Medical Biochemistry Lecture Notes PDF
Document Details
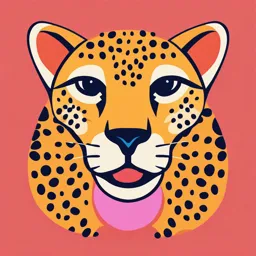
Uploaded by SaneChrysoprase2099
Gondar University
2004
Solomon Adugna, Lakshmi Ahuja Mekonnen Alemu, Tsehayneh Kelemu, Henok Tekola, Belayhun Kibret, Solomon Genet
Tags
Summary
This document is a set of lecture notes on medical biochemistry, intended for health science students at Gondar University, Jimma University, and Debub University in Ethiopia. The document covers various topics including enzymes, carbohydrate metabolism, lipid metabolism, amino acids and proteins, and vitamins. It was produced in collaboration with the Ethiopia Public Health Training Initiative, The Carter Center, and the Ethiopia Ministry of Health, and the Ethiopia Ministry of Education and was funded by USAID.
Full Transcript
LECTURE NOTES For Health Science Students Medical Biochemistry Solomon Adugna, Lakshmi Ahuja Mekonnen Alemu, Tsehayneh Kelemu, Henok Tekola, Belayhun Kibret, Solomon Genet Gondar University, Jimma University, Debub University In collaboration with the Ethiop...
LECTURE NOTES For Health Science Students Medical Biochemistry Solomon Adugna, Lakshmi Ahuja Mekonnen Alemu, Tsehayneh Kelemu, Henok Tekola, Belayhun Kibret, Solomon Genet Gondar University, Jimma University, Debub University In collaboration with the Ethiopia Public Health Training Initiative, The Carter Center, the Ethiopia Ministry of Health, and the Ethiopia Ministry of Education 2004 Funded under USAID Cooperative Agreement No. 663-A-00-00-0358-00. Produced in collaboration with the Ethiopia Public Health Training Initiative, The Carter Center, the Ethiopia Ministry of Health, and the Ethiopia Ministry of Education. Important Guidelines for Printing and Photocopying Limited permission is granted free of charge to print or photocopy all pages of this publication for educational, not-for-profit use by health care workers, students or faculty. All copies must retain all author credits and copyright notices included in the original document. Under no circumstances is it permissible to sell or distribute on a commercial basis, or to claim authorship of, copies of material reproduced from this publication. ©2004 by Solomon Adugna, Lakshmi Ahuja Mekonnen Alemu, Tsehayneh Kelemu, Henok Tekola, Belayhun Kibret, Solomon Genet All rights reserved. Except as expressly provided above, no part of this publication may be reproduced or transmitted in any form or by any means, electronic or mechanical, including photocopying, recording, or by any information storage and retrieval system, without written permission of the author or authors. This material is intended for educational use only by practicing health care workers or students and faculty in a health care field. Table of Contents Table of Contents.................................................................................................................. i Acknowledgement................................................................................................................. v Introduction.......................................................................................................................... vi Solomon Adugna and Lakshmi Ahuja Unit One: Enzymes............................................................................................................... 1 Henok Tekola Principles and classifications of Enzymes.......................................................... 5 Mechanism action of Enzymes.................................................................................. 7 Enzyme Inhibition.............................................................................................. 13 Regulation of Enzyme activity........................................................................... 18 Enzymes in clinical diagnosis............................................................................ 19 Unit Two: Carbohydrate Metabolism..................................................................................... 23 Belayhun Kibret Chemistry of Carbohydrates................................................................................ 23 Functions of Carbohydrates................................................................................. 31 Digestion and absorption of Carbohydrates......................................................... 33 Glycolysis............................................................................................................. 35 Glycogen Metabolism........................................................................................... 42 Glycogen storage diseases.................................................................................. 45 Pentose phosphate pathway................................................................................ 46 The Cori - cycle.................................................................................................... 48 Gluconeogenesis................................................................................................. 49 Unit Three: Integrative Metabolism Bioenergetics................................................................ 54 Tsehayneh Kelemu Introduction.......................................................................................................... 54 Structural basis of high energy phosphate.......................................................... 55 Formation and utilization of ATP.......................................................................... 56 Catabolism of fuel molecules............................................................................... 56 Concept of Free energy....................................................................................... 58 Oxidation-reduction reactions.............................................................................. 58 Aerobic energy generation................................................................................... 61 Kreb’s cycle.......................................................................................................... 63 Function and regulation of kreb’s cycle............................................................... 70 i Electron transport system and oxidative Phosphorylation................................... 71 Respiratory control............................................................................................... 77 Uncouplers........................................................................................................... 77 Respiratory poisons............................................................................................. 78 Unit Four: Lipid Metabolism.............................................................................................. 80 Solomon Genet Classification of Lipids......................................................................................... 80 Types of Lipids..................................................................................................... 80 Digestion and absorption of Lipids....................................................................... 86 Metabolism of Fatty acids and Triacyl Glycerols................................................. 88 β-Oxidation of Fatty acids.................................................................................... 90 Metabolism of Ketone bodies............................................................................... 93 Biosynthesis of Fatty acids and triacyl glycerols.................................................. 97 Cholesterol Metabolism....................................................................................... 99 Atherosclerosis..................................................................................................... 101 Hypercholesterolemic drugs................................................................................ 101 Lipid storage diseases......................................................................................... 102 Fatty Liver............................................................................................................ 102 Lipoproteins.......................................................................................................... 102 Chemical compositions of Membranes................................................................. 104 Unit Five: Amino acids and Proteins.................................................................................... 105 Solomon Adugna Classification of amino acids................................................................................ 106 Properties of amino acids.................................................................................... 115 Peptides............................................................................................................... 119 Glutathione synthesis........................................................................................... 121 Proteins................................................................................................................ 124 Classifications of proteins.................................................................................... 124 Levels of organization of proteins........................................................................ 126 Denaturation of proteins....................................................................................... 131 Hemoglobin and Myoglobin................................................................................. 135 Sickle cell Hemoglobin......................................................................................... 136 Sickle cell disease, Sickle cell trait........................................................................ 136 Digestion and absorption of proteins................................................................... 137 Amino acid catabolism......................................................................................... 141 Nitrogen balance, excretion and the urea cycle.................................................... 144 ii Defects in the urea cycle....................................................................................... 150 The Glucose - Alanine cycle................................................................................. 151 Inborn errors of amino acid metabolism................................................................ 152 Amino acid derived nitrogenous compounds........................................................ 154 Clinical problems................................................................................................... 159 Unit Six: Vitamins and Coenzymes...................................................................................... 161 Solomon Adugna Water soluble vitamins......................................................................................... 161 Chemistry, sources, function and deficiency of: Thamine............................................................................................................... 161 Riboflavin............................................................................................................. 163 Niacin................................................................................................................... 164 Pyridoxine............................................................................................................ 165 Biotin.................................................................................................................... 166 Cobalamin............................................................................................................ 167 Folic acid.............................................................................................................. 169 Panthothenic acid................................................................................................. 170 Ascorbic acid........................................................................................................ 171 Fat soluble vitamins............................................................................................ 172 Chemistry, Sources, function, deficiency and Hypervitaminosis of: Vitamin A.............................................................................................................. 173 Vitamin D.............................................................................................................. 177 Vitamin E.............................................................................................................. 179 Vitamin K.............................................................................................................. 181 Unit Seven: Miniral Metabolism........................................................................................... 183 Lakshmi Ahuja Mineral................................................................................................................. 183 Sodium and potassium........................................................................................ 183 Calcium and Phosphate....................................................................................... 184 Trace elements.................................................................................................... 185 Iron....................................................................................................................... 185 Copper................................................................................................................. 187 Magnesium........................................................................................................... 188 Flourine................................................................................................................ 188 Iodine................................................................................................................... 189 Zinc...................................................................................................................... 189 iii Selenium.............................................................................................................. 191 Unit Eight: Hormones........................................................................................................... 193 Lakshmi Ahuja Definition and classification.................................................................................. 194 Biosynthesis, storage, transport........................................................................... 196 Mechanism of action of steroid hormones........................................................... 197 Mechanism of action of protein hormones........................................................... 198 Receptors and diseases....................................................................................... 202 Second messengers............................................................................................. 203 Insulin synthesis, secretion and metabolic role.................................................... 205 Diabetes mellitus.................................................................................................. 209 Symptoms and complications of DM.................................................................... 210 Glucagon.............................................................................................................. 211 Clinical aspects..................................................................................................... 212 Thyroxin................................................................................................................ 213 Synthesis and Metabolism................................................................................... 213 Hypo and Hyper Thyroidism................................................................................. 214 Goiter.................................................................................................................... 215 Catecholamines.................................................................................................... 216 Pheochromocytoma.............................................................................................. 217 Case Histories...................................................................................................... 217 Unit Nine: Molecular Genetics.............................................................................................. 220 Mekonnen Alemu Structure of Nucleic acids..................................................................................... 220 Types of Nucleic acids......................................................................................... 226 Replication of DNA............................................................................................... 230 DNA Damage and repair mechanism.................................................................. 231 RNA synthesis..................................................................................................... 233 Post transcriptional Modifications......................................................................... 236 Translation/Protein synthesis............................................................................... 240 Regulation of protein synthesis............................................................................ 242 The Genetic code................................................................................................. 243 Post translational processes................................................................................ 244 Glossary.................................................................................................................................... 246 Reference................................................................................................................................. 253 iv ACKNOWLEDGMENT The team of authors from the department of Biochemistry of various Universities would like to thank and express their deep rooted gratitude to the Carter Center (EPHTI) for its initiation and drive to prepare this teaching material. The Center has not only supported the team financially, but also stood behind it firmly throughout the entire period of this experience. Thus Carter Center has become the pioneer in the field of preparing teaching material and also in training a team of authors for future endeavors of the kind. In addition, the task would have been impossible without the directing of the Federal Democratic Republic of Ethiopia, Ministry of Education. It is also not out of place to thank the administration of Gondar University, Debub University and Jimma University for extending cooperation whenever it was needed. The authors extend their appreciation to Ato Akililu Mulugeta, Manager, Carter Center, who has shepherded the team’s effort through manuscript to finished pages. The authors are particularly grateful to Abdel-Aziz Fatouh Mohammed (Ph.D) Associate professor of Biochemistry, Medical Faculty, Addis Ababa University and Ato. Daniel Seifu, Lecturer of Medical Biochemistry, Medical Faculty, Addis Ababa University for their highly professional editing and most helpful comments about many aspects of the text. v INTRODUCTION TO BIOCHEMISTRY Medical biochemistry is an essential component of curriculum for all categories of health professionals. Contemporary Biochemistry plays a crucial role in the Medical field, be it metabolic pathways, storage diseases, mechanism action of varied biomolecules or inter and intra cellular communications. A lecture note on Medical biochemistry integrates and summarizes the essentials of the core subject. Topics are carefully selected to cover the essential areas of the subject for graduate level of Health sciences. The chapters are organized around the following major themes: 1. Conformation of biomolecules, structure and their relationship to biological activity 2. synthesis and degradation of major metabolites 3. Production and storage of energy 4. Biocatalysts and their application 5. Intercellular communication by hormones 6. Molecular events in gene expression and regulation Enzymes: Body proteins perform a large number of functions. One such unique function is, they act as biological catalysts (Enzymes).They are responsible for highly complex reactions. They direct the metabolic events and exhibit specificity toward substrates, regulate the entire metabolism. Thus, they play key role in the degradation and synthesis of nutrients, biomolecules etc. The most important diagnostic procedures involves assay of enzymes. They assist to know damaged tissues, the extent of tissue damage, helps to monitor the course of the disease and used as a therapeutic means of diagnosing a vast array of diseases. Amino acids and Proteins Living systems are made up of Proteins.They are the dehydration polymers of amino acids. Each amino acid residue is joined by a peptide linkage to form proteins. Proteins are the molecular instruments in which genetic information is expressed, Hormones, Antibodies, transporters, the lens protein, the architectural framework of our tissues and a myriad of substances having distinct biological activities are derived. The type, nature and number of amino acids impart characteristic properties to the proteins. There are about 300 amino acids, but only 20 are coded by DNA of higher organisms. Acid base properties of amino acids are important to the individual physical and chemical nature of vi the protein. The structural organization of proteins could be primary, secondary, tertiary and quaternary. The three dimensional structure is the most biologically active one. The unfolding and disorganization of the proteins results in denaturation, the process is mostly irreversible. Such a protein may lose its biological function. Many amino acid derived peptides are of biological importance and special products formed from them are of critical importance to the body. Carbohydrates They are biomolecules, found abundantly in living organisms. They contain more than one hydroxyl group (polyhydric) In addition to aldehyde or ketone group. Thus, they form in to polyhydroxy aldoses or polyhydroxy ketoses. Carbohydrates can be classified in to Monosaccharide, disaccharide, and polysaccharides. Mono is the smallest sugar unit, disaccharide is made up of two monosaccharides joined by glycosidic linkages.The linkage can be α or β. A polymer with more than 10 monosaccharide units is called polysaccharide. Carbohydrates have a wide range of functions. They provide energy; act as storage molecules of energy. Serve as cell membrane components and mediate some forms of communication between cells. Absence of a single enzyme like lactase causes discomfort and diarrhea. The failure of Galactose and fructose metabolism due to deficient enzymes leads to turbidity of lens proteins (Cataract). Blood glucose is controlled by different hormones and metabolic processes. People suffer from Diabetes if the insulin hormone is less or not functioning well, such people are prone to atherosclerosis, vascular diseases, and renal failure. Integrative Metabolism and Bioenergetics Oxygen is utilized for the conversion of glucose to pyruvate. The same metabolite also forms from amino acid and protein metabolism. Other precursors like Glycerol, propionate can give rise to pyruvate. The main breakdown product of pyruvate is acetyl CoA, which is the common intermediate in the energy metabolism of carbohydrates, lipid and amino acids. It enters central metabolic pathway, the Citric acid cycle in the mitochondrial matrix. Here it is converted to CO2, H2O and reduced coenzymes (NADH, FADH2). These reduced nucleotides are the substrates for oxidative Phosphorylation in mitochondria, their oxidation provide the energy for the synthesis of ATP, the free energy currency of cells. vii Lipids The bulk of the living matter is made up of Lipids, carbohydrates and proteins. Lipids are water insoluble, but can be extracted with non-polar solvents like Benzene, methanol, or ether. Some lipids act as storage molecules for example triglycerides stored in adipose tissue. Transport forms of lipids (Lipoproteins),are present in combination with proteins Building blocks of lipids are fatty acids. Some lipids like cholesterol lack fatty acids but are potentially related to them. Lipids are constituents of cell membrane and act as hydrophobic barrier that permits the entry/exit of certain molecules. Lipids carry fat soluble vitamins and form special biomolecules. Lipid imbalance can lead to serious diseases like obesity and atherosclerosis. Break down of fatty acid produce energy, excessive breakdown cause ketosis, ketoacidosis, coma and death. Cholesterol level in blood is controlled by several regulatory mechanisms. Such information is applied in the treatment of patients with high cholesterol levels. Vitamins and Minerals They are organic compounds required in small quantities for the functioning of the body. They are not synthesized in the body, needed to be provided in the diet. Vitamins do not generate energy. Generally they are responsible for the maintenance of health and prevention of chronic diseases. Grossly there are two groups’ Water soluble vitamins are Vit. B-complex and C. Fat soluble vitamins are Vit A, D, E, and K. Minerals are elements present in human body.Elements like C,H,N are provided by the diet and water.Second group includes Ca, P, Mg, Na, K. Cl and Sulphur. These are required in large quantities (100mg or more/day). They are called Macro elements. A third group includes trace elements, which are required in small amounts for example Fe, I, Zn, etc. Fluorine deficiency associated with tooth decay, excess of it causes fluorosis. Sources and requirement are of physiological importance. The metabolic role and deficiency disorders are important for the students of health sciences. Vitamins and trace elements are particularly important for patients with gastrointestinal disorders, who are fed on artificial diets or parenteral nutrition. viii Hormones Hormones are chemical messengers secreted by endocrine glands and specific tissues. They reach distant organs and stimulate or inhibit the function. They play important role in carrying messages to the various organs. They form part of a signaling system. Hormones are synthesized in one tissue, secreted in to blood, transported as mobile messengers.When they reach target tissue, they exhibit their actions. Defect in the secretion, function, metabolism can lead to various diseases. Molecular Biology Human beings are highly developed species. How a person looks, behaves, suffers from diseases and the like are dictated by genetic material called DNA. The information is inherited from parent to offspring. The same is replicated from parent DNA to daughter DNA. Individual characters are translated in to proteins, under the direction of DNA. First RNA is synthesized from DNA (Transcription) which is translated in to Proteins. These proteins are responsible for various metabolic functions. Protein expression during development, adaptation, aging and other related processes of life are controlled by DNA. Changes in the genetic material cause hereditary diseases. ix UNIT ONE ENZYMES General Properties Enzymes are protein catalysts for chemical reaction in biological systems. They increase the rate of chemical reactions taking place within living cells with out changing themselves. Nature of Enzymes Most enzymes are protein in nature. Depending on the presence and absence of a non- protein component with the enzyme enzymes can exist as, simple enzyme or holoenzyme 1. Simple enzyme: It is made up of only protein molecules not bound to any non- proteins. Example: Pancreatic Ribonuclease. 2. Holo enzyme is made up o protein groups and non-protein component. The protein component of this holo enzymes is called apoenzyme The non-protein component of the holo enzyme is called a cofactor. I If this cofactor is an organic compound it is called a coenzyme and if it is an inorganic groups it is called activator. (Fe 2+, Mn 2+, or Zn 2+ ions). If the cofactor is bound so tightly to the apoenzyme and is difficult to remove without damaging the enzyme it is sometimes called a prosthetic group COENZYMES- Coenzymes are derivatives of vitamins without which the enzyme cannot exhibit any reaction. One molecule of coenzyme is able to convert a large number of substrate molecules with the help of enzyme. Coenzyme accepts a particular group removed from the substrate or donates a particular group to the substrate Coenzymes are called co substrate because the changes that take place in substrates are complimentary to the changes in coenzymes. 1 The coenzyme may participate in forming an intermediate enzyme-substrate complex Example: NAD, FAD, Coenzyme A Metal ions in enzymes Many enzymes require metal ions like ca2+, K+, Mg2+, Fe2+, Cu2+, Zn2+, Mn2+ and Co2+ for their activity. Metal-activated enzymes-form only loose and easily dissociable complexes with the metal and can easily release the metal without denaturation. Metalloenzymes hold the metal tightly on the molecule and do not release it even during extensive purification. Metal ions promote enzyme action by a. Maintaining or producing the active structural conformation of the enzyme (e.g. glutamine synthase) b. Promoting the formation of the enzyme-substrate complex (Example: Enolase and carboxypeptidase A.) c. Acting as electron donors or acceptors (Example: Fe-S proteins and cytochromes) d. Causing distortions in the substrate or the enzyme Example: phosphotransferases). Properties of Enzyme A. Active site Enzyme molecules contain a special pocket or cleft called the active site. The active site contains amino acid chains that create a three-dimensional surface complementary to the substrate. The active site binds the substrate, forming an enzyme-substrate (ES) complex. ES is converted to enzyme-product (EP); which subsequently dissociates to enzyme and product. For the combination with substrate, each enzyme is said to possess one or more active sites where the substrate can be taken up. The active site of the enzyme may contain free hydroxyl group of serine, phenolic (hydroxyl) group of tyrosine, SH-thiol (Sulfhydryl) group of cysteine or imindazolle group of histidine to interact with there is substrates. 2 It is also possible that the active site (Catalytic site) is different from the binding site in which case they are situated closely together in the enzyme molecule. B. Catalytic efficiency/ Enzyme turnover number Most enzyme- catalyzed reactions are highly efficient proceeding from 103 to 108 times faster than uncatalyzed reactions. Typically each enzyme molecule is capable of transforming 100 to 1000 substrate molecule in to product each second. Enzyme turn over number refers to the amount of substrate converted per unit time (carbonic anhydrase is the fastest enzyme). C. Specificity Enzymes are specific for their substrate. Specificity of enzymes are divided into: a. Absolute specificity:- this means one enzyme catalyzes or acts on only one substrate. For example: Urease catalyzes hydrolysis of urea but not thiourea. b. Stereo specificity- some enzymes are specific to only one isomer even if the compound is one type of molecule: For example: glucose oxidase catalyzes the oxidation of β-D-glucose but not α-D- glucose, and arginase catalyzes the hydrolysis of L-arginine but not D-arginine. *Maltase catalyzes the hydrolysis of α- but not β –glycosides. Bond Specificity * Enzymes that are specific for a bond or linkage such as ester, peptide or glycosidic belong to this group Examples: 1. Esterases- acts on ester bonds 2. Peptidases-acts on peptide bonds 3. Glycosidases- acts on glycosidic bonds. D. Regulation Enzyme activity can be regulated- that is, enzyme can be, activated or inhibited so that the rate of product formation responds to the needs of the cell. 3 E. Zymogens (- inactive form of enzyme) Some enzymes are produced in nature in an inactive form which can be activated when they are required. Such type of enzymes are called Zymogens (Proenzymes). Many of the digestive enzymes and enzymes concerned with blood coagulation are in this group Examples: Pepsinogen - This zymogen is from gastric juice.When required Pepsinogen converts to Pepsin Trypsinogen - This zymogen is found in the pancreatic juice, and when it is required gets converted to trypsin. * The activation is brought about by specific ions or by other enzymes that are proteolytic. Pepsinogen + H+ Pepsin Trypsinogen Enteropeptidase Trypsin Zymogen forms of enzymes a protective mechanism to prevent auto digestion of tissue producing the digestive enzymes and to prevent intravascular coagulation of blood. F. Isoenzymes (Isozymes) These are enzymes having similar catalytic activity, act on the same substrate and produces the same product but originated at different site and exhibiting different physical and chemical characteristics such as electrophoretic mobilities, amino acid composition and immunological behavior. Example: LDH (Lactate dehydrogenase) exists in five different forms each having four polypeptide chains. H= Heart and M=Muscle. Type Polypeptide chain LDH-1 HHHH LDH-2 HHHM LDH-3 HHMM LDH-4 HMMM LDH-5 MMMM 4 Example. CPK (Creatine phospho kinase) exists in three different forms each having two polypeptide chains. Characteristic sub units are B=Brain and M= Muscle. Type Polypeptide chain CPK-1 BB CPK-2 MB CPK-3 M Classification of Enzymes Enzymes are classified on the basis of the reactions they catalyze- Each enzyme is assigned a four-digit classification number and a systematic name, which identifies the reaction catalyzed. The international union of Biochemistry and Molecular Biology developed a system of nomenclature on which enzymes are divided in to six major classes, each with numerous sub groups. Enzymes are classified based on the reactions they catalyze. Each enzyme is characterized by a code number comprising four digits separated by points. The four digits characterize class, sub-class, sub-sub-class, and serial number of a particular enzyme. Class I. Oxidoreducatases- Enzymes catalyzing oxidation reduction reactions. Example: Lactate-dehydrogenase 1- Lactic acid + NAD+ Pyruvic acid + NADH+H+ Class II. Transferases: Enzymes catalyzing a transfer of a group other than hydrogen (methyl, acyl, amino or phosphate groups) Example: Enzymes catalyzing transfer of phosphorus containing groups. ATP: D-hexose-6 phosphotransferase (Hexokinase) ATP+D-Hexose ADP+D-hexose-6-phosphate Example: Acetyl-CoA: Choline D-acyltransferase (Choline Acyltransferase) 5 Class III. Hydrolases: Enzymes catalyzing hydrolysis of ester, ether, peptido, glycosyl, acid-anhydride, C-C, C-halide, or P-N-bonds by utilizing water. Example: Enzymes action on glycosyl compounds β-D- Galactoside galactohydrolase (β -Galactosidase) α β -D- Galactoside+H2O alcohol +D-Galactose Class IV. Lyases: Enzymes that catalyze removal of groups from substances by mechanisms other than hydrolysis, leaving double bonds. Enzymes acting on C-C, C-O, C-N, C-S and C-halide bonds. Example : Carbon-Oxygen lyases Malate hydro-lyase (Fumarase) COOH COOH CHOH CH + H2O + H2O CH2 HC COOH COOH Malate Fumarate Class V. Isomerases: Includes all enzymes catalyzing interconversion of optical, geometric, or positional isomers. Example: Enzymes catalyzing interconversion of aldose and ketoses D - Glyceraldehyde-3- phosphate ketoisomerase (triosephosphate isomerase) D - Glyceraldehyde-3phosphate Dihydroxyacetone phosphate. Class VI. Ligases or synthetases. Enzymes catalyzing the linking together of 2 compounds coupled to the breaking of a pyrophosphate bond in ATP or similar trinucleotides: GTP, UTP etc. included are enzymes catalyzing reactions forming C-O, C-S, C-N, and C-C bonds, 6 Example: Enzymes catalyzing formation of C-N-bonds L- Glutamine: ammonia ligase (ADP) [Glutamine Synthetase] ATP + L-Glutamate + NH4 = ADP + orthophosphate + L-Glutamine Example: Enzymes catalyzing formation of C-C bonds Acetyl-CoA: CO2 ligase (ADP) [acetyl-CoA carboxylase] ATP+ Acetyl-COA-CO2 Malonyl-CoA+ADP+pi. MECHANISM OF ACTION OF ENZYMES Emil Fischer’s model Lock and Key model 1890. Lock: Key model of enzyme action implies that the active site of the enzyme is complementary in shape to that of its substrate, i.e. the shape of the enzyme molecule and the substrate molecule should fit each other like a lock and Key In 1958, Daniel Koshland, postulated another model; which implies that the shapes & the active sites of enzymes are complementary to that of the substrate only after the substrate is bound. Figure: Models of enzyme- substrate interactions Mechanism of Enzyme Action (1913) Michaels and Menten have proposed a hypothesis for enzyme action, which is most acceptable. According to their hypothesis, the enzyme molecule (E) first combines with a substrate molecule (S) to form an enzyme substrate (ES) complex which further 7 dissociates to form product (P) and enzyme (E) back. Enzyme once dissociated from the complex is free to combine with another molecule of substrate and form product in a similar way. ENZYMES ENHANCE THE RATE OF REACTION BY LOWERING FREE ENERGY OF ACTIVAION A chemical reaction S P (where S is the substrate and P is the product or products) will take place when a certain number of S molecules at any given instant posses enough energy to attain an activated condition called the “transition state”, in which the probability of making or breaking a chemical bond to form the product is very high. The transition state is the top of the energy barrier separating the reactants and products. The rate of a given reaction will vary directly as the number of reactant molecules in the transition state. The “energy of activation is the amount of energy required to bring all the molecules in 1 gram-mole of a substrate at a given temperate to the transition state A rise in temperature, by increasing thermal motion and energy, causes an increase in the number of molecules on the transition state and thus accelerates a chemical reaction. Addition of an enzyme or any catalyst can also bring about such acceleration. The enzyme combines transiently with the substrate to produce a transient state having c lower energy of activation than that of substrate alone. This results in acceleration of the reaction. Once the products are formed, the enzyme (or catalyst) is free or regenerated to combine with another molecule of the substrate and repeat the process. Activation energy is defined as the energy required to convert all molecules in one mole of reacting substance from the ground state to the transition state. Enzyme are said to reduce the magnitude of this activation energy. * During the formation of an ES complex, the substrate attaches itself to the specific active sites on the enzyme molecule by Reversible interactions formed by Electrostatic bonds, Hydrogen bonds, Vanderwaals forces, Hydrophobic interactions. 8 Factors Affecting Enzyme Activity Physical and chemical factors are affecting the enzyme activity. These include 1. Temperature 2. pH 3. Substrate/enzyme concentration etc. Temperature Starting from low temperature as the temperature increases to certain degree the activity of the enzyme increases because the temperature increase the total energy of the chemical system. There is an optimal temperature at which the reaction is most rapid (maximum). Above this the reaction rate decreases sharply, mainly due to denaturation of the enzyme by heat. The temperature at which an enzyme shows maximum activity is known as the optimum temperature for the enzyme. For most body enzymes the optimum temperature is around 370c, which is body temperature. Enzyme activity Optimum temperature 10 20 30 40 50 Temperature Figure. Effect of temperature on enzymatic reaction 1. Effect of pH The concentration of H+ affects reaction velocity in several ways. First, the catalytic process usually requires that the enzyme and substrate have specific chemical groups in an ionized or unionized sate in order to interact. 9 For example, Catalytic activity may require that an amino-group of the enzyme be in the protonated form (-NH3+) At alkaline pH this group is deprotonated and the rate of reaction therefore declines. Extreme pH can also lead to denaturation of the enzyme, because the structure of the catalytically active protein molecule depends on the ionic character of the amino acid chains. The pH at which maximum enzyme activity is achieved is different for different enzymes, and after reflects the pH+] at which the enzyme functions in the body. For example, pepsin, a digestive enzyme in the stomach, has maximum action at pH 2, where as other enzymes, designed to work at neutral pH, are denatured by such an acidic environment. Enzyme activity Optimum pH 6 8 PH Figure. Effect of pH on enzymatic reaction 3. Concentration of substrate At fixed enzyme concentration pH and temperature the activity of enzymes is influenced by increase in substrate concentration. An increase in the substrate concentration increases the enzyme activity till a maximum is reached. Further increase in substrate concentration does not increase rate of reaction. This condition shows that as concentration of substrate is increased, the substrate molecule combine with all available enzyme molecules at their active site till not more active sites are available (The active Sites become saturated). At this state the enzyme is obtained it maximum rate (V max). 10 Figure. Effect of Concentration of substrate on enzyme activity The characteristic shape of the substrate saturation curve for an enzyme can be expressed mathematically by the Michaelis Menten equation: K1 K2 E +S ES E +P Km = K3 +K2 / K1 K-1 V max[S ] V = Km + [ S ] Where: V= Velocity at a given concentration of substrate (initial reaction velocity) Vmax = Maximal velocity possible with excess of substrate [S] = concentration of the substrate at velocity V Km = michaelis-constant of the enzyme for particular substrate. Relationship between [S] and Km Km shows the relationship between the substrate concentration and the velocity of the enzyme catalyzed reaction. Take the point in which 50% of the active site of the enzyme will be saturated by substrate, Assume that at ½ Vmax-50% of the active site of enzyme becomes saturated. Therefore: 11 Vo = ½ Vmax, at 50% saturation ½ Vmax = Vmax[S] Km + [S] 2[S] = Km + [S] Km= [S] Figure: Relationship between [S] and Km Characteristics of Km Km- can defined as the concentration of the substrate at which a given enzyme yields one-half its max. Velocity (i.e Km is numerically equal to the substrate concentration of which the reaction velocity equal to ½ Vmax) Km- is characteristic of n enzyme and a particular substrate, and reflects the affinity of the enzyme for that substrate. Km- values varies from enzyme to enzyme and used to characterized different enzymes. Km- values of an enzyme helps to understand the nature and speed of the enzyme catalysis. Small Km - A numerically small (Low) km reflects a high affinity of the enzyme for substrate because a low conc of substrate is needed to half saturate the enzyme- that is reach a velocity of ½ Vmax. High Km - A numerically large (high) Km reflects a low affinity of enzyme for substrate b/c a high conc of substrate is needed to half saturate the enzyme. 12 High Km Value f an enzyme means the catalysis of that enzyme is slow compared to low Km. Km does not vary with the concentration of enzyme. 4. Relationship of Velocity to Enzyme Concentration The rate of the reaction is directly proportional to enzyme concentration at all substrate concentration. For example, if the enzyme concentration halved, the initial rate of the reaction (Vo) is reduced to one half that of the original. Enzyme activity Enzyme concentration Figure. Effect of Enzyme concentration on enzymatic reaction Order of Reaction When [S] is much less than Km, the velocity of the reaction is roughly proportional to the substrate concentration. The rate of reaction is then said to be first order configuration with respect to substrate. When [S] is much greater than Km, the velocity is constant and equal to V max. The rate of reaction is then independent of substrate concentration and said to be zero order with respect to substrate concentration. Enzyme Inhibition Any substance that can diminish the velocity of an enzyme-catalyzed reaction is called an inhibitor and the process is known as inhibition. There are two major types of enzyme inhibition, Irreversible and Reversible. 13 Irreversible Inhibition The type of inhibition that can not be reversed by increasing substrate concentration or removing the remaining free inhibitor is called Irreversible inhibition Eg. Diisopropyl & luorophosphate (DFP) Inhibits the enzyme acetyl cholinesterase, important in the transmission of nerve impulses. Acetyl cholinesterase catalyzes the hydrolysis of Acetylcholin (to acetic acid and choline) a neurotransmitter substance functioning in certain portions of the nervous system DEP inhibits also trypsin, chymotrypsin elastase, and phosphglucomutase Organo-phosphorus compounds like malathion, parathron pesticides-inhibits acetyl cholinesterase by the same way as DFP. Example: Inhibition of triose phosphate dehydrogenate by iodo acetate which block the activity of the enzyme. REVERSIBLE INHIBITION This type of inhibition can be Competitive, Non-competitive and uncompetitive Competitive Inhibition: This type of inhibition occurs when the inhibitor binds reversibly to the same site that the substrate would normally occupy, therefore, competes with the substrate for that site. In competitive inhibition the inhibitor and substrate compete for the same active site on the enzyme as a result of similarity in structure. The enzyme substrate complex will be broken dawn to products (E+S'ESE+P) where as enzyme inhibitor complex; (EI) will not be broken down to products. A classical example is Malonate that competes with succinate and inhibits the action of succinate dehydrogenase to produce fumarate in the Krebs cycle. The enzyme can be also inhibited by oxalate and glutarate because of the similarity of this substance with succinate Eg.2 Allopurinol used for the treatment of Gout Allopurinol Inhibits Xanthine oxidase by competing with Uric acid precursors for the active site on the enzyme. This competition blocks the conversion of these precursors, and of hypoxanthine and xanthine, to uric acid and result in lower serum urate levels. 14 Inhibition of Enzyme Catalyzed Reactions To avoid dealing with curvilinear plots of enzyme catalyzed reactions, biochemists Lineweaver and Burk introduced an analysis of enzyme kinetics based on the following rearrangement of the Michaelis-Menten equation: [1/v] = [Km (1)/ Vmax[S] + (1)/Vmax] Plots of 1/v versus 1/[S] yield straight lines having a slope of Km/Vmax and an intercept on the ordinate at 1/Vmax. A Lineweaver-Burk Plot An alternative linear transformation of the Michaelis-Menten equation is the Eadie-Hofstee transformation: v/[S] = -v [1/Km] + [Vmax/Km] and when v/[S] is plotted on the y-axis versus v on the x-axis, the result is a linear plot with a slope of -1/Km and the value Vmax/Km as the intercept on the y- axis and Vmax as the intercept on the x-axis. Both the Lineweaver-Burk and Eadie-Hofstee transformation of the Michaelis-Menton equation are useful in the analysis of enzyme inhibition. Since most clinical drug therapy is based on inhibiting the activity of enzymes, analysis of enzyme reactions using the tools described above has been fundamental to the modern design of pharmaceuticals 15 Effect of Competitive inhibitors 1. Effect on Vmax: The effect of a competitive inhibitor is reversed by increasing [s]. at a sufficiently high substrate concentration, the reaction velocity reaches the Vmax. observed in the absence of inhibitor. 2. Effect on Km: A competitive inhibitor increases the apparent Km for a given substrate. This means that in the presence of a competitive inhibitor more substrate is needed to achieve ½ Vmax. Figure: Competitive inhibition Non-Competitive Inhibition In non-competitive inhibition the inhibitor binds at different site rather than the substrate-binding site. When the inhibitor binds at this site there will be a change in conformation of the enzyme molecules, which leads to the reversible inactivation of the catalytic site. Non-competitive inhibitors bind reversibly either to the free-enzyme or the ES complex to form the inactive complexes EI and ESI (Enzyme substrate Inhibition) The most important non-competitive inhibitors are naturally occurring metabolic intermediates that can combine reversibly with specific sites on certain regulatory enzymes, that changes the activity of their catalytic sites. An Example: is the inhibition of L-threonine dehydratase by L-isoleucine. *Such type of Enzyme is called Allosteric Enzyme, which has a specific sites or allosteric site other than the substrate-binding site. 16 1. Effect on Vmax. Non-Competitive inhibition cannot be overcome by increasing the concentration of substrate. Thus, non-competitive inhibitors decrease the Vmas of the reaction. 2. Effect on Km: Non-competitive inhibitors do not interfere with the binding of substrate to enzyme. Thus, the enzyme shows the same Km in the presence or absence of the non- competitive inhibitor. Figure: Noncompetitive inhibition Uncompetitive Inhibition Uncompetitive Inhibitor binds only to ES complex at locations other than the catalytic site. Substrate binding modifies enzyme structure, making inhibitor-binding site available. Inhibition cannot be reversed by substrate. In this case apparent Vmax. and Km decreased. Figure: Uncompetitive inhibition 17 Regulation of enzyme activity There are several means by which the activity of a particular enzyme is specifically regulated. 1. Irreversible covalent Activation / Zymogen activation Some enzymes are secreted in an inactive form called Proenzymes or zymogens. At the site of action specific peptide bonds are hydrolysed either enzymatically or by PH changes to convert it into active form, e.g. Pepsinogen Æ pepsin, TrypsinogenÆ trypsin, plasminogenÆ plasmin. After hydrolysis when it is activated, it cannot be reconverted into proenzyme form. 2. Reversible Covalent Modification By addition of or removal of phosphate or adenylate, certain enzymes are reversibly activated and inactivated as per the requirement. Protein kinase of muscle phosphorylate phosphorylase kinase, glycogen synthetase by making use of ATP. 3. Allosteric Modulation In addition to simple enzymes that interact only with substrates and inhibitors, there is a class of enzymes that bind small, physiologically important molecules and modulate activity in ways other than those described above. These are known as allosteric enzymes; the small regulatory molecules to which they bind are known as effectors. Allosteric effectors bring about catalytic modification by binding to the enzyme at distinct allosteric sites, well removed from the catalytic site, and causing conformational changes that are transmitted through the bulk of the protein to the catalytically active site(s). The hallmark of effectors is that when they bind to enzymes, they alter the catalytic properties of an enzyme's active site. Those that increase catalytic activity are known as positive effectors. Effectors that reduce or inhibit catalytic activity are negative effectors. 18 There are two ways that enzymatic activity can be altered by effectors: the Vmax can be increased or decreased, or the Km can be raised or lowered. 4. Feedback inhibition In allosteric regulation in which end products inhibit the activity of the enzyme is called” feedback inhibition”. A high conc. D typically inhibits conversion of AÆ B. This involves not simple backing up of intermediates but the activity of D to bind to and inhibit E1. D thus acts as negative allosteric affector or feedback inhibitor of E1. The kinetics of feedback inhibition cay be competitive, mixed, etc. It is the commonest way of regulation of a biosynthetic pathway. Feedback regulation generally occurs at the earliest functionally irreversible step unique in the biosynthetic pathway. ENZYMES IN CLINICAL DIAGNOSIS Plasma enzymes can be classified into two major groups 1. Those, relatively, small group of enzymes secreted into the plasma by certain organs (i.e. Enzymes those have function in plasma) For example: - the liver secretes zymogens of the enzymes involved in blood coagulation. 2. Those large enzyme species released from cells during normal cell turnover. These enzymes are normally intracellular and have no physiologic function in the plasma. In healthy individuals the levels of these enzymes are fairly constant and represent steady state in which the rate of release from cells into the plasma is balanced by an equal rate or removal from the plasma. Many diseases that cause tissue damage result in an increased release of intracellular enzymes into the plasma. The activities of many of these enzymes are routinely 19 determined for diagnostic purposes in diseases of the heart, liver, skeletal muscle, and other tissues. The level of specific enzyme activity in the plasma frequently correlates with the extent of tissue damage. Thus, the degree of elevation of a particular enzyme activity in plasma is often useful in evaluating the diagnosis and prognosis for the patient. Measurement of enzymes concentration of mostly the latter type in plasma gives valuable informatio0n about disease involving tissues of their origin. 1. Lipase: It is an enzyme catalyzing the hydrolysis of fats. It is secreted by pancreas and Liver. The plasma lipase level may be low in liver disease, Vitamin A deficiency, some malignancies, and diabetes mellitus. It may be elevated in acute pancreatitis and pancreatic carcinoma. 2. α- Amylase α- amylase is the enzyme concerned with the break down of dietary starch and glycogen to maltose. It is present in pancreatic juice and saliva as well as in liver fallopian tubes and muscles. The enzyme is excreted in the Urine. The main use of amylase estimations is in the diagnosis of acute pancreatitis. The plasma amylase level may be low in liver disease and increased in high intestinal obstruction, mumps, acute pancreatitis and diabetes. 3. Trypsin Trypsin is secreted by pancreas. Elevated levels of trypsin in plasma occur during acute pancreatic disease. 4. Alkaline phosphates (ALP) The alkaline phosphates are a group of enzymes, which hydrolyze phosphate esters at an alkaline pH. They are found in bone, liver, kidney, intestinal wall, lactating mammary gland and placenta. In bone the enzyme is found in osteoblasts and is probably 20 important for normal bone function. The level of these enzymes may be increased in rickets and osteomalacia, hyperparathyroidism, paget's disease of bone, obstructive jaundice, and metastatic carcinoma. Serum alkaline phosphatase levels may be increase in congestive heart failure result of injury to the liver. 5. Acid Phosphatase (ACP) Acid phosphatases catalyzing the hydrolysis of various phosphate esters at acidic pH is found in the prostate, liver, red cells, platelets and bone. It may be elevated in metastatic prostatic carcinoma. 6. Transaminases Two transaminases are of clinical interest. 1. Aspartate Transaminase, AST ( Glutamate oxaloacetate transaminase, GOT ) catalyzes the transfer of the amino group of aspartic acid to α- ketoglutarate forming glutamate and oxaloacetate. AST or GOT is widely distributed, with high concentration, in the heart, liver, skeletal muscle, kidney and erythrocytes, and damage to any of these tissues may cause raised levels. 2. Alanine transaminase, ALT (Glutamate pyruvate transaminase, GPT ) Transfer the amino group of alanine to α- ketoglutarate, forming glutamate and pyruvate. It is present in high concentration in liver and to a lesser extent in skeletal muscle, kidney and heart. - Serum levels of glutamate- pyruvate transaminase (SGOT) and Glutamate- oxaloacetate- transaminase (SGOT) are useful in the diagnosis of liver parenchymal damage and myocardial damage respectively. In liver damage, both enzymes are increased, but SGPT increases more. In myocardial infarction SGOT is increased with little or no increase in SGPT. 21 7. Lactate Dehydrogenase (LDH) It catalyzes the reversible interconversion of lactate and pyruvate. It is widely distributed with high concentrations in the heart, skeletal muscle, liver, kidney, brain and erythrocytes. The enzyme is increased in plasma in myocardial infarction, acute leukemias, generalized carcinomatosis and in acute hepatitis. Estimation of it isoenzymes is more useful in clinical diagnosis to differentiate hepatic disease and myocardial infarction. 8. Creatine kinase (CK) or ceratin phosphokinase (CPK) CK (CPK) is found in heart muscle brain and skeletal muscle. Measurement of serum creatine phosphokinase activity is of value in the diagnosis of disorders affecting skeletal and cardiac muscle. The level of CPK in plasma highly increased in myocardial infarction. 22 UNIT TWO CARBOHYDRATS Objectives Define carbohydrates in chemical terms Classify carbohydrates in to three major groups with examples of each group List the monosaccharides of biological importance and learn their properties List the disaccharides of biological importance and learn their properties List the polysaccharides of biological importance and learn their properties Study the chemistry and functions of glycoproteins Introduction Carbohydrates are the most abundant macromolecules in nature. They are the main source and storage of energy in the body. They serve also as structural component of cell membrane. The general molecular formula of carbohydrate is CnH2nOn or (CH2O)n, where n > 3. Chemically, they contain the elements Carbon, hydrogen and oxygen. Thus they are Carbon compounds that contain large quantities of Hydroxyl groups. Carbohydrates in general are polyhydroxy aldehydes or ketones or compounds which give these substances on hydrolysis. Chemistry of Carbohydrates Classification and Structure Classification There are three major classes of carbohydrates Monosaccharides (Greek, mono = one) Oligosaccharides (Greek, oligo= few) 2-10 monosaccharide units. Polysaccharides (Greek, Poly = many) >10 monosaccharide units. Monosaccharides Monosaccharides also called simple sugars. They consist of a single polyhydroxy aldehyde or ketone units. The most abundant monosaccharides in nature are the 6-carbon sugars like D- glucose and fructose. 23 Structure Monosaccharide has a backbone, which is un- branched, single bonded carbon chain. One of the carbon atoms is double bonded to an oxygen atom to form carbonyl group. Each of the other carbon atoms has a hydroxyl group. Example. Structure of Glucose Open chain D-glucose α-D –glucose α-D –glucose (Fisher formula) (Haworth formula) Fig.2.1. structures of D.Glucose There are two families of monosaccharides. Monosaccharides having aldehyde groups are called Aldoses and monosaccharides with Ketone group are Ketoses. Depending on the number of carbon atoms, the monosaccharides are named trioses (C3), tetroses (C4), pentoses (C5), hexoses (C6), heptoses (C7). No of carbon atoms Generic name Aldose family Ketose family 3 Triose Aldotriose Ketotriose Eg.Glyceraldehyde. Eg. Dihydroxyacetone 4 Tetrose Aldotetrose Ketotetrose Eg. Erythrose Eg. Eyrthrulose 5 Pentose Aldopentose Ketopentose Eg. Ribose Eg. Ribulose,Xylulose 6 Hexose Aldohexose Ketohexose Eg. Glucose Eg. Fructose Galactose Mannose Table 1.1 Common Biologically important monosaccharides with their families. 24 Physical properties Physical properties of Monosaccharides They are colorless, crystalline compounds, readily soluble in water. Their solutions are optically active and exhibit the phenomenon of mutarotation. Carbohydrates spontaneously change between the α and β configuration. Asymmetric Center and Stereoisomerism Asymmetric carbon is a carbon that has four different groups or atoms attached to it and having optically activity in solution. All the monosaccharides except dihydroxyacetone contain one or more asymmetric or chiral carbon atoms and thus occur in optically active isomeric forms. Monosaccharides with n number of asymmetric centers will have (2n) isomeric forms. (n= number of asymmetric carbon atoms). Fig 2.2: The two isomeric forms of glyceraldehyde. The designation of a sugar isomer as the D- form or of its mirror images the L- form is determined by the spatial relationship to the parent compound of the carbohydrate family. The D and L forms of Glyceraldehyde are shown in the Figure 2.2.The orientation of- OH and- H groups around the carbon atom adjacent to the terminal primary alcohol carbon determines its D or L form.When the - OH group on this carbon is on the right, the sugar is a member of the D- series, when it is on the left, it is a member of the L-series. These D and L configuration are also called Enantiomers. Optical Activity The presence of asymmetric carbon atom causes optical activity. When a beam of plane- polarized light is passed through a solution of carbohydrate it will rotate the light either to right or to left. Depending on the rotation, molecules are called dextrorotatory (+) (d) or levorotatory (-) (l). Thus, D- glucose is dextrorotatory but D- fructose is levorotatory. When equal amounts of D 25 and L isomers are present, the resulting mixture has no optical activity, since the activities of each isomer cancel one another. Such a mixture is called racemic or DL mixture. Epimers When sugars are different from one another, only in configuration with regard to a single carbon atom (around one carbon atom) they are called epimers of each other. For example glucose and mannose are epimers. They differ only in configuration around C2. Mannose and Galactose are epimers of Glucose Fig 2.3: Structure of D - glucose, D-mannose and D- Galactose. Anomers The two stereoisomers at the hemiacetal (anomeric) carbon are: oThe alpha anomer: Where- OH group is down (Haworth) oThe beta anomer:Where- OH group is up (Haworth) Anomers are diastereomers (having different physical properties) Cyclization of monosaccharides Monosaccharides with five or more carbon atoms in the backbone usually occur in solution as cyclic or ring structure, in which the carbonyl group is not free as written on the open chain structure but has formed a covalent bond with one of the hydroxyl group along the chain to form a hemiacetal or hemiketal ring. In general, an aldehyde can react with an alcohol to form a hemiacetal or acetal. 26 The C-1 aldehyde in the open-chain form of glucose reacts with the -5th carbon atom containing hydroxyl group to form an intramolecular hemiacetal. The resulting six membered ring is called pyranose because of its similarity to organic molecule Pyran. Two different forms of glucose are formed when the OH group extends to right it is α-D-Glucose and when it extends to left, it is β-D-Glucose commonly called as Anomers. Similarly, a ketone can react with an alcohol to form a hemiketal or ketal. The C-2 keto group in the open chain form of fructose can react with the 5th carbon atom containing hydroxyl group to form an intramolecular hemiketal. This five membered ring is called furanose because of its similarity to organic molecule furan Fig 2.4. α and β forms of Fructose Oligosaccharides Oligosaccharides contain 2 to 10 monosaccharide units. The most abundant oligosaccharides found in nature are the Disaccharides. 27 Disaccharides When two monosaccharides are covalently bonded together by glycosidic linkages a disaccharide is formed. Glycosidic bond is formed when the hydroxyl group on one of the sugars reacts with the anomeric carbon on the second sugar. Biologically important disaccharides are sucrose, maltose, and Lactose. Maltose Maltose contains two D glucose residues joined by a glycosidic linkage between OH at the first carbon atom of the first glucose residues and OH at the fourth carbon atom of the second glucose forming a α-(1,4) glycosidic linkage as shown in Figure below. Maltose is the major degradative product of Starch.Maltose is hydrolyzed to two molecules of D- glucose by the intestinal enzyme maltase, which is specific for the α- (1, 4) glycosidic bond. Fig 2.5. Structure of Maltose Lactose Lactose is a disaccharide of β-D galactose and β-D- glucose which are linked by β-(1,4) glycosidic linkage. Lactose acts as a reducing substance since it has a free carbonyl group on the glucose. It is found exclusively in milk of mammals (Milk sugar). Figure2.6: Structure of Lactose 28 Sucrose (Cane sugar) Sucrose is a disaccharide of α- D- glucose and β-D-fructose. It is obtained from cane sugar. It is also present in various fruits. In contrast to other disaccharides sucrose contains no free anomeric carbon atom. Since the anomeric carbons of both its component monosaccharide units are linked to each other. For this reason sucrose is non reducing sugar. Fig 2.7. Structure of sucrose α-(1, 2) β-Glycosidic bond Polysaccharides Most of the carbohydrates found in nature occur in the form of high molecular polymers called polysaccharides. There are two types of polysaccharides.These are: Homopolysaccharides that contain only one type of monosaccharide building blocks. Heteropolysaccharides, which contain two or more different kinds monosaccharide building blocks. Homopolysaccharides Example of Homopolysaccharides: Starch, glycogen, Cellulose and dextrins. Starch It is one of the most important storage polysaccharide in plant cells.It is especially abundant in tubers, such as potatoes and in seeds such as cereals. Starch consists of two polymeric units made of glucose called Amylose and Amylopectin but they differ in molecular architecture. Amylose is unbranched with 250 to 300 D-Glucose units linked by α-(1, 4) linkages Amylopectin consists of long branched glucose residue (units) with higher molecular weight. 29 The inner part of glucose units in amylopectin are joined by α-(1,4) glycosidic linkage as in amylose , but the branch points of amylopectin are α- (1,6) linkages. The branch points repeat about every 20 to 30 (1-4) linkages Glycogen - Glycogen is the main storage polysaccharide of animal cells (Animal starch). - It is present in liver and in skeletal muscle. - Like amylopectin glycogen is a branched polysaccharide of D-glucose units in α - (1, 4) linkages, but it is highly branched. - The branches are formed by α -(1,6) glycosidic linkage that occurs after every 8 -12 residues. Therefore liver cell can store glycogen within a small space. Multiple terminals of branch points release many glucose units in short time. Cellulose Cellulose is the most abundant structural polysaccharide in plants. It is fibrous, tough, water insoluble. Cellulose is a linear unbranched homopolysaccharide of 10,000 or more D- glucose units connected by β-(1, 4) glycosidic bonds. Humans cannot use cellulose because they lack of enzyme (cellulase) to hydrolyze the β-( 1-4) linkages. Figure: Structure of Cellulose 30 Dextrins These are highly branched homopolymers of glucose units with α-(1, 6), α-(1, 4) and α-(1, 3) linkages. Since they do not easily go out of vascular compartment they are used for intravenous infusion as plasma volume expander in the treatment of hypovolumic shock. Hetero polysaccharides These are polysaccharides containing more than one type of sugar residues 1. Glycosaminoglycans, (GAGs or mucopolysaccharides) They are long, usually unbranched, composed of a repeating disaccharide units * They are negatively charged heteroplolysaccharid chains (polyanions) The amino sugar is either D-glucosamine or D-galactosamine in which the amino group is usually acetylated, thus eliminating its positive charges. The amino sugar may also be sulfated on carbon 4, 6, or on a monoacetylated nitrogen. The acidic sugar is either D-glucuronic acid or its carbon 6 epimer, L-uronic acid. For example Hayluronic acid, Heparin and chondatin sulphate. Function of Glycosammoglycans. (GAGS) 1. They have the special ability to bind large amounts of water, there by producing the gel-like matrix that forms the basis of the body’s ground substance. 2. Since they are negatively charged, for example, in bone, glycosaminoglycans attract and tightly bind cattions like ca++, they also take-up Na+and K+ 3. GAGs stabilize and support cellular and fibrous components of tissue while helping maintain the water and salt balance of the body. 4. Its essential components of the extra cellular matrix, GAGs’ play an important role in mediating cell-cell interactions Ground substance is a part of connective tissue, which is a gel like substance containing water, salt, proteins and polysaccharides. An example of specialized ground substance is the synovial fluid, which serves as a lubricant in joints, and tendon sheaths. 31 3. Heparin: contains a repeating unit of D-glucuronic and D-gluconsamine, with sulfate groups on some of the hydroxyl and aminx-groups It is an important anticoagualtn, prevents the clotting of blood by inhiginting the conversion of prothrombin to throbin. Thrombin is an enzyme that acts on the conversion of plasma fibrinogen into the fibrin. It is found in mast cells in lung, liver skin and intestinal mucosa. Glycoproteins (Mucoproteins) Glycoprotiens are proteins to which oligosaccharides are covalently attached. They differ from the glycosaminoglycans in that the length of the glycoproteins carbohydrate chain is relatively short (usually two to ten sugar residues in length, although they can be longer), whereas it can be very long in the glycosaminoglycans. The glycoprotein carbohydrate chains are often branched instead of linear and may or may not be negatively charged. For example: - Glycophorin, a glycoprotein found in human red cell membranes. - Human gastric glycoprotein (mucin). - Many protein hormones,receptors are glycoproteins Proteoglycans When glycosamnoglycans are attached to a protein molecule the compound is called proteoglycan [proteoglycans = Glycosaminoglycans + proteins] 32 METABOLISM OF CARBOHYDRATES Objectives study utilization of glucose and other carbohydrates in the body study the various mechanisms and fate of glucose in the body study the energetics of the various mechanisms Digestion of Carbohydrates Dietary carbohydrates principally consist of the polysaccharides: starch and glycogen. It also contains disaccharides: sucrose, lactose, maltose and in small amounts monosaccharides like fructose and pentoses. Liquid food materials like milk, soup, fruit juice escape digestion in mouth as they are swallowed, but solid foodstuffs are masticated thoroughly before they are swallowed. 1. Digestion in Mouth Digestion of carbohydrates starts at the mouth, where they come in contact with saliva during mastication. Saliva contains a carbohydrate splitting enzyme called salivary amylase (ptyalin). Action of ptyalin (salivary amylase) It is α - amylase, requires Cl- ion for activation and optimum pH 6-7. The enzyme hydrolyzes α- (1,4) glycosidic linkage at random, from molecules like starch, glycogen and dextrins, producing smaller molecules maltose, glucose and disaccharides maltotriose. Ptyalin action stops in stomach when pH falls to 3.0 α -Amylase Glucose, Maltose Starch or glycogen And Maltotriose 2. Digestion in Stomach No carbohydrate splitting enzymes are available in gastric juice. HCl may hydrolyze some dietary sucrose to equal amounts of glucose and fructose. 3. Digestion in Duodenum Food reaches the duodenum from stomach where it meets the pancreatic juice. Pancreatic juice contains a carbohydrate-splitting enzyme pancreatic amylase. 33 Action of pancreatic Amylase It is also an α - amylase, optimum pH 7.1. Like ptyalin it also requires Cl- for activity. The enzyme hydrolyzes α-(1,4) glycosidic linkage situated well inside polysaccharide molecule. Other criteria and end products of action are similar of ptyalin. 1. Digestion in Small Intestine Action of Intestinal Juice a. pancreatic amylase: It hydrolyzes terminal α-(1,4), glycosidic linkage in polysaccharides and Oligosaccharide molecules liberating free glucose molecules. b. Lactase It is a β- glycosidase, its pH range is 5.4 to 6.0. Lactose is hydrolyzed to glucose and galactose. lactase Lactose Glucose + Galactose Lactose Intolerance Lactose is hydrolyzed to galactose and glucose by lactase in humans (by β- Galactosidase in Bacteria).Some adults do not have lactase.Such adults cannot digest the sugar.It remains in the intestines and gets fermented by the bacteria. The condition is called as Lactose intolerance. Such patients suffer from watery diarrhea, abnormal intestinal flow and chloeic pain. They are advised to avoid the consumption of Lactose containing foods like Milk. C. Maltase The enzyme hydrolyzes the α -(1,4) glycosidic linkage between glucose units in maltose molecule liberating two glucose molecules. Its pH range is 5.8 to 6.2. Maltase Maltose Glucose + Glucose D. Sucrase PH ranges 5.0 to 7.0. It hydrolyzes sucrose molecule to form glucose and fructose. Sucrase Sucrose Glucose + fructose 34 Absorption of Carbohydrates Products of digestion of dietary carbohydrates are practically completely absorbed almost entirely from the small intestine. Absorption from proximal jejunum is three times grater than that of distal ileum. It is also proved that some disaccharides, which escape digestion, may enter the cells of the intestinal lumen by “pinocytosis” and are hydrolyzed within these cells. No carbohydrates higher than the monosaccharides can be absorbed directly in to the blood stream. Mechanism of Absorption Two mechanisms are involved: 1. Simple Diffusion This is dependent on sugar concentration gradients between the intestinal lumen. Mucosal cells and blood plasma. All the monosaccharides are probably absorbed to some extent by simple ‘passive’ diffusion. 2. “Active “Transport Mechanisms Glucose and galactose are absorbed very rapidly and hence it has been suggested that they are absorbed actively and it requires energy. Fructose absorption is also rapid but not so much as compared to glucose and galactose but it is definitely faster than pentoses. Hence fructose is not absorbed by simple diffusion alone and it is suggested that some mechanism facilitates its transport, called as” facilitated transport”. GLYCOLYSIS Oxidation of glucose or glycogen to pyruvate and lactate is called glycolysis. This was described by Embeden, Meyerhoff and Parnas. Hence it is also called as Embden Meyerhoff pathway. It occurs virtually in all tissues. Erythrocytes and nervous tissues derive its energy mainly form glycolysis. This pathway is unique in the sense that it can utilize O2 if available (‘aerobic’) and it can function in absence of O2 also (‘anaerobic’) 35 Fig 2.8: Glycolysis reactions 36 Aerobic Phase Aerobic phase includes the conversion of glucose to pyruvate Oxidation is carried out by dehydrogenation and reducing equivalent is transferred to NAD. NADH + H+ in presence of O2 is oxidized in electron- transport chain producing ATP. Anaerobic Phase This phase includes the conversion of Glucose to lactate NADH cannot be oxidized, so no ATP is produced in electron transport chain. But the NADH is oxidized to NAD+ by conversion of pyruvate to Lactate, without producing ATP. Anaerobic phase limits the amount of energy per molecule of glucose oxidized. Hence, to provide a given amount of energy, more glucose must undergo glycolysis under anaerobic as compared to aerobic. A. Enzymes Enzymes involved in glycolysis are present in cytoplasm. SIGNIFICANCE OF THE PATHWAY: This pathway is meant for provision of energy. It has importance in skeletal muscle as glycolysis provides ATP even in absence of O2, muscles can survive under anaerobic condition. REACTIONS OF GLYCOLYTIC PATHWAY Series of reactions of glycolytic pathway, which degrades glucose/ glycogen to pyruvate/lactate, are discussed below. For discussion and proper understanding, the various reactions can be arbitrarily divided in to four stages. Stage I This is preparatory stage, before the glucose molecule can be split; the rather asymmetric glucose molecule is converted to almost symmetrical form fructose 1, 6 bisphosphate in the presence of ATP. 1. Uptake of Glucose by Cells and its phosphorylation Glucose is freely permeable to Liver cells. In Intestinal mucosa and kidney tubules, glucose is taken up by ‘active’ transport. In other tissues, like skeletal muscle, cardiac muscle, diaphragm, adipose tissue etc. Insulin facilitates the uptake of glucose. Glucose is then phosphorylated to form glucose – 6- Phosphate. The reaction is catalyzed by the specific enzyme glucokinase in liver cells and by nonspecific Hexokinase in liver and extrahepatic tissues. 37 ATP acts as PO4 donor in the presence of Mg.One high energy PO4 bond is utilized and ADP is produced. The reaction is accompanied by considerable loss of free energy as heat, and hence under physiological conditions is regarded as irreversible. Glucose 6 phosphate formed is an important compound at the junction of several metabolic pathways like glycolysis, glycogenesis, glycogenolysis, glyconeogenesis, Hexosemonophosphate Shunt, uronic acid partway. Thus is a “committed step” in metabolic pathways. 2. Conversion of G- 6- phosphate to Fructose6-phosphate Glucose6 phosphate after formation is converted to fructose 6-p by phospho- hexose isomerase, which involves an aldose- ketose isomerization. The enzyme can act only on α - anomer of Glucose 6 phosphate. Phospho- hexose isomerase Glucose 6 phosphate Fructose 6 phosphate 3. Conversion of Fructose 6phosphate to Fructose 1, 6 bisphosphate The above reaction is followed by another phosphorylation. Fructose-6-p is phosphorylated with ATP at 1- position catalyzed by the enzyme phospho- fructokinase-1 to produce the symmetrical molecule fructose –1, 6 bis phosphate. Note: reaction one is irreversible One ATP is utilized for phosphorylation of glucose at position 6 Phosphofruvctokinase I is the key enzyme in glycolysis that regulates the pathway. The enzyme is inducible, as well as allosterically modified Phosphofructokinase II is an is enzyme which catalyzes the reaction to form fructose-2 6- bis phosphate. Fructose-6-phosphate + ATP Fructose-2, 6-bisphosphate + ADP Energetics Note that in this stage glucose oxidation does not yield any useful energy rather there is expenditure of 2 ATP molecules for two phosphorylations (-2 ATP). 38 Stage II Here,Fructose, 1, 6- bisphosphate is split by the enzyme aldolase into two molecules of triose- phosphates, an Aldotriose, glyceraldehyde3 phosphate and a Ketotriose, Dihydroxy acetone phosphate. Note The reaction is reversible There is neither expenditure of energy nor formation ATP Aldolases are tetramers, containing 4 subunits. Two isoenzymes.A,B Aldolase B: occurs in liver and kidney The fructose- 6-p exists in the cells in “furanose” form but they react with isomerase, phosphofructokinase-1 and aldolase in the open-chain configuration. Both triose phosphates are interconvertable Phosphotriose isomerase Dihydroxy acetone-p D- glyceradehyde-3 –p Stage III This is the energy- yielding reaction. Reactions of this type in which an aldehyde group is oxidized to an acid are accompanied by liberation of large amounts of potentially useful energy. This stage consists of the following two reactions: 1. Oxidation of Glyceraldehyde 3phosphate to 1,3 bis phosphoglycerate Glycolysis proceeds by the oxidation of glyceraldehde-3-phosphate,to form1,3-bis phosphoglycerate. Dihydroxyacetone phosphate also forms 1, 3 - bisphosphoglycerate via glyceraldehydes-3- phosphate shuttle.The enzyme responsible is Glyceraldehyde 3 phosphate dehydrogenase, which is NAD+ dependant. Energetics 1. In first reaction of this stage- NADH produced will be oxidized in electron transport chain to produce 3 ATP in presence of O2. Since two molecules of triose phosphate are formed per molecule of glucose oxidized, 2 NADH will produce 6 ATP. +6ATP 39 2. The second reaction will produce one ATP. Two molecules of substrate will produce ATP. +2ATP Net gain at this stage per molecule of glucose oxidized= + 8ATP Stage IV This is the recovery of the PO4 group form 3- phosphoglycerate. The two molecules of 3- phosphoglycerate the end- product of the previous stage, still retains the PO4 group originally derived form ATP in stage 1. Body wants to recover the two ATP spent in first stage for two phosphorylation reactions. This is achieved by following three reactions: 1. Conversion of 3- phosphoglycerate to 2- Phosphoglycerate 3-Phosphoglycerate formed by the above reaction is converted to 2-phosphoglycerate, catalyzed by the enzyme phosphoglycerate mutase. It is likely that 2,3 bisphosphoglycerate is an intermediate in the reaction and probably acts catalytically. 2. Conversion of 2-phosphoglycerate to Phosphoenol pyruvate The reaction is catalyzed by the enzyme enolase, the enzyme requires the presence of either Mg++ or Mn++ for activity. The reaction involves dehydration and redistribution of energy within the molecule raising the PO4 in position 2 to a “high – energy state”. 3. Conversion of phosphoenol pyruvate to pyruvate Phosphoenol pyruvate is converted to ‘Enol’ pyruvate, the reaction is catalyzed by the enzyme pyruvate kinase. The high energy PO4 of phosphoenol pyruvate is directly transferred to ADP producing ATP. Note Reaction is irreversible ATP is formed at the substrate level without electron transport chain. This is another example of “ substrate level phosphorylation “ in glycolytic pathway “Enol“ pyruvate is converted to ‘ Keto’ pyruvate spontaneously. But, cells having limited coenzymes, to continue the glycolytic cycle NADH must be oxidized to NAD+-. This is achieved by re oxidation of NADH by conversion of pyruvate to lactate (without producing ATP). 40 Significance of lactate formation: Under anaerobic conditions NADH is re oxidized via lactate formation. This allows glycolysis to proceed in the absence of oxygen. The process generates enough NAD for another cycle of glycolysis. B. Clinical Importance Tissues that function under hypoxic conditions will produce lactic acid from glucose oxidation. Produces local acidosis. If lactate production is more it can produce metabolic acidosis Vigorously contracting skeletal muscle will produce lactic acid. Whether O2 is present or not, glycolysis in erythrocytes always terminated in to pyruvate and lactate. Entry of fructose in to glycolysis: Liver contains specific enzymes fructokinase. It converts fructose to fructose 1 phosphate in the presence of ATP. In liver fructose1-phosphate is split to glyceraldehyde and dihydroxy acetone phosophate by AldolaseB. Glyceraldehyde enters glycolysis, when it is phosphorylated to glyceraldehyde-3-P by triose kinase. Dihydroxy aceton phosphate and glyceraldehyde-3-P may be degraded via glycolysis or may be condensed to form glucose by aldolase. Lack of fructose kinase leads to fructosuria. Absence of aldolaseB leads to hereditary fructose intolerance. If fructose 1, 6 bisphosphatase is absent, causes fructose induced hypoglycemia. The reason being high concentration of Fructose 1 phosphate and fructose 1, 6 bis phosphate inhibit Liver phosphorylase by allosteric modulation. As in case of Galactose, fructose intolerance can also lead to cataract formation. Galactose: Milk sugar contains galactose. Galactokinase converts galatose to galactose-1-P.It reacts with UDP-glucose to form UDP-galactose and glucose-1-P.The enzyme is Galactose-1-P uridyltransferase. UDP-galactose can be epimerized to UDP-glucose by 4- epimerase.Glycogenesis also requires UDP-glucose. UDP-galactose can be condensed with glucose to form lactose. 41 Galactosemia: Some people cannot metabolize galactose. It is an inherited disorder that the defect may be in the galactokinase, uridlyl transferase or 4-epimerase.Most common is uridyl transferase. Such patients have high concentration of Galactose in blood (Galactosemia).In lense, Galactose is reduced to galactitol by aldose reductase.The product accumulates in lense and leads to accumulation of water by osmotic pull. This leads to turbidity of lense proteins (Cataract). If uridyl transferase was absent galctose 1-phosphate accumulates.Liver is depleted of inorganic phosphate. This ultimately causes failure of liver function and mental retardation. If 4-epimerase is absent, since the patient can form UDP-galactose from glucose the patient remains symptom free. Glycogen metabolism Introduction Glycogen is the major storage form of carbohydrate in animals.It is mainly stored in liver and muscles and is mobilized as glucose whenever body tissues require. Degradation of Glycogen (glycogenolysis) A. Shortening of chains Golycogen phosphorylase cleaves the α-1, 4 glycosidic bonds between the glucose residues at the non reducing ends of the glycogen by simple phosphorolysis. This enzyme contains a molecules of covalently bound pyridoxal phosphate required as a coenzyme, Glycogen phosphorylase is a phosphotransferase that sequentially degrades the glycogen chains at their non reducing ends until four glucose units remain an each chain before a branch point. The resulting structure is called a limit dextrin and phosphorylase cannot degrade it any further. The product of this reaction is Glucose 1 phosphate. The glucose 1 phosphate is then converted to glucose 6 phosphate by phosphoglucomutase. Conversion of glucose 6 phosphate to glucose occurs in the Liver, Kidney and intestines by the action of Glucose 6 phosphatase. This does not occur in the skeletal muscle as it lacks the Enzyme. 42 B. Removal of Branches A debranching enzyme also called Glucantransferase which contains two activities, Glucantransferase and Glucosidase. The transfer activity removes the terminal 3 glucose residues of one branch and attaches them to a free C4 end of the second branch.The glucose in α-(1,6) linkage at the branch is removed by the action of Glucosidase as free glucose. C. Lysosomal Degradation of Glycogen A small amount of glycogen is continuously degraded by the lysosomal enzyme α-(1, 4) glycosidase (acid maltase). The purpose of this pathway is unknown. However, a deficiency of this enzyme causes accumulation of glycogen in vacuoles in the cytosol, resulting in a very serious glycogen storage disease called type II (Pomp’s disease), 43 Fig.2.9 Summary of Glycogenolysis Synthesis of Glygogen (Glycogenesis) Synthesis of glycogen from Glucose is carried out by the enzyme Glycogen Synthase.The activation of glucose to be used for glycogen synthesis is carried out by the enzyme UDP- glucose pyrophosphorylase. The enzyme exchanges the phosphate on C-1 of glucose-1- phosphate for UDP (Uridinediphosphate). The energy of the phospho glycosyl bond of UDP- glucose is utilized by glycogen Synthase to catalize the incorporation of glucose in to Glycogen. UDP is subsequently released from the enzyme.The α-1,6 branches in glucose are produced by amylo-(1,4-1,6) transglycosylase,also termed as branching enzyme.This enzyme transfers a terminal fragment of 6 to 7 glucose residues(from a polymer of atleast 11 glucose residues long) to an internal glucose residue at the C-6 hydroxyl position. 44 Fig 2.10. Glycogenesis Glycogen storage diseases These are a group of genetic diseases that result from a defect in an enzyme required for either glycogen synthesis or degradation.They result in either formation of glycogen that has an abnormal structure or the accumulation of excessive amounts of normal glycogen in specific tissues, A particular enzyme may be defective in a single tissue such as the liver or the defect may be more generalized, affecting muscle, kidney, intestine and myocardium. The severity of the diseases may range from fatal in infancy to mild disorders that are not life threatening some of the more prevalent glycogen storage diseases are the following. 45 Table of Glycogen Storage Diseases Type: Enzyme Affected Primary Manifestations Name Organ Type 0 glycogen synthase liver Hypoglycemia and early death, Type Ia: von glucose-6-phosphatase liver hepatomegaly, kidney Gierke's failure,fatty liver, hyperlacticacidimia and severe hypoglycemia Type II: lysosomal a-1,4- skeletal and muscular dystrophy, severe Pompe's glucosidase, lysosomal acid cardiac muscle cardiomegaly,early death. a-glucosidase acid maltase Type V: muscle phosphorylase skeletal Muscle excercise-induced McArdle's muscle cramps and pain, myoglobinuria The Pentose Phosphate Pathway The pentose phosphate pathway is primarily an anabolic pathway that utilizes the 6 carbons of glucose t