Medical Instrumentation Notes PDF
Document Details
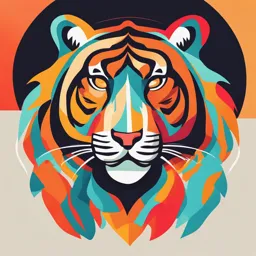
Uploaded by BrighterFantasticArt
Monash University
Tags
Related
- Lecture 3 Smart Medical Instrumentation System and Regulations PDF
- 1st Lecture 2024 PDF
- Medical Instrumentation Definition & Applications PDF
- Medical Instrumentation Clinical Rotation MLT406 PDF
- Medical Instrumentations (I) 2024/2025 PDF - Al-Rafidin University
- Mechatronic for Health Sciences Lecture No. 3 PDF
Summary
This document provides notes on medical instrumentation, including various types of sensors, transducers, and direct/indirect sensing methods. It details topics like electrical safety, temperature sensors, and characteristics of medical instruments.
Full Transcript
**Medical Instrumentation Notes:** Generalized medical instrumentation system Measurand: Physical quantity or parameter to be measured. Can be internal or external. Sensor: Transducer that converts physical energy to an electric output signal. Signal conditioning and processing: Amplification an...
**Medical Instrumentation Notes:** Generalized medical instrumentation system Measurand: Physical quantity or parameter to be measured. Can be internal or external. Sensor: Transducer that converts physical energy to an electric output signal. Signal conditioning and processing: Amplification and filtering. Output display: The output measured is visualized in a readable form. Feedback and control: In case the parameter needs correction and the subject needs to change a behavior. It can be with clinician or without clinician. **Sensors and transducers** Transducer: Converts one type of energy to another. Sensor: Converts physical parameters into an electrical output Actuator: Converts an electrical input into a physical output. A transducer converts primary forms of energy into a corresponding signal with a different energy form. Primary energy forms: - Mechanical - Thermal - Electromagnetic - Optical - Chemical **Direct and indirect sensing:** Direct measurement: When a sensor directly measures the parameter of interest. Indirect measurement: When a sensor measures a parameter that can be translated into the parameter of interest. Types of temperature sensors 1. Resistance based a. Resistance Temperature Devices (RTDs) b. Thermistors 2. Thermoelectric -- Thermocouples 3. Radiation thermometry 4. Fiber optic sensor **Electrical safety:** Conditions for electrocution: 1. A path must exist for current to flow 2. There must be two points of contact on the body: A. Current entry B. Current exit 3. The current must pass through or nearby the heart. At 60 Hz for 1-3 seconds: - 0.5 -- 2mA: Threshold of perception (When you first feel the stimulation). - Let-go current: When the subject starts feeling pain. - Maximum let-go current: The maximum pain the subject can tolerate and still have muscle control to let go of the stimulator. - Threshold of death: Current that will cause ventricular fibrillation (50-500mA). - Static characteristics: Describes the performance of instruments for dc and very low frequency inputs. - Accuracy - Precision - Resolution - Tolerance - Sensitivity - Calibration - Drift - Input Range - Input Impedance - Dynamic characteristics: Uses differential equations to describe the quality of measurements. - Characteristics of signals that are a function of time Complete characteristics: Static characteristics + Dynamic characteristics **The heart as a pump** - Na: Sodium - K: Potasium - Cl: Chlorine Mechanism: 1. Concentration of K+ ions is 30-50 times higher inside as compared to outside. Na+ concentration is 10 times higher outside than inside. In resting state the membrane is permeable for K+ ions only. 2. Potassium flows outwards leaving a balanced charge. 3. Electrostatic attraction pulls potassium and chloride ions close to the membrane. 4. Electric field directed inward forms. 5. Electrostatic force vs diffusional force Nernst equation: Determines the cell potential across the membrane for a specific ion. \ [\$\$V\_{k} = \\ - \\frac{\\text{RT}}{z\_{k}F}\\ln\\frac{c\_{i,k}}{c\_{o,k}}\$\$]{.math.display}\ [*V*~*k*~]{.math.inline}: Equilibrium potential for the ion R: Gas constant T: Temperature (Kelvin) z: Charge of the ion F: Faraday's constant C~i,k~ : Concentration for ion inside the membrane C~o,k~ : Concentration for ion outside the membrane Goldman-Hodgkin-Katz equation: Determines the overall membrane potential.  Vm ≈ -70... -100mV - Vm:the membrane potential. - R: gas constant. - T: the temperature in Kelvin. - F: Faraday\'s constant. - P: The permeabilities of the membrane for potassium, sodium, and chloride ions. - \[K+\]out\[K\^+\]\_{\\text{out}}\[K+\]out: represent the concentrations of these ions inside and outside the cell. **Neurons** Axons: Carry messages between somas around the body. Nodes of Ranvier: "Signal regenerators" along axons. The membrane in axons is relatively more permeable to K+. K+ leaves the high concentration by diffusion and leaves a negative charge. The inside of an axon is negative in resting state relative to the outside **Action potential** **Electrodes** An electrode is a metal-electrolyte interface that can form a half battery. In the anode: The metal oxidates and discharges cations into the electrolyte. Electrons flow to the cathode. In the cathode: Metal reduces (gains electrons released by the metal in the anode) and forms anions that flow into the electrolyte. Polarization: If there is a current between the electrode and electrolyte, the half-cell potential is altered due to polarization. Overpotential: Difference between the observed and theoretical potential needed to induce the electrochemical reaction in a half cell. a. Resistance/Ohmic: A voltage drop occurs due to the current encountering resistance. b. Concentration: Changes in distribution of ions at the electrode-electrolyte interface due to difference in concentration of ions. (This happens when there is a mismatch in the rate at which ions are released and attracted to the surface, due to a difference in concentration) c. Activation: Due to the kinetics of the electrochemical reaction. Polarizable and nonpolarizable electrodes Perfectly polarizable electrodes: - No charge crosses the electrode-electrolyte interface. - The electrode behaves like a capacitor - Overpotential is due to concentration - Mainly used for stimulation - Example: Platinum electrode Perfectly non-polarizable electrodes: - Current passes freely across the electrode-electrolyte interface - Minimal energy to make transition - No overpotential - Mainly used in recording - Example: Ag/AgCl electrode Circuit model of electrode behavior:  Rd and Cd: impedance associated with electrode-electrolyte interface Rs: Resistance in the electrolyte Ehc = Half-cell voltage, represented by a battery Biomedical signals +-----------+-----------+-----------+-----------+-----------+-----------+ | **Biomedi | **Descrip | **Amplitu | **Bandwid | **Sources | **Applica | | cal | tion** | de** | th** | of | tions** | | signal** | | | | error** | | +===========+===========+===========+===========+===========+===========+ | EEG | Electroen | 1-10 µV | 0.5-40Hz | Thermal | Sleep | | | cephalogr | | | RF noise | studies | | | aphy | | | | | | | measures | | | 50Hz | Seizure | | | the | | | power | detection | | | brain's | | | line | | | | electrica | | | | Cortical | | | l | | | Blink and | mapping | | | activity | | | other | | | | from the | | | artifacts | | | | scalp. | | | | | +-----------+-----------+-----------+-----------+-----------+-----------+ | EOG | Electrooc | 10-100 µV | 0-10Hz | Skin | Eye | | | ulography | | | potential | position | | | measures | | | and | | | | potential | | | motion. | Sleep | | | s | | | | state | | | created | | | | | | | as a | | | | Vestibulo | | | result of | | | | -ocular | | | the | | | | reflex | | | movement | | | | | | | of the | | | | | | | eyeballs. | | | | | +-----------+-----------+-----------+-----------+-----------+-----------+ | EMG | Electromy | 1-10mV | 20-2000Hz | 50 Hz | Muscle | | | ography | | | power | function | | | measures | | | line | | | | the | | | | Neuromusc | | | electric | | | RF | ular | | | activity | | | interfere | disease | | | of muscle | | | nce | | | | fibers | | | | Prosthesi | | | | | | | s | +-----------+-----------+-----------+-----------+-----------+-----------+ Data acquisition system overview Gait analysis Gait analysis is the systematic study of a subject's gait in order to: - Diagnose and treat a condition - Track treatment impact after illness or surgery - Enhance performance in sports - Assess risk of falls Gait cycle: The full cycle that one limb goes through from initial heel contact to the next heel contact by the same foot and is divided in two phases: stance and swing. - Stance phase: Limb is in contact with the ground working to keep balance. It takes 60% of the gait cycle time. - Swing phase: The limb is moving forward and divides in initial swing (acceleration), mid swing, and terminal swing (deceleration). This stage takes 40% of the gait cycle. Current technology for gait analysis Wearable systems: - IMU - EMG - Gyro - Ultrasound - Accelerometers - In-sole pressure sensors - Goniometers Non-wearable systems: - Video - Thermal video - Doppler radar - Laser tracker - Pressure sensitive walking surfaces - Clinician's eye Medical uses of accelerometry: - Gait and balance measurement - Pedometers - Fall prevention - Quality of sleep - Sports training Intracellular recording electrodes: Very invasive method of measuring action potentials that uses a micropipette filled with conductive liquid and is stuck through the axon's membrane. Extracellular recording electrodes: Measure the electrical activity of neurons from outside the cell membrane, without penetrating the cell. Neural signals Signal Types: - - - - - - List of signal acquisition techniques in order of invasiveness: 1\. SUA, MUA, LFP 2\. ECoG 3\. MEG and EEG List of signal acquisition techniques in order of size of neuronal cluster: 1\. SUA 2\. MUA 3\. LFP 4\. ECoG 5\. MEG and EEG List of signal acquisition techniques in order of spatial resolution: 1\. SUA 2\. MUA 3\. LFP 4\. ECoG 5\. MEG and EEG Electrode Arrays One dimensional array  Example - Neuropixels, 800 electrodes per shank. Used for ultra high density recordings Two dimensional array: Example: - Flexible surface electrode arrays. Downside: Difficult to get a lot of wires out of the brain. Three dimensional array:  Example: - Blackrock array (Utah array), 100 electrodes Arrhythmias SA Block - 1˚ degree: There is a delay between sinus node activation and atrial activation - 2˚ degree: There are missing heartbeats, the arrhythmia cannot be distinguished from bradycardia because of the slower heart rate. - 3˚ degree: No impulses from the sinus node reach the atria. Only ventricular escape is recorded. Pacemakers A pacemaker uses electrical impulses delivered by electrodes in contact with the heart muscles to regulate the beating of the heart. - It uses very low current and low duty cycle. - It's needed when the heart is not stimulating properly on its own. - Pulses are conducted in: Epicardium, myocardium or endocardium. Two main components: - Pulse generator (battery) - Leads or wires Types of pacemakers based on functionality: 1\. Sequential Can be single or dual chamber. Most systems are dual chamber, which means that is will sense and stimulate the upper chamber and lower chamber in a synchronized way. 2\. Asynchronous (fixed rate) No coordination with the natural activation of the heart. 3\. Synchronous (demand) Coordinates with the natural pacemaker activity from the heart. Ventricular fibrillation: Type of arrhythmia in which the beating of the heart cells is uncoordinated resulting in no blood pressure, and the ECG trace is erratic with no discernible QRS complexes. Defibrillation: It consists of delivering a therapeutic dose of electrical energy to the affected heart. Automated external defibrillators: Automate the diagnosis and treatment of treatable rhythms. Parts of implantable defibrillator:  Defibrillator types based on the electrodes placement: - Internal - External Defibrillator types based on the nature of voltage applied: - AC Defibrillator - DC Defibrillator - Synchronized DC Defibrillator - Square Pulse Defibrillator - Double Square Pulse Defibrillator - Biphasic DC Defibrillator Implantable automatic defibrillators consist of: - A means of sensing cardiac fibrillation or tachycardia - A power supply - Electrodes for delivery of stimuli Signal processing is used to control stimulation (electrical and mechanical signals are used). Stimuli of 5 to 30 Joules. Plethysmograph A plethysmograph is an instrument used mainly to determine and register the variations volume. It can be used to determine blood volume and flow in the body changing with each heartbeat. It can also be used to register air volume for pulmonary function tests. Types of plethysmograph: - Optical sensor (photoplethysmography): Light absorption varies as blood volume changes, thus the amount of the backscattered light is detected by the sensor. - Impedance based: As blood volume changes, impedance changes and this is measured by inducing a small AC current and registering the voltage using two pelectrodes. - Water displacement: The volume of the limb is measured by the displacement of water. Photoplethysmography (PPG) The PPG wave is usually analysed together with its first and second derivative to facilitate interpretation and recognize inflection points. PPG is affected by: heartbeat, haemodynamics, and change in properties of an arteriole. Effects are observed as distortions in the wave profiles. PPG diagnostic structure: PPG signal quality depends on: - Location - Blood oxygen saturation - Blood flow rate - Skin temperature - Measuring environment PPG waveform is subject to sudden amplitude changes due to the automatic gain controller and a low amplitude PPG signal may result. PPG Noise: Powerline interference Design Factors Choice and design of instruments are affected by: - Signal factors 1. Sensitivity 2. Range 3. Linearity 4. Reliability - Environmental factors 5. Signal to noise ratio 6. Stability 7. Temperature 8. Humidity 9. Pressure 10. Acceleration 11. Power requirement - Medical factors 12. Invasiveness 13. Electric safety 14. Radiation 15. Patient discomfort - Economic factors 16. Cost 17. Availability 18. Compatibility 19. Warranty Design steps: 1\. Initial design 2\. Prototype test 3\. Final design 4\. TGA approval 5\. Production Regulatory Environment Regulatory agency in Australia: Therapeutic Goods Administration Medical device: Any instrument, apparatus, appliance, material, or other article, intended to be used for: - Diagnosis, prevention, monitoring, treatment, or alleviation of disease. - Diagnosis, monitoring, treatment, alleviation, or compensation for an injury or handicap. - Investigation, replacement or modification of the anatomy or of a physiological process. - Control or contraception And does not achieve this by pharmacological, immunological, or metabolic means. TGA Device Classes: Classification is based on: - Intended use - Level of risk - Degree of invasiveness in human body **Medical Device Classification** **Level of Potential Harm** ----------------------------------- ----------------------------- Class I Lowest Class Is, Class Im Low Class IIa Low to moderate Class IIb Moderate to High Class III, AIMD High Class I -Wheelchairs -Stethoscopes -Spectacles -Reusable drills -Beds -External electrodes -Gels Class IIa -Anaesthetic breathing circuits -Dental drills -External bone growth stimulators -Dental prosthesis -Hearing aids -MRI equipment Class IIb -Anesthetic machines -Apnea monitors -Baby incubators -Blood pumps for heart lung machines -Blood warmers -Diagnostic x ray sources -Orthopedic implants -External defibrillators -Intensive care monitoring systems -Lung ventilators -Surgical lasers Class III -Absorbable sutures -Breast implants -Heart valves -Intrauterine devices Class AIMD -Implantable drug infusion devices -Implantable electrodes -Implantable pulse generators Device compliance levels