri-miRNA • miRNA folds back on itself to form a stemand loop-structure which is cleaved by Drosha to form pre-miRNA • pre-miRNA exported from the nucleus and cleaved by Dicer to produce a short double stranded RNA containing the miRNA Micro RNA or miRNA #2 • miRNA loaded into RNA induced silencing complex (RISC) • RISC includes the RNA-binding protein Ago, which interacts with the miRNA • RISC is targeted to repress the expression of genes based on sequence complementarity to the miRNA siRNA • RNA interference involves the production of siRNAs • Production similar to miRNAs but siRNAs arise from long doublestranded RNA • Dicer cuts yield multiple siRNAs to load into RISC • Target mRNA is cleaved miRNA or siRNA? Biogenesis of both miRNA and siRNA involves cleavage by Dicer and incorporation into a RISC complex Main difference is target miRNA repress genes different from their origin (non-specific) Endogenous siRNAs tend to repress genes they were derived from (specific) Alternative splicing • Introns are spliced out of pre-mRNAs to produce the mature mRNA • Tissue-specific alternative splicing • Same gene makes calcitonin in the thyroid and calcitonin-gene related peptide (CGRP) in the peripheral, CNS ,hypothalamus Protein Synthesis/Translation • mRNA transcribed from the information coded by DNA is sent to the cytoplasm for translation by ribosomes. • Ribosomes will read the mature mRNA transcript and attach the appropriate amino acid to the growing peptide chain according to the genetic code. • The Peptide chain will undergo enzymatic post translation modification in order to be ready for cellular utilization. The Genetic Code • The genetic code is written in the sequence of the 4 bases of DNA: A, T, C, and G. • The message in the DNA is transcribed into an RNA molecule, and then translated into a polypeptide. • Remember A on the DNA template will have a complimentary base U attached on the mRNA. • Three bases read in sequence on the mRNA, specify one of the 20 amino acids found in protein molecules. A codon is the 3-base sequence for an amino acid. • • • • • Transfer RNA A second type of RNA is transfer RNA, whose function is to attach to a specific amino acid and bring that amino acids to the site where polypeptides are being constructed. This RNA strand is twisted and bonded into the shape seen on the right. One end of the molecule attached to a specific amino acid. The other end has an exposed sequence of 3-bases. These are called the anticodon. Codons and Base Pairs • There are 20 amino acids coded by a 3 base sequence of any of the 4 possible bases A, C, G, U on the mRNA • This means there are 64 anticodons. 4 bases^3= 64 • The anticodons on the tRNAs each have a complimentary codon in the mRNA. • For example the codon AUG would be the compliment of the anticodon UAC. • The Genetic Code There are 64 (4X4X4) possible triplet codes, but only 20 amino acids. • This means that the genetic code is redundant also known as degenerate. • The genetic code is universal across biology and specific codons code for a specific amino acid. Stop and Start Codons • Start signal: AUG • Stop signals: UAA, UAG, UGA • The start signal allows the ribosome to identify the start of an mRNA and to parse the information in 3 codon segments. The start of eukaryotic proteins is AUG and codes for the amino acid Methionine. • Mutations arise from Changes in the • Nucleotide sequence mRNA Silent Mutation: if a codon isof changed (usually the last base), it will often still code for the appropriate amino acid. Thus, the mutation does not affect protein synthesis. • Missense Mutation: If the changed base does change the amino acid sequence a protein mutation may occur. The consequences of this varies from nothing to a slight drop in activity to outright Mutations arise from Changes in the • Nonsense Mutation: if the changed base selects for a Nucleotide sequence ofstop. mRNA termination, the synthesis of the peptide chain will The protein is called truncated. • Splice Site Mutations: Errors in mRNA splicing (posttranscriptional modification errors) can produce protein mutants • Frame Shift Mutations: 1 or 2 Nucleotide deletions or additions can lead to an error in the reading of the mRNA message by ribosome. This causes the entire reading frame to shift. This will lead to mutant proteins and truncated proteins. • Loss or gain of 3 nucleotides does not lead to loss of the reading frame. Sufficient Amino Acids • Protein translation will stop at the codon specifying for an amino acid that is not available. • Essential amino acids are therefore important • Transfer RNA must be The tRNA has an amino acid present attachment site for a specific amino acid at its 3’ end. • The tRNA molecule also has an anticodon which is a 3 base nucleotide sequence which pairs to the codons on mRNA • Some amino acids have more than one specific tRNA. There are more tRNA types than there are amino acids. Aminoacyl-tRNA synthetases This enzyme is responsible for attachment of amino acids to tRNA. The various Aminoacyl tRNA synthetases are very specific for their particular tRNA and amino acids. These synthetases also have proofreading and editing functions. These properties contribute to high fidelity in protein translation. • mRNA must be present The mRNA template must be present and complete. • The 5’ cap protects the mRNA from nucleases • Note: Prokaryotes do not have a 5’ cap. Ribosome The third type of RNA is ribosomal RNA (rRNA) which is a major part of the ribosome. Ribosomes are present in the cytosol or in close association with the rough ER. Ribosomes are the ‘decoding’ units of the cell. They will bring together the mRNA and tRNA Each ribosome consists of a large The subunits are an assembly of and a small subunit. rRNA and proteins and their sizes are given in Svedberg units (sedimentation rate) Ribosomes have binding sites for both tRNA and mRNA molecules. Prokaryotes have a 50S large subunit and a 30 S small subunit. The overall ribosomal size is 70S Eukaryotes have a 60S large subunit and a 40 S small subunit. The overall ribosome is 80S. Tetracycline binds to the 30S subunit blocking the attachment of aminoacyl tRNA to the ribosome. Gentamicin also attaches to the small subunit preventing it’s assembly with the large subunit. The inhibition of protein synthesis by antibiotics. Protein synthesis site Growing polypeptide Tunnel Growing polypeptide 50S 5′ Chloramphenicol Binds to 50S portion and inhibits formation of peptide bond 30S mRNA 50S portion 3′ Three-dimensional detail of the protein synthesis site showing the 30S and 50S subunit portions of the 70S prokaryotic ribosome Messenger RNA Streptomycin Changes shape of 30S portion, causing code on mRNA to be read incorrectly Protein synthesis site tRNA 30S portion 70S prokaryotic ribosome Translation Direction of ribosome movement Tetracyclines Interfere with attachment of tRNA to mRNA–ribosome complex Diagram indicating the different points at which chloramphenicol, the tetracyclines, and streptomycin exert their activities Translation RNA polymerase TRANSLATION Ribosome Met DNA mRNA Ribosomal subunit Protein P Site Leu Met DNA tRNA Anticodon 1 Ribosomal subunit 1 Start codon mRNA Components needed to begin Insert translation come together. 2 Second codon mRNA On the assembled ribosome, a tRNA carrying the first Fig 8.9 (1)amino andacid (2)is paired with the start codon on the mRNA. The place where this first tRNA sits is called the P site. A tRNA carrying the second amino acid approaches. Peptide bond forms Met Met Leu Met Met DNA A site Leu Phe Gly E site 1 mRNA 3 The second codon of the mRNA pairs with a tRNA carrying the second amino acid at the A site. The first amino acid joins to the second by a peptide bond. This attaches the polypeptide to the tRNA in the A site. Ribosome moves along mRNA 4 mRNA The ribosome moves along the mRNA until the second tRNA is in the P site. The next codon to be translated is brought into the A site. The first tRNA now occupies the E site. Insert Fig 8.9 (3) and (4) tRNA released Met Leu Met Gly Leu Phe Gly Growing polypeptide chain Met Phe mRNA Insert Fig 8.9 (5) and (6) 5 The second amino acid joins to the third by another peptide bond, and the first tRNA is released from the E site. 6 mRNA The ribosome continues to move along the mRNA, and new amino acids are added to the polypeptide. et Arg M Leu Met Gly e Ph Met Gl y Polypeptide released Leu Met Gly Met Phe Leu Met Gl Gly y Le u Le u Phe Met Phe Leu Met Gly Arg mRNA New protein Insert Fig 8.9 (7) and (8) mRNA Stop codon 7 When the ribosome reaches a stop codon, the polypeptide is released. 8 Finally, the last tRNA is released, and the ribosome comes apart. The released polypeptide forms a new protein. Protein Synthesis • Translation Termination Ribosome disengages from the mRNA when one of the 3 stop codons enter the A site. Release factors recognize stop codons. This results in hydrolysis of the bond linking the peptide to the tRNA at the P site. • Polysom es Multiple ribosomes can translate mRNA simultaneously. • When multiple ribosomes are simultaneously translating a single mRNA, the entire complex is called a polysome. • Regulation of Translation Most gene expression is regulated at the Transcription level. • Translation regulation does happen to a lesser extent. • Phosphorylation of initiation factors (causes its inhibition) is one way of controlling protein translation in eukaryotes. • Regulation of protein translation can Translational Modification • Modification of a Protein after its release from the ribosome is called post-translational modification. • If still attached to the ribosome, it’s called cotranslational modification. • Modifications can include removal of part of a translated amino acid sequence or addition of various functional chemical groups. • Translational Modification Trimming: Large proteins are often just a precursor to their active form. Trimming of the molecule therefore occurs to produce an active protein. • Trimming may take place in the endoplasmic reticulum or Golgi. • Others are trimmed in secretory vesicles or even after cellular secretion. • An example of this idea we already learned about are the digestive zymogens. • Translational Modification Covalent attachments may serve to activate or inactivate proteins. • Phosphorylation of OH groups on various amino acid residues of proteins is affected by Kinases. Dephosphorylation is affected by phosphatases. • Phosphorylation can increase or decrease the activity of a protein Translational Modification • Glycosylation of proteins involves the addition of carbohydrate chains (non-polar) to the protein. • This is usually in preparation for the protein to become a part of a plasma membrane or be secreted by a cell. Protein folding • Proteins folding is important to their biologic activity. • Misfolded proteins may be tagged for destruction by ubiquitin and subsequent proteolysis by proteasomes in the cytosol. • Folding of the protein can be spontaneous (a function of its design) or facilitated by chaperone molecules. Protein Degradation • Proteins are produced and degraded continually in the cell • Lysosomes house proteases for nonspecific protein digestion • Proteins marked specifically for destruction with ubiquitin • Degradation of proteins marked with ubiquitin occurs at the proteasome