Midterm 1 New PDF
Document Details
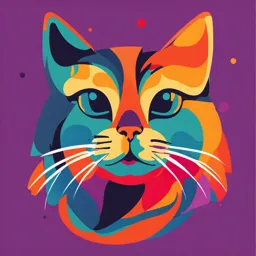
Uploaded by BullishPinkTourmaline4379
Tags
Summary
This document covers the basics of nutrition, defining it as the science of how the body utilizes nutrients for energy, growth, and function. It details the composition of the human body, introducing nutrients and their roles in bodily functions. The document discusses the importance of evidence-based nutritional guidelines and the challenges in establishing them.
Full Transcript
Nutrition is a science that studies how the body can use nutrients to obtain energy, to promote tissue growth, and/or to regulate function at the cell, tissue, and whole-body levels. It encompasses food composition, ingestion, digestion, absorption, and transport of nutrients, functions of nutrients...
Nutrition is a science that studies how the body can use nutrients to obtain energy, to promote tissue growth, and/or to regulate function at the cell, tissue, and whole-body levels. It encompasses food composition, ingestion, digestion, absorption, and transport of nutrients, functions of nutrients, and disposal of by-products of metabolism.The material compiled in this book focuses essentially on evidence-based science to look at nutrition. Knowledge from anatomy, physiology, biochemistry and epidemiology is integrated to understand nutrition and its application to humans under healthy and diseased conditions. There is still a lot that we do not know; therefore, in many instances we will have to use the knowledge with caution. Also, we should never forget that individual responses to food have to be taken into account when looking at food choices. Thus, the "one-size fits all' approach will likely fail. The challenge is to identify the diet that works the best for each individual. The science of nutrition is currently undergoing a revolution. This is because the body of evidence that supports many of the nutritional guidelines provided to the general population originates from studies over long periods of time. The responses obtained from these questionnaires are then used to establish wi veck and or the nur cienie hatroy on opted in it inter in thy hase cain, correlations with the type/frequency of disease occurrence and mortality rates. The lack of precision in these questionnaires compounded by the extreme difficulty in controlling intervening variables raise major concerns regarding the quality of the data. Additionally, these epidemiological studies are essentially correlational and do not prove causality. The food industry also makes things worse because it funds researchers, creates biases, and influences public guidelines for profit. Often, animal activism, climate change issues, and religion beliefs make the basis for defending specific diets. This has only created more confusion and deviated the focus of nutrition away from disease prevention and health promotion. In this confusing environment, the most valuable studies are the randomized clinical trials (RCTs) in which the researchers apply nutritional interventions to groups of people and test the effects of specific foods/nutrients on heath outcomes. These studies allow more control of intervening variables, provide superior quality of data, and are more powerful in establishing causality. However, RCTs are expensive to conduct, time consuming, and the logistics required for them to be successfully completed is often prohibitive. Because of these obstacles to conduct RCT, it's often argued that the epidemiological data are the most abundantly available; therefore, they should constitute the main source of information to base nutritional guidelines. This is a dangerous approach because it assumes that the data are acceptable when scientists know they are not. The bottom line is that health of the population has deteriorated in the last decades and this has been attributed to bad eating habits, bad science, and misleading information provided to the public. Composition of the Human body - The human body is composed of approximately 27 of the currently known 100 existing elements. Oxygen, carbon, hydrogen, and nitrogen are the most abundant atoms accounting for 63%, 18%, 9%, and 3%, respectively, of the foundation of molecules that make up the human body. In humans, water accounts for about 55% to 65% of total body mass, whereas proteins and lipids together contribute 30% to 40%. Nucleic acids, carbohydrates, and other organic molecules add up to about 1% and minerals account for about 5% of the remaining body mass (Table 1). To recycle and maintain the different components that make the human body, we look into the different classes of nutrients found in foods, which are: carbohydrates, lipids, proteins, vitamins, minerals, and water. Carbohydrates, proteins, and fats are called macronutrients because the body needs large amounts of them. Vitamins and minerals, on the other hand, are required in relatively small amounts and are called micronutrients. Nutrients can also be classified as essential and non-essential. The former must be obtained through the diet because the body cannot make it either at all or in adequate amounts to sustain normal body function. The latter can be fully provided by endogenous synthesis if not obtained from dietary sources. Carbohydrates: Are made of carbon, hydrogen, and oxygen. The ratio of carbon to water is typically 1:1. Thus, a carbohydrate literally means "carbon with water". Starches and sugars found in grains, vegetables, legumes, and fruits are the main forms of dietary carbohydrates, and represent a major source of fuel for the body. Dietary fiber is also a carbohydrate, but it does not provide energy for cells. It is very important for normal function of the gastrointestinal (GI) tract. Dairy products also provide some carbohydrate and meat contains very little of it. Our body converts most of the dietary carbohydrate into a simple sugar called glucose? In fact, it is glucose that we find circulating in our blood. Glucose is taken in by cells and used for various purposes including energy production or storage as glycogen. Skeletal muscles and liver metabolize glucose to generate energy, although these organs also store large amounts of glucose as glycogen. The brain relies mostly on glucose for its energy needs and depends on a steady supply of this molecule from the circulation to function properly. However, availability of food is restricted (eg. prolonged fasting or starvation), the brain adapts to use ketones (a fat-derived substrate) as a source of energy. Lipids: Lipids are compounds soluble in organic solvents such as acetone, ether, and chloroform. It refers to fats, oils, and other fatlike substances such as cholesterol and phospholipids that are found in food. Triacylglycerols (TG) make up by far the largest proportion of lipids consumed by humans. The non-water miscibility of lipids requires that these compounds undergo specialized processing during digestion, absorption, transport, storage, and utilization. Besides serving for storage (adipose tissue) and production of energy, lipids are also important for insulation, padding of internal organs, cell signaling, cell membrane formation, synthesis of hormones, and for carrying fat soluble vitamins (A,D, E, and K). Also, humans do not have A-12 and 4-15 desaturases and cannot make 18-carbon ∞-3 and c-6 polyunsaturated fatty acids (PUFAs), which are by nature essential fatty acids. Thus, true essential fatty acids for humans are: Linoleic acid (18:2 c-6) and Linolenic acid (18:3 w-3). Proteins: Are organic compounds made of smaller building blocks called amino acids. Besides carbon, oxygen, and hydrogen, protein also contains nitrogen (N) and sulphur (S). About half of the dry weight of a typical human cell is attributable to protein. Structural components, contractile filaments, antibodies, transporters, neurotransmitters, hormones, and enzymes are made of proteins. Approximately 140 amino acids are known to exist in nature; however, only 20 are genetically coded via mRNA and incorporated into various proteins. Human proteins also contain modifications of a few of these amino acids. Such modifications take place after the protein is initially synthesized. This is also called "post-translation modification". Humans are unable to synthesize 8 to 9 amino acids, either at all or in adequate amounts to meet the body's need for growth and maintenance of tissues and organs. Therefore, these are called essential amino acids (lysine, tryptophan, methionine, valine, phenylalanine, leucine, isoleucine, threonine, and for infants, histidine). Minerals: Are inorganic substances that are essential to health. They can be divided into macro-minerals (needed in relatively large amounts) and micro or trace minerals (needed in small amounts); Calcium, sodium, chloride, potassium, phosphorus, and magnesium are examples of macrominerais, whereas iron, zinc, copper, selenium, iodine, and fluoride are examples of micro (trace) minerals. Vitamins: Organic compounds that contain carbon, hydrogen, and perhaps nitrogen, oxygen, phosphorus, sulphur, and other elements. Vitamins are non-caloric substances and are required in very small amounts. The term was originally coined to describe a vital amine (nitrogen)-containing component of food. However, as scientists discovered that many of these compounds did not contain nitrogen, the "e" was dropped from the word vitamine, converting to the more familiar name vitamin. Either water- or fat-soluble vitamins exist. Importantly, for a substance to gain the status of a vitamin it must be organic and play an essential role in at least one necessary chemical reaction or process in the human body. Also, a vitamin cannot be made in the human body, either at all or in sufficient quantities to meet the body's needs. However, some exceptions to this rule exist, since vitamin D, which can be made by the human body, and two others, vitamin K and biotin, which can be made by bacteria living in the large intestine, are listed in this class of compounds. H20: From a chemical point of view, water is the simplest nutrient; however, it can be argued that it is the most important nutrient. We can survive far longer without any of the other nutrients in the diet than we can without water. Estimated volumes of body fluid compartments for a 73 kg man with 40 L of total body H20: - Intracellular volume → 24 L (60%). This refers to all the water that is localized inside of cells (intracellular compartment). - Extracellular volume → 16 L (40%). Refers to all the water that is localized outside of cells (extracellular compartment). The extracellular compartment can be further divided into: Interstitial volume → 11.2 L (28% of total body water). Plasma volume → 3.2 L (8% of total body water) Transcellular volume → (4% of total body water) Overview of the Digestive system: - Tongue and teeth - Salivary Glands (Parotid, Submandibular, and Sublingual) Oesophagus (25 cm) Stomach (25 cm) Mouth - Fundus - Body - Antrum - Oxyntic glands (HCl secretion) Small Intestine - Duodenum (30 cm) - Jejunum 2.75 m Ileum Large Intestine (Colon) (1.5 to 1.8 m) - Cecum - Colon - Rectum - Anal Canal Mouth - Digestion starts in the mouth with food being broken down through mechanical (chewing) and chemical (enzymatic action) processes. Amylase and lingual lipase are present in saliva; however, food remains in the mouth for a limited amount of time, so little digestion of starch and lipids occurs at this stage. In fact, it is the formation of a lubricated and well-mixed food bolus in the mouth that is important to facilitate swallowing and to set the ideal conditions for a more prolonged and effective exposure of food to gastric juices and enzymes in the stomach and mostly in the small intestine. Stomach - Through the contraction of its antral musculature (longitudinal, circular, and oblique layers) (Fig. 3), the stomach grinds food and turns it into a semi-liquid mass of partially digested food named chyme. The lumen (internal surface) of the stomach contains numerous longitudinal folds (rugae), which distend and flatten as food fills up the space. The distensibility of the stomach allows it to hold a maximum of 4L of food. The cells of the epithelium (inner lining) produce both mucus and an alkaline, bicarbonate containing fluid that protects them from the attack of the stomach’s own acidic digestive juices. Gross anatomy of the stomach. Cardia, fundus, body, and pyloric are the four regions of the stomach. The pylorus is surrounded by a thick ring of smooth muscle that forms the pyloric sphincter. The longitudinal (outer), circular (middle), and oblique (inner) layers of smooth muscle control the flow of chyme towards the pyloric canal. Interspersed with epithelium cells of the stomach are millions of small holes that are openings of the gastric pits or gastric glands. Besides mucus-secreting cells, the gastric pits also contain parietal/oxyntic and chief/zymogenic cells. The former secretes HCl and the latter the pro-enzyme pepsinogen. Secretion of HCl is stimulated by three factors acting at specific receptors on the oxyntic cells: acetylcholine, histamine, and gastrin. Maximal acid production is only achieved when all three signals are present. Acetylcholine is released by the parasympathetic nervous system (vagus nerve). Distension of stomach wall activates stretch receptors that send signals to the brain, which in turn stimulates the vagus nerve and enhances acid secretion. Histamine and the hormone gastrin are released by stomach cells (enteroendocrine cells) as food enters the organ. Histamine acts locally through He-receptors, on nearby cells (paracrine manner). Once released into the bloodstream by enteroendocrine cells found in gastric pits of the pyloric region of the stomach, gastrin acts directly on oxyntic cells to stimulate acid secretion. In between meals or fasting conditions, gastric acid is secreted at about 10 - 15% of its maximal rate, but it drastically increases in the presence of food. Gastric secretion occurs in three phases: Cephalic - Triggered by smell, taste, and/or thought of food and accounts for 30% of acid secretion. Gastric - Triggered by distension of the stomach and by the activation of chemoreceptors by amino acids and peptides. Accounts for 60% of acid secretion. Intestinal - Triggered by distension of the small intestine and by the presence of proteins and products of protein digestion. Accounts for 10% of acid secretion. The stomach secretes gastric juice containing acids [hydrochloric acid (HCI)] and enzymes (e.g. pepsins) that further digest the nutrients in the chyme. The pH of the stomach ranges between 1.5 and 2.5. Proteins entering the stomach exert a buffering effect causing pH to climb to 3. Acidification of the stomach is important because certain digestive enzymes only function under a relatively low pH. For example, pepsin, which breaks down proteins into peptides, is activated in an acidic pH range. Lipases that cleave triglycerides to yield fatty acids also operate at an optimum pH ranging between 4 and 5.5. Conversely, the a-amylase derived from the saliva is inactivated in the highly acid environment, leaving most of the starch to be digested in the small intestine. HCl also destroys many potentially harmful bacteria in the food. Importantly, the stomach is protected from its acids by the production of thick and highly alkaline mucus that resists the action of acids and enzymes. Gastric acids are also important for the absorption of non-heme iron. In fact, in acidic solutions (pH < 4) ferric salts dissolve and can be reduced to ferrous salts, which remain solubilized at the neutral pH found in the duodenum where they are absorbed. Additionally, the stomach produces a mucoprotein named intrinsic factor (IF) that is required for efficient absorption of vitamin B12. The stomach progressively releases small portions of chyme through the pyloric sphincter that opens into the duodenum. This occurs through peristaltic waves. Each of these waves pumps 1 to 3 ml of chyme into the duodenum. Small intestine The small intestine is the longest part of the digestive tube. It is the site where most enzymatic digestion and absorption of nutrients occur. In the small intestine chyme is mixed with pancreatic secretions and bile by segmentation or contractions of circular and longitudinal smooth muscles. Pancreatic enzymes function best in a neutral or under slightly alkaline conditions. Thus, the low pH of the chyme entering the small intestine is quickly neutralized by bicarbonate and H20 secreted by the pancreas and by Brunner's glands located in the mucosa and submucosa of the initial portion of the duodenum. The small intestine is also protected from the acidity of the chyme by a glycoprotein-rich fluid (mucous) that is produced by Goblet cells. Throughout the walls of the small intestine nutrients are absorbed into blood and lymph. At this stage, more than 90% of the ingested carbohydrate, fat, and protein are absorbed and the undigested material is passed on to the large intestine. The small intestine encompasses a huge surface area equivalent to the size of a tennis court. This is possible because the interior surface of the small intestine is folded (wrinkled), which triple the absorptive surface area. Additionally, "finger-like" projections named villi are found lining up on top of such folds, which further enhance absorptive surface area another 10-fold. Each villus is a projection made up of epithelial cells called enterocytes. These cells are highly specialized for digestion and absorption of nutrients. Microvilli located on the surface of enterocytes increase the surface area of the small intestine another 20 times. Microvilli are hair-like extensions of the plasma membrane of the enterocyte that make up the brush border (Figure 4). There are epithelial cells in the crypts of Lieberkuhn located in between the villi that continuously undergo mitosis. The newly arising cells gradually migrate toward the tip of the villi to replace enterocytes that die. In fact, there is a rapid enterocyte turnover approximately every 3 to 5 days. Maintenance of a healthy layer of enterocytes in the small intestine is crucial for digestion and absorption of nutrients, as well as to provide a barrier between the lumen and the internal milieu. Such barrier prevents potentially harmful bacteria from entering the bloodstream or the lymphatic system, which could otherwise lead to serious infections. Cells in the crypt also secrete antimicrobial peptides (defensins) along with large volumes of intestinal juices and electrolytes into the intestinal lumen to facilitate digestion. The microvilli have a surface coat or glycocalyx that together make up the brush border of the enterocytes (Figure 5). The glycocalyx consists of numerous fine actin filaments perpendicularly oriented and attached to the microvilli membrane. An unstirred water layer covers the brush no see of the enterocyte and separates its membrane from the linen of the intestine. This significantly affects the absorption of hydrophobic molecules such as lipids in the small intestine. Structure of the villi in the small intestine and their vascular system. Each villus contains a capillary network and a lymphatic vessel (lacteal) that allows the nutrients that are absorbed by enterocytes to enter the circulatory system. There are small pockets (crypts) in between the villi. Digestive enzymes are glycoproteins that are mostly found embedded in the brush border where they mainly hydrolyze partially digested carbohydrates and proteins. Full digestion of nutrients usually takes place on the brush border, although it may be completed in the cytoplasm of enterocytes. Once absorbed by the enterocytes, nutrients entering the bloodstream (glucose and amino acids) go first to the liver and then are distributed to other organs and tissues throughout the body. Those entering the lymphatic system (e.g. fatty acids and fat-soluble vitamins) bypass the liver and eventually enter the circulation via the thoracic duct. Accessory organs Liver - The liver plays a crucial role in the digestive process because it produces bile, a greenish-yellow alkaline (pH ~7.6 to 86) solution composed essentially of bile acids, salts, cholesterol, phospholipids and pigments (bilirubin and biliverdin, waste products of hemoglobin degradation that confer bile its color). Hepatocytes synthesize bile from cholesterol. The primary bile acids are chenodeoxycholic and cholic acids making up 80% of the body's total bile acids. Once formed, these bile acids conjugate with either the amino acid glycine to produce glycocheno-deoxycholic and glycocholic acids or with taurine to form taurocholic, taurodeoxycholic, and taurochenodeoxycholic acids. The sodium and potassium salts of these conjugated bile acids are called bile salts. The conjugation of bile acids with glycine or taurine improves the ability of bile to form small spherical, cylindrical, or disk-like complexes named micelles during the process of fat digestion. In fact, bile acids and salts act as detergents that emulsify large fat globules by fractionating them into small fat droplets. This is crucial for lipases to access the fat droplets and promote lipid breakdown. Bile is important for lipid digestion; however, it also serves the purpose of eliminating substances that cannot be excreted through the urine. This is the case of organic hydrophobic substances, which are often large (molecular weight >300) and cannot be effectively filtered into the renal tubular system. This is the case of bilirubin, a metabolite of hemoglobin that binds to albumin when released in the bloodstream. The liver removes bilirubin, conjugates it to glucuronic acid, and adds it to bile. The release of bile into the duodenum allows bilirubin to be excreted in the feces. Gallbladder - The gallbladder is a small organ attached to the liver that stores and concentrates bile during inter-digestive periods. The liver secretes as much as 1200 ml of bile every day, but the volume of the gallbladder is around 20 to 60 ml. To deal with such high amounts of bile, the mucosal cells of the gallbladder absorb water, sodium, chloride, and other electrolytes to concentrate bile. Through this process, the gallbladder can produce bile that is concentrated 12- to 20-fold. When needed for fat digestion, the gallbladder releases bile in the small intestine though the hepatopancreatic sphincter. It does so by contracting in response to the hormone cholecystokinin (CCK), which is released by enteroendocrine cells (I-cells) in the duodenum as the acidic chyme enters the small intestine. When bile becomes supersaturated with cholesterol "gallstones" are formed. This is because cholesterol precipitates and provides a crystalline-like structure in which calcium, bilirubin, phospholipids, and other compounds deposit to form a "stone". Gallstones can irritate and cause inflammation in the gallbladder and may also block the flow of bile (choledocholithiasis) into the duodenum or clog the pancreatic duct and cause pancreatitis. Pancreas - The pancreas is an endocrine/exocrine elongated (6 to 9 inches long) organ lying between the stomach and the duodenum. Through its exocrine cells, the pancreas releases pancreatic juice containing bicarbonate, electrolytes, and digestive enzymes. The hormone secretin, released into the bloodstream by S-cells in the mucosa of the proximal small intestine, stimulates exocrine pancreatic secretions. CCK from I-cells, as well as neuropeptide substance P and vasoactive intestinal polypeptide (VIP) present in neurons within the gut, also stimulate pancreatic exocrine secretions. Pancreatic enzymes include trypsinogen, chymotrypsinogen, proelastase, and collagenase (proteases), a-amylase (for CHO digestion), and pancreatic lipase (the main fat-digesting enzyme) and co-lipase. Together, these enzymes digest ~50% of all CHO and protein and 80 to 90% of the fat ingested. Large Intestine - The large intestine is a tube of ~1.5 m and includes the cecum, colon, rectum, and the anal canal (Figure 6). The large intestine lack villi, but it has crypts of Lieberkuhn that abundantly secrete mucus. The primary functions of the large intestine are twofold: 1) to absorb water and electrolytes (taking place mainly in the upper/proximal half), and 2) to store fecal matter (occurring at the distal half) until defecation occurs. The large intestine essentially dries and stores the undigested material present in the chyme. Cellulose and other indigestible substances provide bulk and help maintain regular bowel movement by contributing to the volume of the colonic contents. Figure 6. Gross anatomy of the large intestine encompassing the cecum (blind-end pouch below the junction of the small and large intestines at the ileocecal valve), the appendix (finger-like projection of lymphoid tissue at the bottom of the cecum that houses lymphocytes), the colon that makes up most of the large intestine (ascending colon, transverse colon, and descending colon), the S-shaped sigmoid colon, the rectum, and the anal canal. The large intestine can absorb as much as 5 to 7 liters of fluids and electrolytes per day. The large intestine also houses more than 400 different species of bacteria that can produce absorbable nutrients such as vitamin K (anti hemorrhagic), biotin (co-enzyme for carboxylase reactions), and short-chain fatty acids (eg, acetic, propionic, and butyric acids). The composition of the feces is approximately 30% bacteria, 10 to 20% fat, 10 to 20% inorganic matter, 2 to 3% protein, ad 30% undigested fibers and dried components of digestive juices such as bilirubin and its metabolites. Stercobilin and urobilin are metabolites of bilirubin that provide coloring, whereas bacterial by-products (e.g. indoles, skatole, mercaptans, and hydrogen sulfide) confer the odorous characteristics to the feces. There are trillions of bacteria living in the digestive tract (most of them in the colon), which can be generally classified into two groups: a) those that promote health (probiotics, meaning "for life"), and b) those that can cause harm. It is important to have a balance between the two, ideally a bit more of the former than the latter. Probiotic bacteria are available in some foods (e.g. dairy, soy yogurt, and dietary supplements). Besides promoting a healthy digestive tract and lowering the risk of colon cancer, probiotics have been suggested to reduce cholesterol and blood pressure, as well as support optimal immune function and weight control. There are also food components such as fibers that are not digested in the small intestine but are used as food by bacteria to support their growth and/or activity. Such food components are named "prebiotics". Digestion of Carbohydrates - The term carbohydrate encompasses monosaccharides (e.g. glucose, fructose, galactose, and ribose), disaccharides (e.g. sucrose, lactose and maltose), oligosaccharides (3 to 10 monosaccharide units, e.g. stachyose, raffinose, verbacose, etc.), and polysaccharides (>10 monosaccharide units, e.g. starch, glycogen, fiber, resistant starch, and glycosaminoglycans) (Figure 7). Monosaccharides cannot be reduced in size by hydrolysis and are considered structurally the simplest form of carbohydrate. A hydrolysis reaction occurs during digestion to split a molecule (disaccharide) into two monosaccharides. Water donates H and OH to each of the two separate molecules that result from the hydrolysis reaction to complete the resulting monosaccharides. The most abundant monosaccharide in nature is glucose, a six-carbon sugar represented by the chemical formula C6H1206. Galactose and fructose are two other monosaccharides present in foods that have the same chemical formula as glucose. The former differs from glucose just by the position of a hydroxyl group (OH) on carbon 4, whereas in the latter the arrangement of atoms confers substantial structural differences when compared to glucose (Figure 8). Fructose is the sweetest of the sugars because its structural conformation stimulates the taste buds on the tongue. Also, due to its distinct structural conformation, fructose requires a specific pathway for its metabolism in the liver. Disaccharides are formed by two monosaccharides attached by glycosidic bonds. These are formed through condensation reactions in which a OH group from one monosaccharide and a H atom from another combine to form a molecule of H. Sucrose (glucose + fructose), lactose (glucose + galactose), and maltose (glucose + glucose) are the major energy-supplying nutrients in the diet. Lactose is found naturally only in milk and milk products. Sucrose comes from sugar cane and beet and is the most commonly used natural sweetener. Maltose comes from the partial hydrolysis of starch and is found in malt beverages (beer and malt liquors). The oligosaccharides raffinose (tri-saccharide), stachyose (tetra-saccharide), and verbacose (penta-saccharide) are made up of glucose, galactose, and fructose. They are not hydrolyzed by human digestive enzymes, although bacteria present in the intestine can digest them. Beans, peas, bran, and whole grains contain these oligosaccharides, which are processed by bacteria in the colon often causing flatulence after ingestion of these foods. Polysaccharides or high molecular weight polymers are formed by repeated glycosidic bonding of monosaccharides. The polyglucoses starch and glycogen are the major storage forms of carbohydrate in plant and animal tissues, respectively (Figure 9). Because only limited amounts of glycogen are found in meats and none in plants, food is not considered a significant source of this carbohydrate. Figure 8. Structural characteristics of common monosaccharides. Glucose and galactose differ only by the position of one OH group on carbon 4. Starch is abundant in plants and exists in two forms: amylose and amylopectin. The former is a linear non-branched chain in which the glucose molecules are linked solely through a (1-4) glycosidic bonds. The latter is a branched-chain polymer with branching taking place through a (1-6) bonds. Cereal grains, potatoes, legumes, and other vegetables are rich in both amylose and amylopectin. The contribution of amylopectin and amylose to the total starch content of these foods are 80 to 85% and 15 to 20%, respectively. Amylose - linear a (1-4) glycosidic bonds only Amylopectin Glycogen Human Ni muscles u moderate demonstr a high-c 31(2):20. ingestin known : food in with th Cellul plants. that lit betwe (the c grouf resist make Imp ente mor inv pro gly dis SW tak bi ch a a (1-6) a (1-6) Amylopectin has both a (1-4) and a (1-6) Glycogen is highly branched with many glycosidic bonds more branch points than amylopectin Figure 9. Structure of starches and glycogen. a (1-4) glycosidic bonds confer the linear configuration of the polymer whereas the a (1-6) glycosidic bonds allow the branching to occur. The large number of glucose molecules within glycogen and amylopectin can be cleaved and serve as a readily available source of glucose. Liver and skeletal muscles store glycogen from dietary carbohydrates. Liver breaks down glycogen (glycogenolysis) and releases glucose into the circulation to regulate glycemia under conditions of prolonged fasting. Glycogenolysis is also activated in skeletal muscles under conditions of exercise for energy production. In fact, the ability to sustain prolonged moderate to heavy exercise is dependent on the starting glycogen levels in the muscles. Early studies demonstrated that high exercise intensity can be maintained longer when the same individual consumes a high-carbohydrate diet instead of a normal mixed diet (B. Karlsson and B. Saltin J. Appl. Physiol. 31(2): 203-206,1971). Based on these observations, athletes have adopted the common practice of ingesting large amounts of carbohydrates 24 to 48h prior to long distance races. This is popularly known as "carbo-loading", a dietary strategy used to improve performance in long distance races. The food industry has also made available beverages designed to replace carbohydrates during exercise with the intent of minimizing the impact of endogenous glycogen depletion on endurance performance. Cellulose is also a polysaccharide of glucose, which is found as a major component of cell walls in plants. However, this molecule is resistant to human digestive enzymes because the glycosidic bonds that link the glucose molecules are B (1-4) instead of the a (1-4) found in starch. Briefly, the difference between the a and Bisomers is the orientation of an OH group in relationship to the anomeric carbon (the carbon double-bonded to an oxygen atom). The B configuration occurs when the anomeric OH group is positioned to the left instead of the right (a), which is sufficient to render the molecule resistant to a-amylase, which is stereospecific to a (1-4) glycosidic bonds. Indigestibility of cellulose makes it a fiber, not an energy source. Importantly, for dietary carbohydrate to be used by the cells of the body it must first be absorbed by enterocytes from the gastrointestinal tract into the bloodstream, a process normally restricted to monosaccharides. Therefore, poly-, tri-, and disaccharides must be first hydrolyzed. The enzymes involved in this process are named glycosidases or carbohydrases. As previously mentioned, the process of carbohydrate digestion starts in the mouth by salivary a-amylase hydrolyzing a (1-4) glycosidic bonds. Because food remains in the mouth for a short period, only a few mono and disaccharides are produced at this stage. Even though salivary a-amylase continues to work as food is swallowed; it is inactivated by the low pH of the stomach. Thus, most digestion of polysaccharides takes place later as chyme reaches the small intestine. In the duodenum, the pH is elevated by bicarbonate to a level that optimizes pancreatic a-amylase activity allowing polysaccharides in the chyme to be digested in a stepwise fashion. Because a-amylase (salivary and pancreatic) cannot hydrolyze a (1-6) glycosidic bonds and has very little specificity for a (1-4) links adjacent to branch points, amylopectin is only partially hydrolyzed. This produces large oligosaccharides containing on average about 8 glucose units with one or more a (1-6) links (dextrins or limit dextrins), as well as the disaccharide maltose and the trisaccharide maltotriose. Subsequently, a-limit dextrinase (also known as glucoamylase) sequentially removes a single glucose unit from the ends of linear a (1-4)-glucosyl chains of limit dextrins until isomaltose [a disaccharide with one unit attached by a a (1-6) glycosydic bond] remains. Isomaltose is hydrolyzed by a (1-6)-glucosidase (located in the brush border), the only glucosidase capable of hydrolyzing a (1-6) bonds. Remaining disaccharides are then split by disaccharidases such as lactase, sucrase, maltase, isomaltase, and trehalase that are located within the microvilli. The respective monosaccharide units can then be absorbed by the enterocytes. Lactose has a B (1-4) linkage and requires lactase for its digestion, an enzyme that is stereospecific for this linkage. Infants have high lactase activity, which is significantly reduced after weaning. This can lead to lactose malabsorption and intolerance. Lactase can be added to regular dairy products to minimize the deleterious effects in those that are lactose intolerant. The absorption of monosaccharides is mediated by specific transporters present on the apical side (the intestinal lumen side) and the basolateral membrane (the bloodstream side) of enterocytes. Sodium glucose transporter 1 (SGLT1), glucose transporter 2 (GLUT2), and glucose transporter 5 (GLUTS) are the main monosaccharide transporters in the small intestine. SGLT1 is an active transporter located in the apical membrane. It has two binding sites (one for Na* and another for glucose or galactose). It simultaneous sransports Nat and either glucose or galactose in the same direction, and for that reason it is called a symporter. GLUT2 (a facilitated transporter) moves glucose from the intestinal lumen into enterocytes and glucose, galactose, and fructose from the enterocyte into the bloodstream. GLUTS, also a facilitated transporter, is located on the apical membrane of enterocytes and has high affinity for fructose (Figure Glucose, galactose, and fructose are transported from the intestinal lumen into the enterocyte, and then gain access to the circulation through the capillary network that irrigates the small intestine. The gut vascular bed drains to the portal vein that delivers monosaccharides directly to the liver (Figure 11). Galactose and fructose are ultimately converted to glucose in the liver. The pool of glucose present in the liver after a meal undergoes distinct pathways. A portion of it is stored as glycogen while some is either utilized for energy production or released into the systemic circulation to be used by peripheral tissues (Figure 11). The amount of glycogen stored in skeletal muscles and liver correspond to 1 to 2% and 8 to 10% of tissue weight, respectively. Storage of glycogen in liver and skeletal muscles is mainly driven by insulin. The concentration of glucose in the circulation (glycemia) increases after a meal and stimulates pancreatic cells to secrete insulin. The portal vein receives a bolus of insulin that is drained directly to the liver where it triggers an intracellular signaling cascade that promotes a glycogen synthesis response. The insulin that does not bind to its receptors in the liver continues in the circulation and travels to other peripheral organs. Skeletal muscles and adipose tissue are two peripheral organs that rely on insulin to uptake and metabolize glucose. In fact, after a meal, insulin stimulates skeletal muscles to store glucose in the form of glycogen (glycogenesis), whereas in the adipose tissue insulin promotes the esterification of fatty acids (FA) to form triglycerides (TG, lipogenesis). Figure 10. Transport of monosaccharides from the intestinal lumen into enterocytes, and then into the blood. Brain metabolism - The adult human brain weighs ~1.5 kg and it is estimated that approximately 100 billion neurons and 900 billion glial cells exist in this organ, which confer a high metabolic rate to the brain. The rate of blood supply to the brain is high, about 750 ml/min or 50 ml of blood/min/100g of tissue. This can be compared with about 2-5 ml/min/100g and 50 ml/min/100g of tissue for skeletal muscle under resting and vigorous exercise, respectively. This high rate of blood flow to the brain reflects its high metabolic rate. To maintain such high metabolic rate, the brain oxidizes 120g of glucose per day or ~20% of whole-body energy consumption in a typical day, provided that a diet rich in carbohydrates is common practice. This has been determined by measuring the difference in glucose content between the jugular vein and arterial blood. No significant differences can be detected in brain metabolism during intense mental activities (e.g. arithmetic), likely because the increase in mental activity during such tasks take place in small and specific brain regions that adds very little to overall rate of metabolism. However, such changes in specific brain regions can be detected by specialized techniques that allow scanning of metabolic activity (based on glucose uptake and blood flow changes) when a subject is performing mental tasks. Figure 11. Monosaccharides absorbed by the small intestine enter the liver, which converts galactose and fructose into glucose. Glucose molecules enter the circulation and travel to the brain, adipose tissue, skeletal muscle and pancreas. The latter organ senses elevation in glycemia and releases insulin. This hormone is required for skeletal muscle and adipose tissue to uptake glucose. Adipocytes use glucose to form glycerol-3P (Gly-3P) for fatty acid esterification and storage of TG, whereas skeletal muscles store glucose as glycogen and also use it for energy production. In the liver, insulin is not required for glucose uptake, but it is crucial for glycogen synthesis. Because the blood-brain barrier (BBB) prevents the access of lipid soluble molecules to the brain, the use of fatty acids as metabolic fuel by the organ is extremely limited. Thus, it essentially relies on glucose for its energy supply under normal circumstances, although under prolonged fasting or starvation the brain adapts to use ketones for its energy production. Glucose is for the most part completely oxidized in the brain with just a small portion being released as lactate. Therefore, the brain has relatively high rates of oxygen consumption and COz production. In general, the rate of glucose utilization by the brain is not affected by insulin, although there are particular insulin-sensitive areas where insulin receptors exist. Glucose is transported into nerve cells by the glucose transporter GLUT3, which has characteristics that are suitable for this function. It has a low Km for glucose transport into the cell so that at normal plasma glucose concentrations it is saturated with substrate. Thus, wide variations in blood glucose concentrations cause little change in the rate at which it is taken up by the brain. This also confers a certain protection to the brain against a fall in plasma glucose concentrations. In fact, it is not until glycemia falls below ~2 mmol/l that glucose uptake by the brain decreases and mental function is impaired. GLUTI is also expressed in the brain and it seems to mediate glucose transport at the BBB. Since its Km for glucose is considerably higher than that of GLUT3, it could mean that it would pose a limit to glucose entry to the interstitial fluid at low plasma glucose concentrations. However, it seems that its capacity is sufficiently high not to limit glucose utilization by brain cells. Normal elucase homeostasis - Glucose homeostasis is maintained by e blaind keleback loop that involves the pancreatic iseasels, Giver, and peripheral tissues including the brain, -letal muscle, and adipose tissue. The is is are ile spersed throughout the pancreas and make up 1-2% of pancreatic mass. The islets are composed of 70-80% cells (the ones that produce insulins, 20-25% a-cells (the ones the produce glucago, and -10% of somatostatin and pancreatic peptide threting cells. Insulin and glucagon are to hoones with opposing regulatory effects on glycemia. The pancreatic islet is a well-vascularized structe, so as the insulin synthesized by cells enters the islet vasculature it is sensed by a-ells to regulate lucagon secretion. As blood glucose levels rise after a meal, Bells increase in suicis to ron. This leads to a reduction in glucagon secretion by the c-cells. Conversely, fasting and prolonged exercise), insulin secretion is when blood glucose levels are reduced (e.g: reduced, a condition that leads to an increase in glucagon secretion. After being secreted, insulin and glucagon flow into the portal circulation, so the liver is exposed to these hormones to concentrations at are S- to 1-fold hisher than peripheral tissues, (e.g, skeletal muscle, adipose tissue, and brain). This allows the liver to tightly control its rate of glucose production. In peripheral tissues (skeletal muse and adipose tissue), glucose uptake, either mediated by insulin or not, is modulated in part by the spregulation and down regulation of glucose transport (GLUT) proteins in target cell membranes. In the basal state (after an overnight fast), hepatic glucose production (via glycogenolysis and gluconeogenesis) and renal production (via gluconeogenesis) are responsible for supplying the basal! lucose requirement of peripheral tissues. Approximately 75-80% of glucose utilization in the fasting state occurs in cells of insulin-independent tissues (brain and gut), as well as in red blood cells. Tissues such as skeletal muscle and adipose tissue that are largely dependent on insulin to uptake significant amounts of glucose have minimal requirement of this substrate under fasting conditions. In the fed state, an elevation in circulating glucose causes an increase in insulin secretion by the B cells, which then stimulates glucose uptake in insulin-dependent tissues (Skeletal muscle and adipose tissue), while concomitantly inhibiting hepatic and renal glucose production. This inhibition is essentially because insulin suppresses glucagon release by a-pancreatic cells and signals the liver to store glucose as glycogen. Contrary to the fasting state, glucose utilization in the fed state is dominated by skeletal muscle and adipose tissues accounting for 85% and 5% of the insulin-stimulated glucose uptake, respectively. The rise in isulinemia also results in decreased plasma non-esterified fatty acids (NEFAs) because of potent inhibition of adipose tissue lipolysis by insulin. Lower availability of plasma NEFAs also contributes to increase the utilization of glucose by skeletal muscles. Physiologic insulin secretion is also modulated by non-glucose nutrients, hormones, and neural influences. Amino acids, specially leucine, arginine, and lysine, stimulate insulin secretion in the absence of glucose and augment insulin secretion in its presence. Glucose absorption in the GI tract stimulates the release of gastrointestinal peptide hormones, known as incretins, including glucagon-like peptide-1 (GLP-1) and glucose-dependent insulinotropic polypeptide (GIP), and CCK. Incretins do not independently stimulate insulin secretion, but augment f-cell response to the glucose load in a glucose concentration-dependent manner. At equivalent plasma glucose concentrations, insulin the incretin effect. secretion after an oral glucose load is higher than with an intravenous glucose load, which illustrates Neural influences on pancreatic islet cells are mediated via cholinergic and adrenergic innervation. Insulin secretion is increased with parasympathetic or vagal stimulation and suppressed by sympathetic activation. However, both sympathetic and parasympathetic stimulation have been associated with increased glucagon secretion. This helps explain why under prolonged exercising conditions insulinemia is reduced, whereas circulating glucagon levels are elevated. Dietary sources of sugars - Sugar sweetened beverages are the main source of dietary sugar in North America. In fact, according to the National Health and Nutrition Examination Survey (NHANEShad assesses the health and nutritional status of adults and children in the United States, sugar sweetened beverages (soft drinks, fruit drinks, and coffee and tea) along with snacks and sweets account for about 70% of all added sugars in the diet. These are sources of simple sugars and normally referred to as "empty calories" because they are poor in other crucial nutrients required for normal physiological function. Fruits, vegetables, and grains combined account for only about 10% of all the added sugars consumed (Figure 12). Importantly, simple sugars can quickly increase circulating levels of glucose and lead to a spike in insulin release. The latter greatly favors the storage of fat in the adipose tissue. There has been great interest in limiting the intake of added dietary sugars, particularly from soft drinks and fruit juices, in an attempt to reduce obesity and its comorbidities (insulin resistance and type 2 diabetes) in the population. Two recent meta-analyses (published between 2010 and 2014) have concluded that a moderate to strict low carbohydrate diet is highly effective for achieving weight loss and improving most heart disease risk factors in the short term (six months) (Obes Rev 2012;13:1048-66 and JAMA 2014;312:923-33). Also, another meta-analysis found that if carbohydrates are kept very low, weight loss is greater than with a low-fat diet maintained for a year (Br J Nutr 2013;110:1178- 87). These findings have led public health authorities to shift the focus from fat towards excessive sugar consumption as being the main culprit for obesity and its comorbidities. The 2015 US Dietary Guidelines for Americans states that added sugars should be reduced in the diet and not replaced with low-calorie sweeteners, but rather with healthy options, such as water in place of sugar-sweetened beverages. The Dietary Guidelines Advisory Committee puts a cap on sugar consumption to less than 10 percent of the total daily energy intake. These should come preferentially from fruits (especially whole fruits) and grains (at least half of which are whole grains). The idea of capping the consumption of added sugars has also taken other approaches such as the additional taxation of soft drinks ("soda tax"). This has been implemented in some countries in Europe (France, Hungary, UK) and in North America (Mexico and United States). Whether this approach will lead to a significant reduction in obesity rates is certainly debatable. According to the National Obesity Forum (NOF) from the United Kingdom "the roots of obesity and type 2 diabetes are firmly embedded in the food environment". In its latest publication, the NOF-UK recommends people to eat fat, cut the carbs, and avoid snacking to reverse obesity and type 2 Diabetes (www.NationalObesityForum.org.uk). It also emphasizes that added sugar has no nutritional value whatsoever, and that excess sugar consumption is strongly associated with increasing risk of type 2 diabetes, hypertension, and cardiovascular disease, independent of its calories or its effects on body weight [Can J Diabetes 2016;40(4):282-6; Obesity Silver Spring 2016;24(2):453-60; JAMA Intern Med. 2014;174(4):516-24). The NOF also states that "the optimum sugar consumption for health is ZERO" and it recommends that food labelling on added sugar should be recorded as number of teaspoons. This enables consumers to make more informed decisions when purchasing products in the supermarket. The concept of glycemic index - The glycemic index GI) represents the degree and duration that a specific food increases glycemia. It is determined by calculating the area under the curve for blood carbohydrate. The Gl of a food is a direct comparison of its glycemic response to that of a standard food (usually 50 g of glucose or a slice of white bread containing 50 g of carbohydrate). For instance, if a food raises glycemia to a level that corresponds to 70% of the value found with glucose, ther the GI of that food is 70. Because the amount of food needed to determine the GI is not the amount of food typically ingested by people, the utilization of the GI by regular people has proven to be challenging. To facilitate things, the glycemic load (GL) has been created. Basically, it is the GI normalized to serving standards. The GL is calculated by multiplying the GI by the amount of digestible carbohydrate present in a food and then dividing it by 100. The GI and GL can be very different. This is because a food can have a high GI, but if ingested in a small portion produces a low GL. Carrots are commonly used to exemplify the discrepancy that can be found between GI and GL. Carrots have a relatively high GI (90); however, only 4g of digestible carbohydrate is found in a serving of 1 cup (90 x 4 ÷ 100 = 3.6). This means that a food can have a high GI and a low GL; therefore, its impact on glycemia will depend on how much of it is consumed on a regular basis. GI and GL levels can be classified as low, medium or high as shown in the Table 2. Table 2. Classification of glycemic index (GI) and respective glycemic load (GL) Level GI GL GL/day Low 55 or less 10 or less Less than 80 Medium 56 - 69 11-12 80 - 100 High 70 or more 20 or more More than 120 Ingestion of foods with high GI and GL has been associated with the development of chronic diseases (Type 2 diabetes and coronary heart disease) and obesity. However, the data linking the regular ingestion of high GI/GL foods with metabolic disorders have been challenged, since they derive from epidemiological studies in which self-reported questionnaires were used. Despite the controversy, the use of Gl and GL in dietary planning can be helpful in the prevention of obesity, as well as in the management of diabetes. The Gut Microbiota An enormous population of bacteria (~39 trillion bacterial cells) live in and on the human body along with a substantial number of viruses (eukaryotes and prokaryotes), archaea (microorganisms that resemble bacteria but are different from them in their genetic makeup and certain aspects of their cell structure, and fungi (spore-producing organisms such as molds, yeast, and mushrooms that feed on organic matter. The assemblage of microbes living in a habitat is now referred to as microbiota. The microbes in and on the average adult human body comprise 1%-3% of body mass, with the bacteria vastly outnumbering the other kinds of microorganisms. The ratio of bacterial cells to human cells is estimated to be around 1.3 to 1 (Sender R. et al. PLoS Biol. 2016, 19;14(8):e1002533). Of all sites in and on the body, the digestive tract or gut houses the most dense, diverse and dynamic collection of microorganisms. Within the gut environment (particularly the colon), microorganisms interact with nervous, immune, and endocrine systems. The majority (Over 90%) of bacteria present in the adult human intestinal tract are members of the phyla Bacteriodetes and Firmicutes, with the remaining being members of Proteobacteria, Actinobacteria, Fusobacteria, Verrucomicrobia, and Cyanobacteria. The colon is the site of breakdown of materials that escape digestion early on in the digestive tract, namely dietary fiber, resistant starches, and non-carbohydrate substrates. Colonic bacteria produce enzymes that ferment these materials. Among the waste products of this process is an important group of metabolites called short-chain fatty acids (SCFAs, acetate, propionate, and butyrate). Some of these SCFAs are excreted, but the majority (95%) are used by colonic cells as a source of energy and provide ~10% of the total daily calories for the host. SCFAs also serve as important messengers between the microbiota and the immune system, playing a role in the development and function of intestinal epithelial cells and leukocytes. Bacteria in the colon also produce B vitamins (thiamine, riboflavin, niacin, biotin, pantothenic acid, and folate) and vitamin K. Vitamins originating from the diet are absorbed in the small intestine, whereas microbe-produced vitamins are absorbed in the colon. Figure 13. GI of various foods commonly ingested compared to white bread (GI 100). An extensive list of GI for various foods can be found in K. Foster-Powell et al. Am J Clin Nutr 2002, 76:5-56. Dietary Fiber - There has been a lot of debate regarding what comprises dietary fiber. In this context, two definitions can be found: one for dietary fiber and another for functional fiber. The former referring to non-digestible carbohydrates and lignin that are intrinsic and intact in plants, whereas the latter consists of isolated, nondigestible carbohydrates, extracted, or manufactured that have beneficial effects in humans. The sum of dietary and functional fibers encompasses the total amount of fiber consumed by an individual. Fiber can also be classified in either soluble or insoluble groups, based on its propensity to dissolve in H2O. Pectin (polysaccharide present in plant cell walls), gums (polysaccharide composed of the galactose and mannose), and mucilages (polar glycoprotein/exopolysaccharide from plants that forms a gluey substance) are examples of soluble fibers, whereas cellulose (linear polysaccharide found within plant cell walls), hemicellulose (a branched polysaccharide also present in plant cell walls), lignin (highly branched hydrophobic and aromatic polymer that fills the spaces in the cell wall between cellulose, hemicellulose, and pectin), and modified cellulose are members of the non-soluble group. Fruits, legumes, oats, and some vegetables are good sources of soluble fibers. Cereals, grains, nuts, seeds, corn bran, and some vegetables and fruits provide insoluble fibers. Dietary fibers regulate in several ways the digestive process as food travels through the digestive system. The presence of fiber in the food causes several effects: Forms a viscous gel-like mass that delays the rate of stomach emptying of ingested foods into the duodenum Reduces the mixing of gastrointestinal contents with digestive enzymes Reduces digestive enzyme function Reduces nutrient diffusion and attenuates the glycemic response to food Alters small intestine transit time Diminishes absorption of lipids Increases bile acid excretion Lowers cholesterolemia Besides is efcts in nutrient digestion and absorption, fibers (essentially entatouble ones) are also Feries iby health-promoting bacteria residing in the colon. Through fe mentation of fibers, bacteria cembain energy and other substances (eg. nitrogen that prominicateronah. Additionally, fem ebain produos short-chain faty acids (acetic, butyric, and propionic acids) that can be used by the human host. This occurs mostly in the cecum and in the ascending portion of the colon. These short-chain fatty acids exert the following effects in the GI tract: Stimulate H20 and Na* absorption into the mucosal cells of the colon Promote differentiation and proliferation of mucosal cells of the colon Lower pH that reduce bile solubility and increase Ca* binding to bile and fatty acids. Such effects may exert a protective effect against colon cancer Provide energy for colonic cells Inhibit hepatic cholesterol synthesis Enhance colonic blood flow Enhance immune function Prevent the growth of potentially harmful cells The insoluble fibers that are poorly or non-fermentable (mainly cellulose and lignin) also play an coring chi, honing role id beli to aese by ingreasing Peal volume and encericing with the colonic mucosa and facilitating their excretion in the feces. Recommendations for carbohydrate and fiber intake - The recommendation for carbohydrate intake in Canada and the USA is 45% to 65% of total energy intake. Most of the carbohydrate ingested should derive from nutrient-rich sources such as whole-grain products, legumes, low-fat dairy products, fruits, and vegetables. There have been some concerns with regards to the content of added sugars in foods. The recommended daily intake (RDI) suggests that added sugars should not account for more than 25% of the total daily energy intake. The World Health Organization (WHO) and the Food and Agriculture Organization (FAO) have been much stricter, proposing that the intake of added sugars should be capped to 10% or even 5% of total energy intake. Health Canada set the daily reference value for fiber at 25 g for a 2000 kcal energy intake. The RDI for fiber intake is slightly higher, set at 38g and 25 g/day for young men and women, respectively. The WHO provides a more generic recommendation of 25g to 40g of fibre daily for adults. These recommendations can be met by ingesting daily whole grain products (1 to 2 g of fiber per serving), vegetables ( 2 to 3 g of fiber/serving), fruits (about 2 g of fiber/serving), and legumes (6 to 8 g of fiber/serving)