Lecture Notes on Natural Selection and Genetic Linkage PDF
Document Details
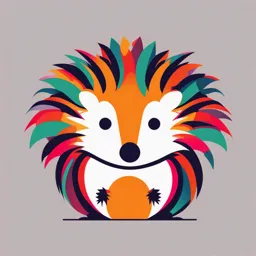
Uploaded by SpectacularInequality
Tags
Summary
This lecture delves into natural selection, using examples like Galapagos finches and stickleback fish to illustrate the process. It also explores the concept of genetic linkage and recombination, highlighting how they contribute to evolutionary processes. The lecture emphasizes the importance of understanding these concepts within the broader context of evolutionary biology.
Full Transcript
Welcome. With this lecture, I’d like to add a little perspective to some of the examples detailed in Chapter 8, which discusses a large number of well‐documented cases of natural selection operating in natural systems....
Welcome. With this lecture, I’d like to add a little perspective to some of the examples detailed in Chapter 8, which discusses a large number of well‐documented cases of natural selection operating in natural systems. 1 One of the most thoroughly documented examples is the famous work by Peter and Rosemary Grant, who were inspired to revisit the radiation of ground finches on the Galapagos. Where Darwin saw great phenotypic diversity among closely related birds, the Grants set out to systematically test each factor required to establish the operation of NS. The great thing about the Galapagos is that as a remote volcanic archipelago, it is ecologically simple. The Grants’ work focuses primarily on documenting change through time in response to changing fitness. Beak size influences fitness and is heritable Natural selection can cause change Long‐term studies reveal fluctuation in the direction and strength of natural selection 2 One species that they’ve focused on is the Medium ground finch 3 In this species, on Daphne Major, they documented that beak size was heritable, fufilling one necessary requirement for NS to operate. By banding birds and carefully observing them in the field, they were able to establish that individuals differed systematically in their foraging behavior according to the size of their bills, with larger beaked birds having an easier time breaking open the hard seeds of one of the most abundant plants on the island, the caltrop. This species is very drought‐tolerant, and persists when other plants are die from lack of water. Most years, another species, the spurge, is abundant enough to feed even those finches that were unable to open the caltrops. But the Grants observed this population during a drought year, and were able to document the death of most of the smaller billed finches in the population when spurge failed to produce seed. The survivors that bred the following year, were not a representative sample of the pre‐drought finch population, but rather consisted primarily of large‐beaked individuals. The high amount of selection on a strongly heritable trait led to a very strong response in subsequent generations that could be seen in Drought results in more hard, woody seeds, which favor larger beaked birds 4 Generations that followed for several years as shown by these figures that show enlarged beaks persisting for 10 years after the drought. But why did the population return to the prior, pre‐drought mean? When a wet year happened a few years after the drought, spurge bloomed profusely, covering the island in seeds that the smaller‐beaked individuals were more nimble at finding. By the early 80’s small‐beaked individuals were reproducing at a faster rate than their large‐beaked conspecifics. This goes to show that NS operates without foresight, and traits that are good at one time may in fact be less good at other times. The beauty of the process is that it continuously adapts and shapes populations, but in unpredictable environments, populations will be continuously fluctuating back and forth as we see here. 5 Selection can work in different directions in different areas, too. Kingsnakes that mimic venomous coral snakes are rarely attacked within the range of coral snakes (because predators in this region learn to avoid the painful bites of coral snakes) but outside the range of coral snakes, less conspicuous coloration is is favored by selection, and predominates, because predators are not “educated” to refrain from attacking coralsnakes leaving non‐venomous kingsnakes unprotected. Aposematism (or warning coloration) is favored only in areas where coral snakes co‐occur. On the other hand, researchers were able to document that Gene flow can bring alleles causing mimic coloration to new locations outside the range of coral snakes, where the unfortunate bearers of these face greater risk of attack, leading to a cline in coloration toward redder individuals. Thus, within the range of this species, certain alleles may increase or decrease fitness, and natural selection can lead variation over the geographic range of a species 6 We already saw an example of this in the previous module, where Hopi Hoekstra showed a strong difference in predation among white and brown “mice”, but that the direction of the predation depended on the background of the substrate her models were placed on. This experiment was designed to show the selective forces at work in driving the changes in coat color in Peromyscus. 7 In this case the story was complicated by the fact that there were several populations that had independently colonized white sand barrier islands. Each time this happened (as shown by the phylogenetic analysis) a shift from brown to white cryptic coloration was still favored, but the specific mutations that enabled this phenotype to be expressed turned out to be unique in each case. This illustrates a few important points: NS often leads to convergent phenotypes. Only sometimes, however, will the genetic basis of these phenotypes be the same. This is because each time the transition between habitat types is made, NS only can work with the genetic variation present in the population that is adapting. Without heritable genetic variation, no evolution can happen, no matter how strong selection is. The other thing is that a phylogeny was critical to this study. Without a phylogenetic tree (built using independent molecular data that is unrelated to the coat color trait), Hoekstra and her team would not have had much indication that white coat color was independently evolved more than once! 8 Repeated evolution of particular traits is often the best evidence we have for the action of NS in wild populations. The text goes into great detail about the evolution that has been observed over and over in isolated populations of stickleback fish, that tend to lose their metabolically costly dorsal spines and plates of body armor when isolated in freshwater lakes that have few predator species. 9 The text describes numerous analyses done by Dolph Schluter and others demonstrating this trend in lake after lake, including analyses of fossil deposits that record, for instance, an influx of large‐spined individuals associated with a marine connection, followed by a loss of protective spines once this lake was re‐isolated. 10 The genetic basis for the armor plate trait has been determined, being cotrolled by a gene known as Low Eda allele favored in freshwater populations. Because the production of armor is so costly to these fish, if predators are not found, Low Eda (which is a recessive allele that exists in most populations at low frequency) is favored. This graph shows the result of an inadvertant experiment that occurred when fisheries managers killed all fish in a lake in order to prepare for the introduction of gamefish. Once sticklebacks recolonized the lake, the low‐eda allele rapidly increased to a very high frequency. Populations that independently experience parallel environmental changes represent replicated natural experiments, which enable biologists to conduct evolutionary studies. One occurrence is interesting, but multiple examples of the same phenomenon happening provide strong evidence for the action of natural selection operating in predictable ways. 11 There’s a replicated example of NS in our own species. Populations of people have domesticated cattle and begun the use dairy products in Europe, Africa, and India. 12 Lactase persistence (defined as the ability to digest lactose in adulthood) is a derived trait possessed by only 30% of humans, but it is concentrated in people originating in northern Europe, Africa, and Arabia and India, reflecting the long history of dairy consumption in these regions. Non-dairy cultures, particulary in Asia, have a difficult time with lactose and have gastrointestinal difficulties when dairy is consumed. That’s why there’s no cheese in Chinese cuisine! 13 Please, if you have questions, post them to the discussion board. 14 Welcome. With this lecture, I want to delve a bit deeper into the concept of genetic linkage. We’ve seen a little of this already, when we talked about QTLs, but there are many important evolutionary concepts that depend on understanding this concept, so it’s worth taking the time to make sense of it. 1 TALK THRU THIS SLIDE… In fact, proportion of gametes with each allele is the product of the frequencies of those alleles: 0.5* 0.5 = 0.25. Keep that number in mind. 2 Now, remember back to the process of Meiosis that you’ve learned in Intro Bio. Before Meiosis occurs, each chromosome will replicate, then, during Meiosis I chromosomes line up next to their homologs. 3 Still during Prophase I of meiosis, crossing over occurs between homologous chromosomes. This is important. The location of crossing over events (or chiasmata) is somewhat random. (It’s not entirely random, crossing over is less frequent close to centromeres, for example) 4 And, as you can see, multiple chiasmata can form along the length of the chromosomes. In real life, there would be several pieces exchanged from each chromosome with its homolog, but for the sake of simplicity, let’s consider a single crossing over event. 5 Once this is Prophase I complete, segments of each chromosome will have been exchanged. If you examine closely, you see the result of a crossing over event is that two of the chromosomes can be considered recombinant. 6 At the end of meiosis II, each chromosome separates into an individual gamete. What this has done is create 4 unique chromosomes among the gametes. 7 Let’s return to our two loci, B and D, and say, now, that we know that they lay on the same chromosome, although some distance apart, one near the end and the other near the centromere. A recombination event that falls between B and D produces gametes in the expected proportion, one BD, one Bd, and so on. Of course there could be several recombination events between B and D, and within an organism, lots of gametes will be produced, so the overall effect of this is to make it so that alleles at B and D are inherited independently, as though they were on separate chromosomes. 8 Let’s add a third locus to the mix‐ A. A is heterozygous, too, and it lies really close to B on the chromosome. So close, in fact, that random recombination events only rarely happen in the segment of chromosome between A and B. Let’s look at the genotypes of the gametes that result: ABD, ABd,, abD, and abd. Now ask, what proportion of gametes are AB? Remember, we’d expect them to be 25%, because as a heterozygote, A is 0.5, B is 0.5, therefore the product is 0.25. Instead, we see that AB is found at 50%. what about ab? 50%. Are there any Ab or aB? No. Because they lie so close together on the chromosome, they behave as a single locus, and you’re twice as likely to find A and B together as you would expect if you were estimating based on allele frequencies alone. This deviation from expected genotype frequencies is called linkage disequilbrium. 9 10 You may recall that in the previous module we discussed QTL analysis. This form of genetic mapmaking takes advantage of linkage to look for pairs of loci that are in high LD with each other, and then these data are used to infer the ordering of loci along chromosomes (or linkage groups). If many markers are used, then it is possible to “saturate” the genome with informative marker loci, for which some alleles will covary with those in traits of interest, allowing the location of trait‐influencing alleles to be identified. 11 If LD is calculated between sites along the length of a mapped chromosome, clues can be obtained about sites under selection. For example, if the gene in the middle of this chromosome is subject to strong selection, then LD will be estimated to be high near that site (Blue Line). Since those variants that are near the selected site on the same chromosome are linked, they will tend to increase in the population like the selected allele, meaning that other alternative alleles will decrease in frequency, and overall genetic diversity (red line) will drop near a selected site. The farther you go from the selected site, the more likely it is that recombination will destroy the linkage between the selected alleles and other sites. 12 A related method relies on the fact that strong selection on an allele (say a beneficial “star” mutation) will tend to bring along nearby variants to high frequencies. This reduces variation around the selected locus. In the case of positive selection for an advantageous allele, we call this a selective sweep. A similar effect is found when rare deleterious alleles that are selected out of a population may remove nearby variation too. This is called “background selection. 13 Recall from the previous lecture that two different mutations are known that confer the trait of lactase persistence on human populations that consume dairy in adulthood. Surrounding each of these mutations in their respective populations there is a characteristic pattern of a loss of genetic diversity in sites within 1‐1.5 mB, indicating that these regions of the chromosome are under strong positive selection. 14 Please, if you have questions, post them to the discussion board. 15 Welcome back. With this lecture I want to emphasize a couple of prominent ways in which our species is affecting the evolution of many species we interact with. 1 Perhaps the most famous example is the production of domestic crops. When was the last time you saw a wild Cauliflower? In fact, many of the common vegetables we enjoy (or don’t enjoy?) are all derived from a single speces of wild mustard. Brassica oleracea has been bred into all of the vegetables shown here by selecting individuals that contained mutations in flowering branch architechture, root size, or or bud or leaf expansion. 2 In fact, every single one of our crop species has undergone substantial genetic modification. The changes wrought on wild species that have been favored by us for food have, compared to their wild progenitors: Rapid growth, Large fruits Lesser amounts of bitter/toxic compounds Synchronization of ripening Retention of fruit on plant for easier harvest Annual vs. perennial habit 3 For example, over the last 10000 years has transformed a wild grass known as Teosinte into modern Corn or Maize. Teosinte has few hard grains that easily shatter from the stalk. By comparison, Maize has many more kernels that are retained on the cob, and sweet corn has a much higher sugar content and softer shells on each kernel. Many cobs of maize have been recovered from archaeological sites in Mexico that show rapid enlargement of the cob over only a short time period! Interestingly, one early way that corn was consumed was as popcorn! 1100 years of selection produced a large change in cob size 4 Domesication happened to animals, as well. Traits such as docility, diversity of coat color, changes to ear and tail shape, smaller brains, and extended breeding seasons are all noted among domesticated animals. Dogs have been bred from their wolf-like ancestors over 15000 years of association with humans, with much of the diversity in breeds that we’d recognize today being only a few hundred years old at most. Dogs actually retain for their entire lives a lot of juvenile behaviors- behaviors that wolves and coyotes grow out of- like barking- and their bodies resemble grown up puppies. Some research shows that they have unique abilities to relate to our species- that wild progenitors completely lack. 5 By some estimates, our species affects 83 percent of the Earth’s land surface, and 12 percent of the land is directly used for growing crops like wheat, corn, and rice- representing 1/3 of the Earth’s terrestrial biomass. This enormous resource is irresistible for numerous pest species, and the use of pesticides and herbicides has become an integral part of modern agriculture. 6 Weedkillers have several modes of action, targeting different physiological pathways. Many weeds have been found that are able to withstand multiple different classes of herbicides, rendering them increasingly able to flourish despite farmers’ efforts to counteract the evolution of resistance by using multiple herbicides on each field- with the idea that if one herbicide doesn’t work, another will. 7 In the 1990s Monsanto corporation developed several transgenic crops that had a gene that provided resistance to the herbicide glyphosate, also known as roundup. This herbicide is much less toxic than most others, and so, farmers who planted “roundup ready” crops could apply the herbicide in massive doses multiple times per year, and not damage their crops while keeping weeds out of the fields. This meant workers and consumers would not be exposed to more toxic chemicals. While GM crops like this were erroneously perceived as dangerous by the general public, in fact, evolutionary biologists predicted that over time, weeds would emerge that would be resistant to this herbicide. In fact, at least three weeds have been found invading fields that tolerate roundup. Two of these species have independently altered the enzyme, EPSPS, that roundup attacks, while the third, Palmer Amaranth, duplicated the locus in its genome several times, and thus produces enough of the essential enzyme that roundup isn’t effective. Multiple ways to arrive at the same outcome. 8 Other undesireable species that live in close association with humans have evolved pesticide resistence as well. The common housefly has continuously adapted to each new insecticide we have developed, with resistant individuals originating within a few years of the introduction of new chemicals, and full resistance developing within a few more. 9 The other main creation of the biotech crop industry was the engineering of crops to produce a bacterial toxin that kills certain insects, known as Bt (for Bacillus thuringensis) This toxin doesn’t affect vertebrates, but is lethal to caterpillars and moths, especially, including the corn rootworm moth. As the emergence of resistance to the toxin was seen to be inevitable, strategies were devised to prolong the amount time before the technology becomes useless. This diagram shows the reasoning behind the strategy of planting “refuges” of susceptible crops near fields of Bt crops. If many pests are bred on unaltered plants, then those few individuals that survive in the Bt field (and are resistent) breed with the susceptible moths, instead of breeding with other resistant individuals. Heterozygotes that result from these crosses, however, are still susceptible to the Bt toxin. In effect, a beneficial recessive allele (which could be selected to fixation, theoretically) is kept at low frequency. Bt crops select for resistance in pests but resistance has a fitness cost. Creation of Bt-free refuges favors Bt-susceptible insects Refuges are now required by law 10 The importance of this strategy is now recognized by laws that REQUIRE the planting of non-BT refuge areas within or adjacent to fields of Bt crops. In fact, seed companies are now simply blending a small percentage of non-Bt seed into each bag they sell, to meet these requirements and maintain the usefulness of Bt as pest mangement tool. Knowledge of Evolutionary biology, and the management of natural selection, is being put to good use. 11 Note that this chapter discusses several other cases where human-altered selection pressure has produced changes in natural populations. For example, by hunting and fishing wild animals, we have altered aspects of behavior, morphology, and life history. We truly are one of the most powerful selective forces on the planet! Whether we’re talking about pest management, management of infectious disease, harvesting wild populations, or developing desirable features in domestic species- actually, in nearly any case where we are intentionally or unintentionally interacting with populations of organisms- an understanding of evolutionary biology is essential to intelligently managing outcomes! Characterizing variation, and understanding selection can often lead to predictable outcomes. 12 …even if the details are left up to nature, as they put it in Jurassic Park- Life finds a way! Actually, the Ian Malcomb character could have been even more pithy by specifying the following: 13 14 Please, if you have questions, post them to the discussion board. 15 Practical review of modern phylogenetic methods. 1 We’ve already seen that phylogeneticists use molecular data‐ but how exactly is it done? To begin with, let’s get a few basics out of the way: GO THROUGH SLIDE CLICK 2 There are more optimality criteria than we’ve alluded to before‐ besides Parsimony GO THROUGH SLIDE 3 Just to refresh your mind about how parsimony handles character data, consider the simplist non‐trivial tree you could build‐ four taxa with one character!, and no outgroup! GO THROUGH SLIDE 4 Here it’s easy to see that the simplest way to reconstruct this char is a single change here. GO THROUGH SLIDE 5 The CI can be as high as 1.0, if all the characters can be added to the same tree with no homoplasy. (this is not easy to achieve with molecular data) 6 See for example this familiar tree‐ by requiring 6 steps to explain changes in 5 characters, the CI is only 5/6ths 7 GO THROUGH SLIDE 8 Weakness of Parsimony LBA With lots of substitutions, parallel subs will occur and tend to bring branches into more similarity as they get longer‐ attracting more. Bad feature. 9 One of the problems with many methods is the need to calculate tree scores and compare across trees. Distance methods in their simplest form don’t requrie this. Of course, you could count the percentage of base pairs that differ between species and put into a pairwise matrix. 10 Or be more sophisticated, doing other distance methods that account for known prob. of different mutations occuring, to try to be more accurate in measuring how distinct seqs are. 11 With a distance matrix, you can start adding the most similar taxa together, reconstructing an artificial node that is closer to other species, and repeating until all taxa are added. FINISH WITH THIS SLIDE 12 ML= distance but likelihood is treated as a tree score‐ that is prob. Of observing the data matrix you have, given a particular tree and the model. Then, like pars. trees compared to find one with maximal likelihood. 13 GO THROUGH SLIDE 14 Once you’ve decided on an optimality criterion, you need to use it to decide among the zillions of possible trees, which is impossible. So how do you increase the odds of finding good trees. Distance NJ is often used to get an intial tree prior to branch‐swapping in more formal searches. Then, swapping GO THROUGH SLIDE 15 You might wonder, after all this, whether your tree is good enough? EXPLAIN PSEUDOREPLICATES 16 THEN EXPLAIN HOW ALL replicates are reanalysed to determine how many trees have each branch. 17 Bayes‐ READ SLIDE & GO THROUGH 18 EXPLAIN THRESHOLDS 19 Phylogenetic trees are hypotheses that are constantly reevaluated when new data & methods become available Statistical models help scientists sift through molecular evidence to determine the best hypothesis or hypotheses that explain the data 20 Please, if you have questions, post them to the discussion board! 21 One of the biggest breakthroughs in modern evolutionary biology in recent years has been the unification of population genetics with phylogenetic systematics. This has led to a lot of progress in understanding the biology behind phylogenetic trees, from historical demography (the movements and changing sizes of populations through time) to phylogeography (the study of population structure produced by geographic factors) and many other applications. 1 The essence of coalescent theory is that it starts from a sample of alleles or gene copies at the present time, and works backward in time. In coalescent‐speak, the term allele is used pretty interchangably with gene copy‐ and it simply refers to a copy of a segment of DNA on a single chromosome. Consider A‐ this represents the gene copies present in a population of five diploid individuals, each with two copies. So, this population has 10 copies, or alleles. This is a little different than our previous use of allele‐ all of these copies might be identical in state, or they might carry mutations that make them different. It’s not important right now. Look at B. In this case, the alleles are considered separately of the individual they are found in. There’s no need to keep the diploid individuals’ gene copies together, we’re just interested in the history of the gene copies themselves. Now look at C. In this figure, alleles at each generation have simply been re‐ordered to eliminate crossing lines. Some of these gene copies will be found in successfully reproducing gametes, and others won’t. If there’s no reproduction, then that allele goes extinct. Other alleles leave multiple copies of themselves. This is the process of genetic drift‐ allele frequencies changing through time. When an allele reproduces itself, then its descendents are considered identical by descent, meaning they have a gene copy that came from a particular ancestor. If you start with the oldest generation, you’ll see that only 6/10 alleles make it into the subsequent generation. Of these, two more fail to leave descendents in the third 2 generation. I think you see where this is going… Now if, rather than looking forward in time trying to keep track of each gene copy at each generation, we turn the problem on it’s head, we can track alleles at the present day back in time. 2 If you take a bunch of gene copies from a population, say 1, 2, and 3, then there exists a history for those copies in which some of those copies will have been found in a common ancestral gene copy. In this case, it is a single generation back in time for 2 and 3, and for 1, 2, and 3 it is 5 generations back in time. It’s generally true, that as you start to sample more gene copies in a population as we’ve done in B, the number of generations back you must go increases, because each new copy you add might descend from an ancestor you’ve already identified, or it might descend from an earlier ancestor. Look at A and B. There are different common ancestors identified, a different number of generations back, depending on which gene copies were sampled! In the case of A, it was 5 generations back that this last common ancestor lived, whereas if more copies are included, it’s 8 generations back to the most recent common ancestor. These ancestors are individuals in which alleles are said to coalesce. Multiple lineages become one, looking backward in time. There are probably many copies of that allele that pre‐existed the most recent common ancestor, so they are ancestors too, but not coalescent events. 3 So far, everything I’ve said has not depended on the number of identifiably different alleles‐ alleles that are identical by STATE. I’ve just been talking about history. And of course, while that history actually happened, we can’t know much about it when different gene copies have the same sequence. It’s the occurrence of mutations that give us identifiable polymorphisms in populations, and allow us to determine which copies have shared history, due to their being identical by state. In the case of this figure‐ gene copies were being replicated generation after generation, and most of that history, we can’t know after the fact. But surveying a population that has a G and T at a locus, we can determine that there was a mutation from G to T (or vice versa, technically) that gave us the two different alleleic states. And, we can use phylogenetic techniques that we’ve already learned to build gene trees of these copies. Instead of nodes that represent speciation events, though, we are going to see them as mutations, that give rise to new alleleic states! (I’m going to assume here that all mutations are neutral with respect to selection here). 4 These mutations will give us information on the existence of some of the coalescent events that occurred in the history of a population. Coalescent events that are not associated with mutations are, unfortunately, invisible to us. Looking backward in time, each time there is a coalescent event, then there is one fewer allele we have to consider. In this case, by four generations back in time, there are not 2 alleles to consider, but only one. (there are others that are unsampled at the present and in the past, but we don’t have to waste time worrying about those) 5 It’s important to understand that certain aspects of the demography of populations have predictable effects on the time of coalescence. If a population is small, then it doesn’t take long for an average allele to coalesce, whereas if it is a large population, then more generations, on average, will pass before alleles will coalesce. This is exactly in parallel with the pattern of fixation we talked about with genetic drift‐ small populations will drift to fixation faster than large ones, due to the extinction of all the non‐fixed alleles. In this case, all the existing alleles will have a more recent common ancestor if the population size is small (i.e. fixation for alleles that are IBD has taken fewer generations). Other aspects of population history can affect the recency of coalescence‐ shrinking populations will appear to have recent coalescent times, compared to expanding populations. 6 The nice thing about coalescent theory is that it is mathematically tractable. (we won’t do too much of it) The mathematics is involved, but it’s possible to show that the EXPECTED value of the time t for two randomly chosen alleles in a population to coalesce in population is a function of the (effective) population size Ne, specifically, it is 2Ne. The EXPECTED time for all the alleles in a population to coalesce is 4Ne. Effective pop size is a somewhat complicated term that has several loosely related definitions. In short, not all the individuals you could count in a population (the census size) are going to breed. Also, individuals are all related to each other to some degree or other, mating isn’t random, etc. etc. It is only weakly connected to census pop size, but is sensitive to inbreeding, unequal sex ratios, and things like population bottlenecks. Suffice it to say, the effective population size is some number that is usually smaller than the census population size, maybe by a lot. For example, humans have an effective population size that is only around 10,000. 7 If you know something about the rate of mutation‐ i.e. how often new alleles arise, then you could estimate the number of generations would be needed to account for the genetic diversity in your sample! If you know something about generation time‐ how much time passes before an individual has half her offspring (on average) you could then convert number of generations to the number of years for coalescent events to occur! By other mathematical tricks, it is possible to estimate effective population size, and if you assume that it is related to census size, you could look for increasing or decreasing population sizes in the recent past. Other applications infer the existence of subdivided populations, or migration rates, and so on‐ a very diverse set of interesting things can be learned about populations by application of coalescent theory. 8 So as you can see, the expected time to coalescence is just the beginning! There are a few other things to remember though‐ Anything that has a mean, has a variance. And in the case of expected coalescence times, the variance is pretty large, especially in smaller populations. What this means, practically, is that a single gene or a single locus, isn’t of much use. Think of it as the number of generations all the alleles in a randomly chosen locus to coalesce. It might take more, and another might take less. The Expected time is the mean coalesence time for a large number of alleles. The converse of this is, that if you can sample a large number of alleles, you can get quite accurate estimates of the coalescence time or any other parameter you’re interested in. Human genes coalesce between 200000 years and 8 mya! 9 By chance, some alleles in a given species will coalesce at times older than the existence of the species itself‐ in other words, they coalesce in the ancestral lineage. This means, that there was polymorphism at a particular locus that was passed down through the speciation event and that polymorphism may be retained in the daughter species for some time prior to fixation! This is shown in A, where sister species 1 and 2 contain sister alleles Purple and Green. The alleles were produced by a mutation that occurred in the ancestor of 1 and 2. Because they’re sister, if you reconstruct a phylogeny of the genes, you will get a tree that happens to match the history of divergence in these populations. BUT consider another gene in panel B. In this case, Green is sister to Blue allele, and those two then coalesce with purple. It’s entirely possible that Green, Blue, and Purple might even have been produced in the ancestor of species 1, 2 and 3! (and the ancestor would have had three alleles at the locus). Simply by chance, the allele‐lineages might be inherited and fixed in the daughter species in a way that did not reflect the order in which they were produced, and the gene tree, once reconstructed, might disagree with the species tree. 10 This problem gets worse when divergences aren’t very old, and populations are relatively large. The human species isn’t even 8 million years old, and while we have a small effective population size, other primates have much larger effective (not census!) sizes. This means that not every allele of every gene is unique to our species‐ in fact, some of the alleles at our genes were alleles of those genes in earlier primates, and we share many alleles with our closest relatives! Genetic variation can be passed down through speciation events, and the randomness associated with individual inheritance means that nearly a third of our genes support a relationship that disagrees with the overall pattern of similarity in the other 70% of the genome. In the early days of molecular phylogenetics, this was seen as a problem. Now, it’s seen as an expected feature of real history, and scientists use the parameters I mentioned to have a more nuanced view of the history of life‐ this is why the unification of population genetics and systematics has been so important. 11 12 With this lecture I want to take a look at some of the most important ways that sequence variation provides very specific information about the timing of evolutionary events, and the signatures of natural selection on DNA polymorphism. 1 Syn muts vs. non‐syn mutations. Read definitions. 2 One pervasive feature of gene sequences is that non‐synonymous sites seem to evolve at a much slower rate than syn sites, or non‐coding DNA such as introns or pseudogenes. Why? 3 Thought you might ask! To understand this, we should begin by breaking down what a substitution rate is- This is the rate at which mutations in a population become fixed. This is a product of two probabilities- the probability of a mutation occuring in the first place, and the prob. of it driftng to fixation. 4 The easiest way to estimate this is to calculate the number of new mutations, which simply is the number of gene copies (twice the size of the population in diploid species) times the mutation rate (RED) times the prob. that, at the instant the new mutation occurs, (in ONE gene copy in ONE of many diploid organisms) it drifts to fixation. (Blue). Both of these terms contain a 2N, which cancels out, leaving us with the surprising result that the substitution rate m equals the mutation rate µ. That means the rate of fixation of newly-arisen NEUTRAL mutations by genetic drift is independent of population size. If mutation rates are similar, substitution rates will be too. We can use this to compare rates of evolution in different populations or species. 5 Motoo Kimura (1968) proposed that most evolution at the molecular level is due to drift at neutral sites. Neutral substitutions should accrue in a clock‐like fashion, as we’ve just seen. If you look at the graph here of the number of substitutions that have occurred in a locus compared between species that have diverged at different times, you can see plainly that older divergences have more substitutions than more recent ones. Different types of DNA should evolve at different rates for reasons of ploidy (and copy number) or selection. 6 The use of molecular clocks has become quite sophisticated with maximum likelihood estimates of substitution rates providing more robust estimates of divergence times, and now molecular clocks are being used even in medicine, to date, for example, the emergence of viruses in human populations. The origin of the HIV virus in human populations has been dated to the early 20th century, and perhaps is related to the beginning of large urban settlements in Central Africa in the Colonial period. This also informs us that the virus went undetected in our species for over 80 years, killing only a few people in an underdeveloped part of the world. Not until the 1980s did it begin to occur at high frequency in western nations and gain the attention of doctors and researchers. 7 Recall the redundancy in the genetic code leads to non and syn mutations and how they work. The mutations that occur at 3rd codon positions are usually, but not always, syn. For example, a change between AGG and AGC makes the difference between Arg and Ser. 8 So, if you decode the different codons and infer the amino acid produced, then you can see that not all changes are meaningful to protein structure, while others are. Now let’s explore what gene trees would look like under the prevailing influence of different modes of selection. Under neutral drift, neither syn or non syn subs will be particularly selected for or against, and will be expected to occur randomly with respect to their effect on amino acids. Positive selection, however, will show that the number of non‐syn subs is higher than expected by chance‐ as estimated by the rate of syn subs. On the other hand, purifying selection is analogous to stabilizing selection‐ changes away from existing state are evolutionarilly worse‐ and selected out immediately. So, the only mutations that will become fixed are those that occur at syn sites, and drift to fixation. 9 A comparison of the relative frequency of synonymous subs versus non‐syn subs shows that in the lineage that leads to humans, there are two amino acids that are changed in the FOXP2 gene, which has some role in language ability, as shown by the negative effects mutant versions of this gene produce in their bearers. Overall, this gene has a very low non‐syn sub rate, however, with only 2 Non‐syn substitutions identified between other orangutans and mice! 10 There are many tests available to identify the fingerprints of natural selection in molecular sequences. Many of them compare sequences from different species, or different populations within species. In general, looking for different levels of polymorphism at synonymous sites versus non‐synonymous sites is one way, and looking for signs of reduced genetic diversity around a selected locus due to linkage disequilibrium is another. 11 Please if you have any questions, post them to the discussion board. 12 Evolution of Sex PCB4674 Evolution Dr. Norman Douglas Mechanisms of reproduction are diverse Populus tremuloides Mechanisms of reproduction are diverse Acropora palmata Mechanisms of reproduction are diverse Cnemidophorus neomexicanus Sex is both reproduction and recombination Recombinant Recombinant Gametes Chromosomes ↓ ↓ ↓ ↓ Sex is both reproduction and recombination Mechanisms of reproduction are diverse Ariolimax columbianus “Two-fold cost” of sex The effect of recombination on the evolution of MDR in polycultures of antibiotic- resistant strains of A. baylyi. Gabriel G. Perron et al. Proc. R. Soc. B doi:10.1098/rspb.2011.1933 ©2011 by The Royal Society Muller’s ratchet Red queen effect makes sex beneficial Example of red queen effect Image credits All images Zimmer & Emlen, Evolution: Making Sense of Life, except: http://www.fs.usda.gov/Internet/FSE_MEDIA/stelprdb5393642.jpg http://www.uniprot.org/taxonomy/6131 http://garden.org/courseweb/images/diagram.gif https://c4.staticflickr.com/6/5004/5308401379_f7e40ab52e_b.jpg https://classconnection.s3.amazonaws.com/1527/flashcards/691779/jpg/pars-of-a-flower.jpg Gabriel G. Perron et al. Proc. R. Soc. B doi:10.1098/rspb.2011.1933 http://scitechdaily.com/images/the-evolutionary-model-of-Muller’s-ratchet.jpg Welcome back. With this lecture, I want to look at a few ways that sexual selection can shape traits in animals that require mating to reproduce. 1 Anisogamy- in other words, the production of two different sizes of gametes, egg and sperm, is an important driver of behavior because it constitutes a difference between the sexes in their relative investment in offspring. A female’s reproductive success is often limited by the number of eggs she produces. 2 Females are limited by fecundity- the parent that produces the larger gamete, generally, has a higher investment in each offspring, and a lower limit on POTENTIAL offspring number over their lifetime. Usually this is the female, in which egg cells are much larger than sperm. But what really matters is overall investment: In species like seahorses and pipefish, the male accepts eggs from the female in a special pouch, where he fertilizes them and then spends a lot of time brooding them and taking care of them. So, even though the male could potentially fertilize a lot more eggs, his investment in these fish is actually higher than the females. 3 Males, typically, are limited in fecundity by the number of mates they can obtain. Females have certain paternity. They amost always know their offspring are theirs. Males have uncertain paternity, and there is always a chance that resources they invest in parental care could be directed toward offspring not their own. This leads to selection, frequently, for male behavioral strategies that seek to maximize the number of matings or fertilizations. Here you see that as the number of females that male reed warblers are able to guard increases, so does the number of offspring expected. 4 Even when species have a 50-50 sex ratio, this is a misleading description of the contribution of the sexes to the reproductive value of a population. When reproductive females, which can reproduce at a finite rate, are outnumbered by males, this sets populations up for sexual selection. 5 Darwin figured out the main aspects of sexual selection in one of the many books he produced following the publication of the Origin of species. In The descent of man and selection in relation to sex, he outlined mechanisms for sexual conflict and sexual selection between males for access to females (intrasexual), and for females to be selective in which males they mate with (intersexual). 6 Males often fight over access to females, these traits are well known. In many species there has been selection for large body size or weaponry (inter). Often, these characteristics serve as ornaments that advertise a male’s quality to females (intra) 7 To really see what this is about- look at the variance in reproductive success among the sexes. While females Elephant seals (bottom graph, in red, can expect no more than ten pups over their entire lives, the story for males is much different. Most males (like most females, actually) don’t successfully reproduce at all. And while some manage to have a few offspring, a few males have enormous numbers of offspring- up to 100. This extreme variance among males in fitness influences opportunity for sexual selection. Male contests over access to harems of females in this species are legendary. 8 All this competition is costly, as has been demonstrated with elk, in which antlers are grown and shed each year- Male elk have their highest number of offspring only after they reach 7-8 years old, but then their overall lifespan is reduced relative to females, which have a slow and steady repro rate. 9 Females may select males based on characteristics that benefit her directly or indirectly READ SLIDE 10 Direct benefits can take the form of so-called Nuptial Gifts. Male katydids provide females with nutrient-rich spermatophores that the female eats after mating- keeps her busy and reduces the chance of another male mating immediately, but also increases both the number and size of eggs she is able to produce. 11 The most extreme form of this is found in spiders, where males will allow themselves to be eaten as they mate- also increasing the time between female matings and provisioning her with extra nutrients for the male’s offspring. 12 On the other hand, Males may display elaborate ornaments – arbitrary traits that signal that a particular male is healthy enough –in other words has a high enough genetic quality- to get on with the business of surviving, even with a particualar often expensive set of characteristics- ornaments or behaviors that might otherwise decrease fitness. Successful males pass down their exaggerated traits- but here’s the less obvious thing: Females pass down their preference for males with exaggerated traits! Their daughters prefer males that have the characteristics of their fathers! 13 If you think this through, you can see that this has the potential to have a positive feedback loop, which results in often completely ridiculous traits being exaggerated until males simply can’t take it anymore, and good ol’ natural selection takes over. This process is expected to work, even if traits aren’t especially related to any honest signal of good genes- RA Fisher termed it the runaway hypothesis. 14 But most evidence indicates that there is some honest signalling of good genes though Females who choose males with attractive traits pass alleles to their offspring that influence expression of the trait and the preference. Leads to stronger preferences and larger display traits. Although traits that attract females may be arbitrary initially, they allow females to choose the highest quality males because costly display traits are more reliable indicators of male quality 15 Mating systems evolve because of the benefits and costs they confer on males and females Can depend on opportunities that arise from sexual selection In some species, a combination of systems evolves 16 For example, many bird species have a substantial amount of paternal investment in nest building and caring for young. But, even though females give the appearance of monogamy, genetic studies of chicks from individual nests reveals a hidden amount of extra-pair paternity, meaning that fathers are often raising chicks that aren’t theirs. Of course, those birds may well be fathering extra-pair chicks of their own with other females, too! 17 In species with many males mating with each female, sexual selection has even shaped the amount of sperm males produce. Humans, gorillas, and orangutans have relatively few partners at a time, and the weight of their testes is about what you’d expect for animals of their size (or even a little smaller). Female chimpanzees and baboons will mate with several males during estrus, and thus males with larger testes, capable of producing more sperm are favored, increasing the size of this trait in these species. 18 A particularly amazing example comes from deer mice (this is also the work of Hopi Hoekstra). They were able to demonstrate by staining techniques that sperm from individual fathers are able to recognize each other, and team up to form clumps that can swim toward eggs faster than individual sperm! This demonstrates how strong sexual selection is in these animals, and foreshadows our discussion of kin selection, which we’ll get to in the next module. 19 Thanks for listening, and please post any questions to the discussion board. 20 Welcome. With this lecture we will consider how Natural Selection works within populations, in particular in interaction with the other forces we’ve discussed already. 1 There are many instances where the function of single genes has been tied to fundamental changes in the behavior of organisms. Some of these traits are very clearly linked to fitness consequences for the organisms, as they affect certain aspects of mating or courtship behavior, or the sensitivity of organisms to social interactions among members of their species. 2 Innate, instinctual behaviors can sometimes be manipulated experimentally, breeding for hamsters that have higher or lower levels of running preference, or timidity in birds. 3 There is a famous experiment conducted by Dmitri Belyaev over several decades in Russia, in which captive foxes were selected for either tame or aggressive responses to humans. In this experiment, those foxes that were more sociable were bred together, and over only a few generations, the tame‐selected animals became as friendly as dogs. Interestingly, they did so by retaining a lot of juvenile traits, that we see commonly in domesticated dogs, such as variable coat color, floppy ears, and the willingness to bark as adults, something that is not done by adult foxes or wolves. When you strip away all the lingo, behavior is a coordinated response to external stimuli, and behavior can be shaped by evolution just like any other phenotype. 4 We discussed the work done on stickleback fish in previous modules. Behavior has changed repeatedly in these fish, too, as they move from predator‐rich marine waters into safer freshwater lakes. Freshwater fish spend less time in schools, with more preferring to live on their own, and they become more aggressive in defending their territories. 5 In the study of the evolution of behavior, Nikko Tinbergen laid much of the groundwork that has guided the questions of researchers since the 50s. For example, he broke questions about behavior into questions related to function‐ how a behavior actually manifests, and how it develops within organisms (learned, innate?) One could then begin to ask questions about how fitness is affected by the exhibition of a particular behavior, which would give clues into how it actually evolved. 6 Put another way, he advocated for clarifying explanations of behavioral evolution into proximate reasons for behavior, that focus on how a behavior is elicited, from studies ultimate, root causes of behavior, that focus on why particular behaviors have evolved. For example, if one is interested in why a particular bird has cliks in its song, one might look at the regions of the brain that are active during singing behavior, or whether there is a period in a young birds life during which it must be exposed to other conspecifics singing the song so it can learn the “right” song. Or, one might focus on the distribution of clicky songs among related species, and infer that the species of focus has clicky songs simply because its ancestor did, moving the point of focus to events in ancestral species. 7 Depending on your definition, even Plants can exhibit behaviors. Everyone is familiar with carnivorous plants that can quickly seize insects in sticky or snap traps, and certainly, they can can be sensitive to touch. But there are other more subtle, and slow, behaviors regarding chemical responses to damage and attack, for example, that plants communicate by sending and receiving chemical signals‐ sometimes through the air. 8 For the most part, we associate behavior with organsims that have nervous systems, though, made of neurons. 9 This particular tissue type evolved early in animal evolution‐ some of the earliest fossils of bilaterians show nervous system tissue that resembles living animals. 10 How this developed, however, is still a subject of a lot of investigation, however. Many of the genes invovled in nervous system development are found in non‐nerve‐bearing organisms. Some genes are present in plants, even. The overall story is that different componenets of the nervous system (even down to specific neurotransmittes) have origins at different times in the history of life. 11 For example, some proteins that are used have similarity to proteins that are used in the construction of the flagellae in Choanoflagellates, the protozoans that are the closest relatives to sponges. 12 Current evidence suggests animal nervous system either evolved once or twice, all depending on the phylogenetic position of these beautiful and interesting animals, the comb jellies. Though they look like jellyfish, they are different. They have nervous systems, and new studies place them as the sister group to all other animals, including the sponges. If this is the case, then that changes the likely order that nervous tissue developed‐ either gained twice in Ctenophores and the ancestor of other animals except sponges, or gained once and lost in sponges. 13 Early chordates began to show some concentration of nervous tissue near the anterior end of the animal, leading to what we’d call a brain. 14 Modern Vertebrate brains are divided into several specialized regions, that each have certain functions (often identified after the victim of a brain injury loses a particular ability). 15 These regions are retained among most vertebrates you’d look at, but in different lineages, there are greater or lesser percentages of tissue devoted to regions that are associated with different functions, possibly related in some way to the lifestyle of the organism. 16 One clever way to visualize this is to redraw an organism with it’s various body parts magnified according to the proportion of their brains devoted to those regions of the body. In various burrowing animals, facial tissues and forlimbs (used for sensing prey and digging) receive disproportionate allocations of space in the brain. We have a lot of attention given to our sense of touch and the ability to manipulate objects as you can see by our enlarged hands, and our sense of taste and ability to speak has also been reflected by added emphasis in brain anatomy. 17 Thanks, and please post questions in the discussion board! 18 Welcome. With this lecture we will consider how Natural Selection works within groups, in particular in interaction with the other forces we’ve discussed already. 1 To begin with, let’s consider the field of Game Theory. Game theory examines the ways that different strategies can interact in groups, and the expected outcomes of interacting strategies. This, it turns out, is instrumental in understanding many social interactions in the animal world. Of course, certain strategies may always result in higher fitness for the animal that employs them, in which case, natural selection would be expected to favor any genetic variation that was associated with these fit strategies. But, sometimes, different strategies are favored under different conditions, and rather than positive or negative directional selection driving the evolution of behavior, frequency‐dependent selection can take over. Regardless, An evolutionary stable strategy is a behavior that, when adopted by a population of players, cannot be invaded by an alternative strategy‐ in other words, organisms that adopt an ESS will be maintained in the population. 2 Let’s consider this example‐ side blotched lizards in the western US. Three different mating behaviors are employed by these lizards as outlined here. Conveniently, the throat colors on these lizards is associated with different behavioral strategies. Orange males defend large territories with many females and have high fitness. However, when there are many orange lizards that are so busy defending their territories, yellow lizards are able to sneak into the territories and mate with the females, increasing the number of offspring of the yellow males. If yellow males then become more common at the expense of orange, then they lose out. Yellow males don’t defend territories with females of their own. Blue males do however, and unlike orange males, they defend much smaller territories with only a few females. They can keep much better watch over their mates, and prevent yellow males from mating with their females. So, yellow does poorly when it gets too common, but Blue does well. Once blue becomes common, however, they are vulnerable to being run off their territories by orange males, who are more aggressive, and who attempt to “steal” females from blue males as they expand their territories. This means Orange does better once Blue becomes common. As you can see, this is the rock‐paper‐sissors game. Orange beats blue, blue beats yellow, yellow beats orange, depending on which one is most common at the moment. 3 Many species have developed the habit of living in packs, herds, flocks, schools, etc. There are costs and benefits to group living 4 For example, in African Wild Dogs, small packs consume significantly fewer calories per capita, than larger packs. 5 Living in a group may offer protection from predation, or increased availability of mates, or any number of other benefits. 6 The social contract comes with measurable costs, as well. In colony nesting birds, larger groups have a higher burden of parasites in the nests than do smaller groups. 7 Nevertheless, many organisms live in groups‐ and more than that, they often exhibit cooperative behaviors that may even be seen as “altruistic”, such as these MeerKats, which have individuals that stand watch for predators over the group. If a predator is found, the sentry sounds an alarm, which increases the chance that it will become the victim of the predator. Up until the 1960s, many behaviorists thought that social species were favored because altruistic behavior‐ in which one individual lowers his/her fitness on behalf of the groups’‐ that these traits evolved for the good of the species. 8 One of the longest running debates‐ in evolutionary biology concerns the possibility of selection of groups to contribute meaningfully to adaptation. In other words, can selection of groups of individuals that share a trait cause those groups to persist and expand at the expense of other groups. Actually, calling it a debate is too kind, there is an overwhelming consensus that only NS working at the level of individuals is required, and group selection is implausible and unlikely. It is because individual selection predominates that behaviors beneficial to individuals will evolve even if detrimental to groups. Also, it is very hard to define groups & their members through time because they have migration. 9 WD Hamilton in 1964 realized that allele frequencies can be increased by natural selection, even if their bearer suffers lower fitness. Many altruistic behaviors reflect situations like this. In short, the idea is that an organisms total fitness controlled not only by the number of offspring they leave directly, but also by the number of allele copies they leave. 10 Hamilton came up with a very simple rule, that altruistic alleles increase whenever they can leave the most offspring, whether themselves, or by virtue of helping their relatives have more offspring. If the benefits of a particular strategy, results in benefits to relatives that carry the same alleles and these weighted benefits outweigh the costs,, then that variant can be favored. 11 What makes altruism an evolutionarily stable stragegy, then, is the fact that animals that live in groups are often fairly close relatives. If you examine this table you can see the numerical value in the center, r. r is the coefficient of relatedness, which can be thought of as the percentage of alleles two individuals share. So a parent will share have of their alleles with their offspring. Siblings with the same parents, do, to. Each grandparent shares only 25% of your alleles, and so on. Calculating the coefficent of relationship for other situations becomes more complex, particularly if there is inbreeding, but it can be done. 12 Many birds will engage in altruistic behavior‐ forgoing reproduction (especially when they are young) in favor of helping their parents raise more brothers and sisters. 13 In white‐fronted bee eater birds from Africa, there is nearly ½ an offspring, on average, added to an individual’s fitness by raising siblings over what the bird might produce on its own in its first year of attempting to breed, and the liklihood that a bird will engage in cooperative behavior is directly related to the relatedness of the bird to the beneficiaries of his help. 14 Bees, wasps, ants have haploid males and diploid females Diploid females (all sisters) share ¾ of alleles with each other, but only ½ with queen (or potential offspring!) Higher relatedness means winning strategy is to help queen produce more sisters. Thus we see how social behavior can be promoted evolutionarily if you can increase the number of your alleles by helping relatives have higher fitness! 15 Thanks, and please post any questions to the discussion board! 16 Welcome. In this module, we’ll be discussing species. This is paradoxically one of the most essential and most frought concepts in evolutionary biology, and for years, numerous alternative concepts have been offered purporting to be biologically meaningful, or universally applicable, or emphasize the most important aspects of biological diversification. 1 To begin with, it’s pretty clear that life falls into clear categories. There are certain groups, such as these warblers, that have several recognizablely different types. Even in very diverse taxa don’t consist of a continuum of morphological variation smearing into each other. 2 Even to the untrained eye, it’s clear that there are different kinds of organisms. You probably don’t know much about mosses, but you can see two different types in this clump pictured here, right? 3 Darwin‐ who titled his book, On the Origin of Species, ironically had almost nothing to say about the process of speciation, and he had a working definition that seems now to be very flexible READ DEFINITION where it seems that there is no clear rule about when two different types might be species or varieties. In this case, you might wonder, are species even real? Do they represent something special, an actual product of evolution, or are they just convenient groupings? 4 Well, in fact, many biologists have a very operational species definition‐ basically, that they know it when they see it. So long as a group is different somehow, in a recognizable manner, you can call it a different species. Art Cronquist enunciated such a concept, now called the Morpho Sp. Con. That is pretty satisfactory for a lot of taxonomists. In fact, this is the actual default position of most biologists, that species are what we recognize, and just “being different than everything else” is the sum total of evidence ever offered for the existence of almost all described species. 5 Looking more deeply at certain groups, the shortcomings of this method become apparent. For one, the MSC is blind to cryptic species, that don’t differ morphologically, but may still represent independently evolving groups. These two birds were considered one species prior to the advent of DNA barcoding that showed that there were two speices that did not interbreed. Even though WE can’t tell them apart easily, they can recognize each other well enough. You can easily think of other strengths and weaknesses of a concept like this‐ for example, a paleontologist might default to this conception of species, because they don’t have much evidence of behavior or genetics. Organisms with very simple morphology, like bacteria, might be difficult to classify by this method. 6 With the dawn of the Modern Evolutionary synthesis and the unification of genetics and darwin’s ideas, it became clear that one thing that held species together was the exchange of genetic material. Ernst Mayr authored a very influential hypothesis about the nature of species, decreeing them to consist of READ DEF. This definition has been almost universally adopted as the textbook definition of species, because it defines groups in which genes can be exchanged. 7 Despite its esteemed position in biology, the BSC has a number of obvious shortcomings. For one, what about asexual lineages? Many microbes have never, or only rarely, been observed in a sexual state. The mold that produces penicillin was only discovered in its sexual phase in the early 2000s. Many bacteria divide continuously without regularly exchanging genes, or they may simply pick up random DNA from the environment or very different bacteria and incorporate it into their genomes, a process known as HGT. In these cases, the BSC is very difficult to implement. 8 Many sexual eukaryotes still hybridize. Oak trees are famous for this; this diagram depicts hypothesized pairs of white oak morphological species from Eastern North America that have been seen to hybridize and form fertile offspring. You can see that some identifiable species (like Quercus virginiana one the iconic Live Oak of the SE, seen all over the UF campus, the bottom circle. It hybridizes with at least six other oaks!), By the BSC, there might only be three species of oak in this group, instead of sixteen! 9 Many species limits can’t be tested. Of course paleontologists can’t study reproductive compatibility, yet they can recognize species in the fossil record. They’ve described a number of Tyrannosaurus species without knowing about their breeding behavior. I suspect that if they could travel in time, they’d still be hesitant to actually test whether any of these species could mate! 10 It goes the other way, too‐ by the BSC, Chihuahuas and Great Danes would be different species, because even though they are in theory interfertile, mating can’t happen without in‐vitro fertilization, and can only work if the Chihuahua is the male. 11 Ultimately, though, the issue is that reproductive compatibility is ancestral character state! Look at this phylogeny depicting a clade of 4 species, a‐d. The ancestral taxon had reproductive compatibility. If B and D, respectively, evolve reproductive isolation from the other species, then fine, they’re biological species, but what about A & C? They should still be interfertile, and the same species. 12 The obvious problems with the BSC let taxonomists to propose that evolutionary history be the predominant criterion for recognizing species. Phylogenetic systematists‐ cladists, like Joel Cracraft – were making great strides in uncovering the tree of life, and so were increasingly confident that species themselves could be identified on the basis of synapomorphies (or technically, apomorphies, because synapomorphies are derived characters that are shared between species). If there’s a reproductively interfertile group that has a diagnosible character, then it’s a phylogenetic species. 13 This can produce some weird situations too, unfortunately. Instead of A‐D representing species, now, let’s let them represent populations that are all interfertile, but are diagnosably different from each other due to Characters 1‐4. Thus, A‐D are all different species. 14 But what happens if only population B has an apomorphy? Then, a, c, and d become another species. But while B has common ancestry, the group of population a, c, and d is “paraphyletic”. 15 From a phylogenetic species concept, the process of speciation has a particular consequence. When one species splits into two, that species becomes extinct, and two new species are formed. So, we have species that existed at some point, that may never have been seen, that only “vanished” due to a speciation event. This is considered true in phylogenetic systematics in any case. 16 Phylogenetic species also require monophyly, and this is only something that, if you remember our discussion of coalescence, only happens after reproductive isolation. If you look at a phylogeny of alleles sampled early after speciation, you will find non‐ monophyletic species, as shown in the lower phylogenetic tree. After time has passed, and alleles have coalesced within each species, species alleles become reciprocally monophyletic. At each locus you sample will have something like this happening; after a long period of separation, gene trees may become reciprocally monophyletic, but for a long time, polymorphism will remain, even after cessation of interbreeding. There are still genes in our species for which we share polymorphic alleles with other primates, for example. 17 At the root of this “problem” is that the idea of species is trying to do too much, at the same time, we need to have some way to discuss the groups that are the products of evolution! Depending on your point of view, the most important job that species concepts do for us may differ. An ecologist might want to count the species of tree in a forest, a taxonomist may simply want to write a description of a new species and want to know how to describe it‐ these scientists will be most concerned with the pattern of diversity, and need to have an organized system of recognizable species. On the other hand, population geneticists will want to study the process of divergence and may want to focus on the evolution of reproductive isolating mechanisms. This isn’t irrelevant‐ for example consider the endangered species act, that grants legal protections to recognized species. This legislation doesn’t say much about how to define species or what threshold an endangered species needs to meet! 18 Recognizing that the species problem was one of definition; that species exist, and that the process of speciation differs in each situation, Kevin de Quieroz has proposed that one common pattern in most species concepts, the idea that there is an interbreeding lineage of organisms that continues through history, suffice for species existence. Species status then becomes a hypothesis that can be corroborated by, for example, by morphological evidence, or reciprocal monophyly (or partial monophyly), or especially, complete reproductive isolation. In other words, the elements of process that are considered not as arbitrary criteria that must be strictly met, but recognizes that there is a lot of diversity in the way that evolution proceeds in different lineages. The debates over species concepts have simmered down lately, as people have realized the futility of finding a one‐size fits all definition. Most people now are satisfied with the idea that species are contingent hypotheses, subject to corroboration or refutation in light of additional evidence. 19 So you might wonder, have we come back around to Darwin’s view of species? We certainly know a lot more now that Darwin did, specifically about the genetics of the speciation process, and, we have the capability to know with astonishing precision the pattern of divergence between and within species. But I think that Darwin would be very comfortable with the idea that there is no clear threshold, no instant at which a new species is born, but instead, species are the endpoint of divergence that may occur for a variety of reasons and by differing pathways. In the next lectures, we’ll discuss some of these. 20 Thanks, and please post any questions to the discussion board! 21 Welcome. In this lecture we’ll look at some of the many mechanisms by which different populations become reproductively isolated. 1 Fundamentally speciation involves the evolution of barriers to reproduction. What this really means is that one population enter breeding individuals becomes divided by some mechanism into two populations, in which breeding takes place within the new populations, but they no longer exchange genes. Of course, when we talked about species concepts, we saw there are many ways in which reality is a little messier this idealized version, but for the purposes of this lecture let's just focus on the splitting of one lineage into two. 2 Isolating barriers or mechanisms can be categorized into two main groups. The first is composed of geographic barriers that prevent populations on either side of some barrier from exchanging genes. When geography is the primary force involved in separating populations, that is called “allopatry” for “different countries”. Populations of some taxon that are not separated by a geographic barrier are said to be in sympatry, which means the “same country”. While it's easy to see how allopatric populations are unable to exchange genes, understanding the isolating mechanisms that prevent sympatric populations from exchanging genes is a little harder. 3 For example the American elk and the Eurasian red deer could interbreed if they ever encountered each other, but being distributed on different continents, they never get the chance. This is a dramatic example of allopatric speciation, where these two animals are free to evolve separately. 4 Through geological time changes in the earths surface such as the formation of valleys or mountains or other large‐scale changes can certainly create or destroy connections between different habitats. When this happens, new subpopulations are free to evolve through the process of genetic drift or natural selection, and differences between populations will begin to accumulate. 5 Sometimes changes in the Earth's surface are dramatic enough to affect many populations at once. A very well‐known instance of this was caused when the Ithsmus of Panama emerged during the Miocene or Pliocene. Populations of many marine organisms that lived in the area of the Central American Seaway became subdivided by the formation of the ithsmus. Molecular phylogenies estimate that the dates of divergence between pairs of closely related species occurring in the Pacific and the Caribbean on either side of the ithsmus to the Miocene or Pliocene. This is also a good reminder that different organisms perceive the landscape in very different ways. The formation of the Ithsmus of Panama actually brought together populations of many terrestrial taxa just as it separated populations of marine organisms. 6 Volcanic islands also presented a really amazing opportunity for new species to form as they are colonized buy organisms from other parts of the world. The Hawaiian Archipelago has a rich, and highly threatened, biota that evolved as initial colonists underwent amazing adaptive radiations. This figure shows a molecular phylogeny of Hawaiian Cricket in the genus Lappula that diversified in the Hawaiian Islands as the archipelago was forming. Take note of the molecular dating results at the nodes indicated by the colored dots. The oldest divergence is dated to 3.7 million years ago. Other divergences are more recent. The species that involved from these common ancestors are found on successively newer islands. To illustrate… 7 … look at the estimated dates of emergence of each of the different islands in the Hawaiian Archipelago. The oldest island Niihau and Kauai are the farthest west while the newest islands, particularly the Big Island, Hawaii proper, are the most recent. The pattern of divergence on the molecular phylogeny shows that initial colonists of lobular were on Hawaii and then dispersed to new islands as they were formed by volcanic eruptions. 8 So it's clear that allopatry is responsible for all lot of the diversification of life on earth. But there's only so many volcanic islands and mountain ranges and new rivers out there. What other mechanisms do organisms have that keep recently diverged taxa from merging back together after they come back into contact? For one, organisms could differ in time of day, or season that they choose to reproduce. Monostrea corals release their gametes at different times of the night, increasing the probability that each species’ gametes will find the proper counterpart. Wind pollinated plants may release their pollen at different times of the year. Two interfertile and common live Oaks in Gainesville actually shed pollen about six weeks apart, one in the spring and the other in early summer. 9 Different floral morphology on plants often attracts different pollinators. In the well studied monkey flowers, some species are pollinated by bees, While other closely related species, are pollinated by hummingbirds. As we learned in a previous module researchers are using genetic techniques to uncover genes involved in generating the different floral morphologies. Because these floral morphologies prevent interbreeding between the two species, these genes can be thought of as speciation genes! 10 Many organisms, Especially marine animals that spawn by simply releasing gametes into the ocean, rely on the gametes themselves being able to recognize when fertilization is appropriate. There are a number of diverse genetic mechanisms where sperm are attracted to eggs by particular species specific chemical cues, or where eggs prevent fertilization by sperm cells from the wrong species So far, the mechanisms we’ve discussed serve to prevent fertilization from ever taking place between closely related species. How do traits like these evolve? It could just be due to evolution proceeding in different directions. After a long period of separation, differences will happen. Or, is there more to it than that? 11 Well it turns out there is a lot more to it than that. It begins with the observation that when mating happens between pairs of the recently diverged populations, the offspring often have lower fitness then either of the parental populations. This can be due to a lot of reasons. The hybrids could simply not develop properly and be inviable they could be sterile there could also be ecological or behavioral problems with hybrid offspring that lowers their fitness. 12 For example you may be aware of that donkeys or burros and horses are in the same genus Equus. They're pretty closely related to each other. But they do differ. He's have 62 chromosomes and horses have 64. When mating occurs between a horse and a donkey a gamete with 31 chromosomes pairs with a gamete with 32 chromosomes… 13 … forming an embryo that has 63 chromosomes. This is known as a mule. People breed mules all the time. They inherit stamina and strength from their donkey parent and size and trainability from their horse parent. 14 Having an odd number of chromosomes doesn't mean that the hybrid animal doesn't survive, although that certainly true in some cases. Sometimes they do just fine in spite of their chromosomal abnormalities. But because mules have 63 chromosomes, they can't form proper gametes because their chromosomes cannot pair up during meiosis, so they’re sterile. 15 There's an interesting way that plants are able to overcome hybrid sterility sometimes. If a plant that had an uneven number of chromosomes produced unreduced gametes (not an unusual error), and then self– fertilized, the seeds would have twice the number of chromosomes. In other words, a polyploid would have been formed. But these offspring, even though they have twice the number of chromosomes, would be interfertile with each other and reproductively isolated from either of the parental species. This is, In effect, instant speciation. It happens all the time in flowering plants, Because most species of flowering plants produce both pollen and ovules in the same flower, and can self– fertilize, it’s possible for occasional diploid sperm to fertilize diploid eggs, and produce a tetraploid seed. 16 Researchers at the University of Florida Study the genus Tragopogon. Species from different parts of Europe and Asia were introduced to Washington State about 100 years ago where they hybridized and formed to new species by polyploidization. So, not only has the birth of at least two new species been directly observed, scientists in this lab are able to re‐create the new species by artificially hybridizing the parental species and encouraging chromosome doubling. The artificial polyploids are then interfertile with the naturally occurring polyploid plants! In fact many polyploid plants probably originate multiple times. 17 Sometimes divergence between two new species in allopatry leads to genetic changes with that prove to result in incompatibilities when the species are brought into contact. In a different pair of monkey flower species Mimulus guttatus and M. nasutus, Andrea Sweigart found that the genotypes at two different loci, hybrid male sterility1 and hybrid male sterility 2, resulted in lower fitness in hybrid offspring. She used QTL analysis to map these genes to their chromosome locations, and in fact they occur on different chromosomes and are inherited independently of each other. 18 If plants inherit and allele from Mimulus guttatus at the hybrid male sterility 1 locus, then they must inherit at least one guttatus allele at the hybrid male sterility 2 locus. Plants that have guttatus HMS1 and only nasutus HMS2 produce sterile pollen. 19 If a hybrid offspring between two species is sterile, it has a fitness of zero, and whether you're talking about horses or monkey flowers, the same logic applies. You'd expect that any mechanism that prevented horses and donkeys for example, from recognizing each other as potential mates would be favored by natural selection. A mare that spent two years gestating and raising sterile mule colt will have lower fitness than a mare that only mated with other horses. 20 So that’s why we have such a large number of different pre‐mating isolating mechanisms! A very diverse set of pre‐mating reproductive isolating barriers has been characterized from a number of species. Figure 13.7 describes a lot of these, and it's very interesting to see how many different ways nature has of keeping species a part. And, in fact, a lot of times these mechansims aren’t perfect! 21 It's been known for a long time that polar bears and brown, or grizzly bears are ve