MCB3020 Viruses and Other Acellular Infectious Agents (Textbook Chapter 6) PDF
Document Details
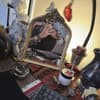
Uploaded by rafawar1000
Florida Atlantic University
Tags
Summary
This chapter from a microbiology textbook introduces viruses and other acellular infectious agents, discussing their structure, multiplication, and significance. It covers different types of capsid symmetry (helical and icosahedral) and examples of viruses with various structures, including the simple virion structure and an example of a complex virus, vaccinia virion.
Full Transcript
6 Viruses and Other Acellular Infectious Agents ©Ingram Publishing/age fotostock Mustard, Ketchup, and Viruses? T wenty million hot dogs are sold annually to fans attending games at major league baseball parks in the United States. Hot dogs and lunch meats are popular at baseball games and in lun...
6 Viruses and Other Acellular Infectious Agents ©Ingram Publishing/age fotostock Mustard, Ketchup, and Viruses? T wenty million hot dogs are sold annually to fans attending games at major league baseball parks in the United States. Hot dogs and lunch meats are popular at baseball games and in lunches carried to work or school. Yet each year in the United States, approximately 1,600 people are sickened by a bacterium that can contaminate the meat and, worse, survive and grow even when the meat is properly refrigerated. The disease culprit is Listeria monocytogenes, a Gram-positive rod found in soil and many other environmental sites. It is not only cold tolerant but salt and acid tolerant as well. Although it is in the minor leagues when compared to some of the big hitters of food-borne disease (e.g., Salmonella enterica), it is of concern for two reasons: who it kills and how many it kills. L. monocytogenes targets the young and old, pregnant women, and immunocompromised individuals; about 15% of those infected die. Its effect on pregnant women is particularly heartbreaking. The woman usually only suffers mild, flulike symptoms; however, these innocuous symptoms belie the fact that the child she carries is in serious danger. Her pregnancy often ends in miscarriage or stillbirth. Newborns infected with the bacterium are likely to develop meningitis. Many will die as a result. Those who survive often have neurological disorders. Currently, pregnant women are counseled against eating ready-toeat foods unless they have been cooked prior to consumption. However, L. monocytogenes is known to contaminate many foods other than hot dogs and these can’t always be heated. In 2006 the U.S. Food and Drug Administration (FDA) approved a new approach to prevent listeriosis: spraying viruses that attack and destroy the bacterium on ready-to-eat cold cuts and luncheon meats. In other words, the viruses are a food additive! The method is safe because the viruses only attack L. monocytogenes, not human cells. Since approval, the use of viruses to control the transmission of listeriosis by other foods has been studied. Unfortunately, those studies did not include fresh fruit. In 2011 L. monocytogenes–contaminated cantaloupe caused an outbreak of listeriosis in 20 U.S. states, which infected over 100 and killed over 20. Viruses as agents of good will come as a surprise to many. Typically we think of them as major causes of disease. However, viruses are significant for other reasons. They are vital members of aquatic ecosystems. There they interact with cellular microbes and contribute to the movement of organic matter from particulate forms to dissolved forms. Bacterial viruses are being used in some European countries to treat bacterial infections, and a number of animal viruses are being used to target and destroy cancer cells. Finally, they are important model organisms. In this chapter, we introduce viruses and other acellular infectious agents. Marine viruses: mortality at sea (section 30.2); Microorganisms can be controlled by biological methods (section 8.7) Readiness Check: Based on what you have learned previously, you should be able to: ✓ Define the term acellular ✓ Compare and contrast in general terms viruses, viroids, satellites, and prions (section 1.1) 6.1 Viruses Are Acellular After reading this section, you should be able to: a. Define the terms virology, bacteriophages, and phages b. List organisms that are hosts to viruses The discipline of virology studies viruses, a group of infectious agents unique in their simple, acellular organization and pattern of multiplication. Despite this simplicity, viruses are major causes of disease. For instance, many human diseases are caused by viruses, and more are discovered every year, as demonstrated by the appearance of the H1N1 (swine) influenza virus in 2009, the H7N9 avian influenza virus in 2013, and the Middle East respiratory syndrome coronavirus (MERS-CoV) also in 2013. However, their simplicity also has made them attractive model organisms. They served as models for understanding DNA replication, RNA synthesis, and protein synthesis. Therefore the study of viruses has contributed significantly to the discipline of molecular biology. In fact, the field of genetic engineering is based in large part on the use of viruses and viral enzymes such as the enzyme reverse transcriptase. Microbial DNA technologies (chapter 17) Viruses can exist either extracellularly or intracellularly. When extracellular, they are inactive because they possess few, if any, enzymes and cannot reproduce outside of living cells. When intracellular, viruses exist primarily as nucleic acids that can, at 106 wil11886_ch06_106-127.indd 106 23/10/18 9:12 am 6.2 Virion Structure Is Defined by Capsid Symmetry and Presence or Absence of an Envelope 107 some point in the viral life cycle, commandeer host cells and use them to synthesize viral components from which progeny virions are assembled and eventually released. Viruses can infect all cell types. Numerous viruses infect bacteria. They are called bacteriophages, or phages for short. Fewer archaeal viruses have been identified. Most known viruses infect eukaryotic organisms, including plants, animals, protists, and fungi. Viruses have been classified into numerous families based primarily on genome structure, life cycle, morphology, and genetic relatedness. Virus phylogeny is difficult to establish (section 26.1) In this chapter, we introduce the structure and multiplication strategies of viruses. Their taxonomic diversity is presented in chapter 26, their ecological relevance is discussed in chapter 30, and their role in human disease is the topic of chapter 38. 6.2 Virion Structure Is Defined by Capsid Symmetry and Presence or Absence of an Envelope After reading this section, you should be able to: a. b. c. d. State the size range of virions Identify the parts of a virion and describe their function Distinguish enveloped viruses from nonenveloped viruses Describe the types of capsid symmetry A complete virus particle is called a virion. Virion morphology has been intensely studied because of the importance of viruses and the realization that virion structure is simple enough to be understood in detail. Progress has come from the use of several different techniques: electron microscopy, X-ray diffraction, biochemical analysis, and immunology. Although our knowledge is incomplete due to the vast diversity of viruses, we can discuss the general nature of virion structure. General Structural Properties Virions can be extraordinarily tiny (about 20 nm in diameter) to about the same size as a rod-shaped bacterial cell (1.5 × 0.5 µm) (figure 6.1). The smallest are a little larger than ribosomes, whereas mimiviruses, among the largest viruses known, can be seen in the light microscope. However, most virus particles must be viewed with electron microscopes. Electron microscopes use beams of electrons to create highly magnified images (section 2.4) The simplest virions consist only of a nucleocapsid, which is composed of a nucleic acid, either DNA or RNA, and a protein coat called a capsid (figure 6.2). The capsid surrounds the viral nucleic acid, protects the viral genome, and often aids in its transfer between host cells. Some virions are covered by a lipid membrane, and these are termed enveloped viruses, whereas those lacking a membrane are called nonenveloped or naked viruses. Notice what is missing from viruses: ribosomes for protein synthesis and a mechanism for generating ATP. Cytoplasm is wil11886_ch06_106-127.indd 107 absent, and while a few enzymes may be found, there are not enough to sustain cellular processes. Nonenveloped viruses construct a capsid from many copies of one protein and a few minor proteins. Each subunit is termed a protomer, and thousands of protomers self-assemble to form the capsid (figure 6.3). In contrast, enveloped viruses require both nucleocapsid proteins and additional proteins to anchor the membrane. Some viruses use noncapsid proteins as scaffolding upon which the capsids are assembled. Probably the most important advantage of this design strategy is that the viral genome is used with maximum efficiency. For example, the tobacco mosaic virus (TMV) capsid is constructed using a single type of protomer. Recall that the building blocks of proteins are amino acids and that each amino acid is encoded by three nucleotides. The TMV protomer is 158 amino acids in length. Therefore only about 474 nucleotides are required to code for the coat protein. The entire TMV genome consists of only 6,400 nucleotides. Thus only a small fraction of the genome is used to code for the capsid. Suppose, however, that the TMV capsid were composed of six different protomers, all about 150 amino acids in length. If this were the case, about 2,700 nucleotides in the TMV genome would be required just for capsid construction, and much less genetic material would be available for other purposes. Helical Capsids Helical capsids are shaped like hollow tubes with protein walls. Tobacco mosaic virus is a well-studied example of helical capsid structure (figure 6.3). The self-assembly of TMV protomers into a helical arrangement produces a rigid tube. The capsid encloses an RNA genome, which is wound in a spiral and lies within a groove formed by the protein subunits. Not all helical capsids are as rigid as the TMV capsid. The nucleocapsids of influenza viruses are thin and flexible and are enclosed within an envelope (figure 6.4). Tobacco mosaic virus (section 26.5) The size of a helical capsid is influenced by both its protomers and the viral genome. The diameter of the capsid is a function of the size, shape, and interactions of the protomers. The length of the capsid appears to be determined by the nucleic acid because a helical capsid does not extend much beyond the end of the viral genome. Icosahedral Capsids An icosahedron is a regular polyhedron with 20 equilateral triangular faces and 12 vertices (figure 6.1b,g,h). Icosahedral capsids are the most efficient way to enclose a space. They are constructed from ring- or knob-shaped assemblages of five or six protomers; the assemblages are called capsomers (figure 6.5). Capsomers composed of five protomers are called pentamers (pentons); hexamers (hexons) are capsomers that possess six protomers. Pentamers are usually at the vertices of the icosahedron, whereas hexamers generally form its edges and triangular faces. The virions of some RNA viruses have pentamers and hexamers constructed with only one type of subunit. Other virions have pentamers and hexamers composed of different proteins. 23/10/18 9:12 am 108 CHAPTER 6 | Viruses and Other Acellular Infectious Agents (a) Vaccinia virus (b) Herpesvirus (c) Rhabdovirus (d) T-even coliphage (f ) Sulfolobus spindleshaped virus 1 (e) A flexuoustailed phage (h) ϕX174 phage (g) Human papillomavirus (i) Tobacco mosaic virus (j) Mimivirus (k) Acidianus two-tailed virus 1 µm Figure 6.1 The Size and Virion Morphology of Selected Viruses. The virions are drawn to scale. MICRO INQUIRY Which capsids are icosahedral? Which are helical? Which have complex symmetry? Although many icosahedral capsids contain both pentamers and hexamers, some have only pentamers. Capsids of Complex Symmetry Most viruses have either icosahedral or helical capsids, but some viruses do not fit into either category. Poxviruses and large bacteriophages are two important examples. Poxvirus virions are among the largest of the animal viruses (about 400 by 240 by 200 nm in size) and can be seen with a light microscope. They possess an exceptionally complex internal wil11886_ch06_106-127.indd 108 structure with an ovoid- to brick-shaped exterior. Figure 6.6 shows the virion morphology of the vaccinia virus. Its doublestranded DNA genome is associated with proteins and contained in the core, a central structure shaped like a biconcave disk and surrounded by a membrane. Two lateral bodies lie between the core and the virion’s outer envelope and contain viral enzymes. Some large bacteriophages have virions that are even more elaborate than those of poxviruses. The virions of T2, T4, and T6 phages (T-even phages) that infect Escherichia coli are said to have binal symmetry because they have a head that resembles an icosahedron and a tail that is helical. The icosahedral head is 23/10/18 9:12 am 6.2 Virion Structure Is Defined by Capsid Symmetry and Presence or Absence of an Envelope 109 usually arise from the plasma or nuclear membranes of the host cell. Envelope lipids and carbohydrates are therefore acquired from the host. In contrast, envelope proteins are coded for by viral genes and may even project from the envelope surface as spikes, which are also called peplomers (figure 6.8). In many cases, spikes are involved in virion attachment to the host cell surface. Because spikes differ among viruses, they also can be used to identify some viruses. Many enveloped viruses have virions with a somewhat variable shape and are called pleomorphic. However, the envelopes of viruses such as the bullet-shaped rabies viruses are firmly attached to the underlying nucleocapsid and endow the virion with a constant, characteristic shape (figure 6.8a). Influenza virus (figure 6.4) is a well-studied enveloped virus with two types of spikes. Some spikes consist of the enzyme neuraminidase, which functions in the release of mature virions from the host cell. Other spikes are hemagglutinin proteins, so named because they bind virions to red blood cells and cause the cells to clump together—a process called hemagglutination. Influenza virus’s hemagglutinins participate in virion attachment to host cells. Most of its envelope proteins are glycoproteins—proteins that have carbohydrate attached to them. A nonglycosylated protein, the M1 (matrix) protein, is found on the inner surface of the envelope and stabilizes both the lipid envelope and the display of both types of spike. In addition to enzymes associated with the envelope or capsid (e.g., influenza neuraminidase), some viruses have enzymes within their capsids. Such enzymes are usually involved in nucleic acid replication. For example, influenza virus virions have an RNA genome and carry an enzyme that synthesizes RNA using Envelope Spike Capsid Capsid Nucleic acid Nucleic acid (a) Nonenveloped virus (b) Enveloped virus Figure 6.2 Generalized Structure of Virions. (a) The simplest virion is that of a nonenveloped virus, consisting of a capsid assembled around its nucleic acid (nucleocapsid). (b) Virions of enveloped viruses are composed of a nucleocapsid surrounded by a membrane called an envelope. The envelope usually has viral proteins called spikes inserted into it. elongated by one or two rows of hexamers in the middle and contains the DNA genome (figure 6.7). The tail is composed of a collar joining it to the head, a central hollow tube, a sheath surrounding the tube, and a complex baseplate. In T-even phages, the baseplate is hexagonal and has a pin and a jointed long tail fiber and a short tail fiber at each corner. Bacteriophage T4: a virulent bacteriophage (section 26.2) Viral Envelopes and Enzymes The nucleocapsids of many animal viruses, some plant viruses, and at least one bacterial virus are surrounded by an outer membranous layer called an envelope (figure 6.8). Animal virus envelopes Protomer (a) 0 (b) RNA 10 nm 20 nm (c) Figure 6.3 Tobacco Mosaic Virus (TMV) Virions. (a) An electron micrograph of negatively stained helical capsids. The virions are 15 to 18 nm in diameter and 300 nm long. (b) Illustration of a TMV nucleocapsid. It is composed of a helical array of protomers with the RNA spiraling on the inside. (c) A model of a TMV virion. (a) ©Science History Images/Alamy Stock Photo wil11886_ch06_106-127.indd 109 23/10/18 9:12 am 110 CHAPTER 6 | Viruses and Other Acellular Infectious Agents Envelope Nucleocapsid 80–120 nm (a) Figure 6.4 Influenza Virus Virions: An Enveloped Virus with Helical Nucleocapsids. (a) Schematic view. Influenza viruses have segmented genomes consisting of seven to eight different RNA molecules. Each is coated by capsid proteins to create seven to eight flexible nucleocapsids. The envelope has two types of spikes that project 10 nm from the surface at 7- to 8-nm intervals. (b) Because the nucleocapsids are flexible, the virions are pleomorphic. Electron micrograph. (b) Source: CDC/Dr. F.A. Murphy an RNA template (see figure 26.24). Thus although viruses lack true metabolism and cannot reproduce independently of living cells, their virions may carry one or more enzymes essential to the completion of their life cycles. Viral Genomes Are Structurally Diverse One clear distinction between cellular organisms and viruses is the nature of their genomes. Cellular genomes are always doublestranded (ds) DNA. Viruses, on the other hand, employ all (b) four possible nucleic acid types: dsDNA, single-stranded (ss) DNA, ssRNA, and dsRNA. All four types are used by animal viruses. Most plant viruses have ssRNA genomes, and most bacterial and archaeal viruses have dsDNA. The size of viral genomes also varies greatly. Very small genomes are around 4,000 nucleotides—just large enough to code for three or four proteins. Some viruses save additional space by using overlapping genes. At the other extreme are the genomes of pandoraviruses, which infect protists. They are about 2.5 × 10 6 (a) (b) Figure 6.5 The Icosahedral Capsid of an Adenovirus. (a) Electron micrograph of adenovirus virions, 252 capsomers. (b) Computer-simulated model of an adenovirus virion. (a) ©Biophoto Associates/Science Source; (b) ©Division of Computer Research & Technology, NIH/Science Source MICRO INQUIRY What is the difference between a pentamer and a hexamer? Are the capsomers at the vertices of an adenovirus virion pentamers or hexamers? wil11886_ch06_106-127.indd 110 23/10/18 9:12 am 6.2 Virion Structure Is Defined by Capsid Symmetry and Presence or Absence of an Envelope 111 240– 300 nm DNA Core membrane 200 nm Nucleic acid Envelope Outer envelope Soluble protein antigens Lateral body Core membrane (a) Lateral body (b) Figure 6.6 Morphology of Vaccinia Virus Virions. (a) Diagram of virion structure. (b) Electron cryotomogram of the virion. (b) ©Marek Cyrklaff Nucleic acid Capsid head Collar Sheath Long tail fibers Short tail fibers Tail pins Baseplate (a) (b) Figure 6.7 T4 Phages of E. coli. (a) The structure of T4 bacteriophage virion. (b) Electron micrograph of the virion. (b) ©Ami Images/Science Source MICRO INQUIRY Why is T4 said to have binal symmetry? nucleotides long, exceeding some bacteria and archaea in coding capacity. Most DNA viruses use dsDNA as their genetic material. However, some have ssDNA genomes (e.g., ϕX174 and M13). In both cases, the genomes may be either linear or circular. The ends of linear viral genomes may be covalently closed, attached to a protein, or otherwise masked. Some DNA genomes can switch from one form to the other. For instance, the E. coli phages lambda has a linear genome in its capsid, but it becomes circular once it enters the host cell. Bacteriophages ϕX174 and Pf1 (section 26.3); Bacteriophage lambda: a temperate bacteriophage (section 26.2) wil11886_ch06_106-127.indd 111 Relatively few RNA viruses have dsRNA genomes. More common are viruses with ssRNA genomes. Polio, tobacco mosaic, rabies, influenza, and human immunodeficiency (HIV) are all ssRNA viruses. Some RNA viruses have segmented genomes—genomes that consist of multiple pieces (segments) of RNA. In many cases, each segment codes for one protein and there may be as many as 10 to 12 segments. Usually all segments are enclosed in the same capsid (figure 6.4). However, the genome of brome mosaic virus, a virus that infects certain grasses, is composed of three segments distributed among three different virions. 23/10/18 9:12 am 112 CHAPTER 6 | Viruses and Other Acellular Infectious Agents Envelope spike Nucleocapsid Tegument Envelope Glycoprotein β envelope spikes (a) Rabies virus (b) Herpesvirus Figure 6.8 Examples of Enveloped Viruses. (a) Negatively stained virion of a rabies virus. (b) Herpesvirus virion. Images are artificially colorized. (a) ©Chris Bjornberg/Science Source; (b) ©Dr. Linda Stannard, UCT/Science Source Comprehension Check 1. How are viruses similar to cellular organisms? In what fundamental way do they differ? 2. What is the difference between a nucleocapsid and a capsid? 3. Compare the structure of an icosahedral capsid with that of a helical capsid. How do pentamers and hexamers associate to form a complete icosahedron? Which virus would have a longer helical capsid: a virus with a 7,200 base pair DNA genome or one with an 11,000 base ssRNA genome? 4. What is an envelope? What are spikes (peplomers)? Why do some enveloped viruses have pleomorphic virions? 5. All four nucleic acid forms can serve as viral genomes. Describe each. What is a segmented RNA genome? 6.3 Viral Life Cycles Have Five Steps After reading this section, you should be able to: a. Describe the five steps common to the life cycles of viruses b. Discuss the role of receptors, capsid proteins, and envelope proteins in the life cycles of viruses c. Describe the two most common methods for virion release from a host cell In the early years of virology, one of the fundamental questions was how progeny viruses are made. The one-step growth experiment devised in 1939 by Max Delbrück (1906–1981) and Emory Ellis (1906– 2003) served as an experimental approach to answering this question. Delbrück and Ellis worked with bacteriophage T4. They knew that T4 lysed its host, Escherichia coli, and released progeny phages. In their experiment, E. coli cells were mixed with T4. After a short interval, the mixture was greatly diluted so that any virions released upon host cell lysis would not encounter and infect other cells. The diluted culture was then incubated, and over time samples were removed to determine the number of infectious phages particles in the culture. This wil11886_ch06_106-127.indd 112 was determined using a plaque assay as we describe in section 6.5. A plot of the number of phages particles versus time shows several distinct periods in the resulting growth curve (figure 6.9, red line). The latent period occurs immediately following addition of the phages. During this period, no virions are released. The rise period follows and is characterized by the rapid release of infective phages. Finally, a plateau is reached and no more virions are produced. The one-step growth experiment was important for several reasons. It employed procedures that are still used today to culture and enumerate viruses, and it ushered in the modern era of phages biology. It also led to another fundamental question: What is occurring during the latent period? In a subsequent set of experiments, infected cells were artificially lysed during the latent period. This revealed that intracellular virions could not be detected early in the latent period. In essence, the phages disappeared once inside the cell. This period is called the eclipse period because the infecting virions were concealed or eclipsed within the host cell. These experiments also demonstrated that the number of completed, infective phages within the host cell increases after the end of the eclipse period. Still other experiments eventually showed that a carefully orchestrated series of events occurs during the latent period. These events are the focus of this section. If a virus is to multiply and give rise to new progeny viruses, it must find and use (and in many cases abuse) a host cell. To accomplish this, a virus must use guile and subterfuge to access an appropriate host, enter the host, and avoid any defenses the host might employ to rid itself of the virus or prevent its multiplication. Once inside a host cell, a virus uses a repertoire of clever tricks to take control of cellular functions, thereby ensuring that viral genomes, mRNAs, and proteins are synthesized using ATP generated by the host. There are a plethora of distinctive viral life cycles (also called viral replicative cycles). Viral strategies are often related to virion structure, in particular the nature of its genome. Thus viruses with a similar type of genome (e.g., dsDNA, ssRNA) often employ similar tactics, as we explore in chapter 26. 23/10/18 9:12 am 6.3 Viral Life Cycles Have Five Steps 113 Latent period Rise period Virus particle Eclipse Host cell Phage count Attachment of virion to host cell Burst size Entry of viral nucleocapsid Time (minutes) Figure 6.9 The One-Step Growth Curve. The eclipse period is the initial portion of the latent period in which host cells do not contain any complete, infective virions. During the remainder of the latent period, an increasing number of infective virions are present, but none are released. The latent period ends with host cell lysis and rapid release of virions (rise period). In this figure the blue line represents the total number of complete virions, both intracellular and free in the medium. The red line represents the number of free viruses (virions that were free in the culture and had not infected a host cell plus those virions that were released from infected cells). The burst size is the number of progeny viruses produced per infected cell. Despite the diversity of viral life cycles, a general pattern of viral replicative cycles can be discerned; it can be divided into five steps. Because viruses need a host cell in which to multiply, the first step is usually attachment, often called adsorption (Latin sorbere, to suck in), to a host (figure 6.10). This is followed by entry of either the entire nucleocapsid or just the viral nucleic acid into the host. If the nucleocapsid enters, uncoating of the genome usually occurs before the life cycle continues. Once inside the host cell, the synthesis stage begins. During this stage, viral genes are transcribed and translated. This allows the virus to take control of the host cell, forcing it to manufacture viral genomes and viral proteins. Next is the assembly stage, during which new nucleocapsids are constructed by self-assembly of coat proteins and nucleic acids are packaged within. Finally, during the release step, mature virions escape the host. Attachment (Adsorption) All viruses, with the exception of plant viruses, must attach to a potential host cell long enough to gain entry into the cell. Attachment to the host is accomplished by specific interactions between molecules on the surface of the virion (ligands) and molecules on the surface of the host cell called receptors. In many cases, the viral surface ligand is a protein, but some animal viruses use lipids derived from the cell membrane as ligands. A recurring theme among archaeal viruses is the presence of virion appendages for host cell attachment. These include beardlike fiber wil11886_ch06_106-127.indd 113 Viral proteins Viral nucleic acids Synthesis of viral proteins and nucleic acids Self-assembly of virions Release of progeny virions Figure 6.10 Generalized Illustration of Virus Multiplication. The life cycle of a virus that infects a eukaryotic cell is shown. Figure 6.16 illustrates the generalized life cycle of many bacterial and archaeal viruses. Virions are not drawn to scale. 23/10/18 9:12 am 114 CHAPTER 6 | Viruses and Other Acellular Infectious Agents clusters and structures resembling claws. Bacteriophages attach to cell wall lipopolysaccharides and proteins as receptors, while others use teichoic acids, flagella, or pili. Binding of an animal virus particle to its receptor often causes conformational changes in virion proteins that facilitate interaction with secondary receptors, entry into the host, and nucleocapsid uncoating. Receptor specificity is at least partly responsible for the preferences viruses have for a particular host. Bacteriophages not only infect a particular bacterial species but often infect only certain strains within a given species. Likewise, animal viruses infect specific animals and, in some cases, only particular tissues within that host. However, if the receptor recognized by a virus is present in numerous animals, then the virus will infect more than one animal species. Such is the case with rabies viruses. Viruses have evolved such that they use host receptors that are always present on the surface of the host cell and are important for normal host cell function. In some cases, two or more host cell receptors are involved in attachment. For instance, human immunodeficiency virus (HIV) particles bind to two different proteins on human cells (e.g., CD4 and CCR5). In complex animals like humans, the presence of receptor molecules on a cell surface varies with the organ and tissue. Viruses have a tropism; that is, particular cell types are infected based on the distribution of surface receptors in tissues. For example, poliovirus receptors are found only in the human nasopharynx, gut, and anterior horn cells of the spinal cord. Therefore polioviruses infect these tissues, causing disease that ranges from milder forms such as gastrointestinal disease to more serious paralytic disease. In contrast, measles virus receptors are present in most tissues and disease is disseminated throughout the body. Some viruses, including Ebola, mimic damaged cells by exposing certain phospholipids on their surface. This display is a hallmark of dying cells targeted for phagocytosis. By camouflaging themselves as damaged cells, viruses can gain entry to a wide variety of cell types. Eukaryotic cell envelopes (section 5.2) Human diseases caused by viruses and prions (chapter 38) Plant viruses are a notable exception to attachment based on receptor binding as no receptors have been identified for plant viruses. Rather, damage of host cells is required for the virus particles to enter the host. Infection is often achieved by plant-eating insects that carry virions from one plant to another. The virions are deposited in plant tissues as the insect devours the plant. Interestingly, some viruses may alter their plant hosts to promote activity of the insects and thereby foster transmission to new plants. Entry into the Host After attachment to the host cell, the virus’s genome or the entire nucleocapsid enters the cytoplasm. For many bacteriophages only their nucleic acid enters the host’s cytoplasm, leaving the capsid outside and attached to the cell. In contrast to phages, many eukaryotic viral nucleocapsids enter the cytoplasm with the genome still enclosed. Once inside the cytoplasm, some shed their capsid proteins in a process called uncoating, whereas others remain encapsidated. Because penetration and uncoating are often coupled, we consider them together. wil11886_ch06_106-127.indd 114 The mechanisms of penetration and uncoating vary with the type of virus, and for many animal viruses, detailed mechanisms of penetration are unclear. However, it appears that one of three different modes of entry is usually employed by animal viruses: fusion of the viral envelope with the host cell’s plasma membrane, entry by endocytosis, and release of viral nucleic acid into the cytoplasm of the host cell (figure 6.11). Entry of Animal Viruses into Host Cells Fusion of viral envelopes with the host cell’s plasma membrane often involves viral envelope glycoproteins or phospholipids that interact with proteins in the plasma membrane of the host cell (figure 6.11a). This interaction sets into motion events that allow the nucleocapsid to enter. For example, after attachment of paramyxovirus virions (e.g., the measles virus), membrane lipids rearrange, the adjacent halves of the contacting membranes merge, and a proteinaceous fusion pore forms. The nucleocapsid then enters the host cell cytoplasm, where a viral enzyme carried within the nucleocapsid begins synthesizing viral mRNA while it is still within the capsid. Virions of nonenveloped viruses and some enveloped viruses enter cells by one of the endocytic pathways, including clathrindependent endocytosis and macropinocytosis (figure 6.11b). The resulting endocytic vesicle contains the virion and fuses with an endosome; depending on the virus, escape of the nucleocapsid or its genome from the endocytic vesicle may occur either before or after fusion with an endosome. Endosomal enzymes can aid in virion uncoating, and low pH often triggers the uncoating process. For some enveloped viruses, the viral envelope fuses with the endosomal membrane, and the nucleocapsid is released into the cytosol (the capsid proteins may have been partially removed by endosomal enzymes). Once in the cytosol, the viral nucleic acid may be released from the capsid upon completion of uncoating or may function while still attached to capsid components. Nonenveloped animal viruses cannot employ the membrane fusion mechanism for release from the endosome (figure 6.11c). In this case, it is thought that the low pH of the endosome causes a conformational change in the capsid. The altered capsid contacts the endosome membrane and either releases the viral nucleic acid into the cytosol or ruptures the membrane to release the intact nucleocapsid. There are several endocytic pathways (section 5.4) Synthesis Stage This stage of the viral life cycle differs dramatically among viruses because the genome of a virus dictates the events that occur. For dsDNA viruses, the synthesis stage can be very similar to the typical flow of information in cells. That is, the genetic information is stored in DNA and replicated by enzymes called DNA polymerases, recoded as mRNA (transcription), and decoded during protein synthesis (translation). Because of this similarity, some dsDNA viruses have the luxury of depending solely on their host cells’ biosynthetic machinery to replicate their genomes and synthesize their proteins. The same is not true for RNA viruses. Cellular organisms (except for plants) lack the enzymes needed to replicate RNA or to synthesize mRNA from an RNA genome. Therefore RNA viruses 23/10/18 9:12 am 6.3 Viral Life Cycles Have Five Steps 115 Spikes Capsid protein Nucleic acid Envelope Receptor Viral envelope spikes bind to receptors on surface of host cell. Lipid bilayer of viral envelope fuses with host cell membrane. Nucleocapsid is released into the cytoplasm. (a) Entry of enveloped virus by fusing with plasma membrane H+ Endosome Viral envelope spikes bind to receptors on the cell’s surface. Binding to the receptor triggers receptor-mediated endocytosis. Increased acidity allows nucleocapsid to escape from the endosome and enter the cytosol. (b) Entry of enveloped virus by endocytosis Capsid Nucleic acid Receptor Capsid proteins bind to receptors on cell surface and trigger receptor-mediated endocytosis. Nucleic acid is extruded from the endosome into the cytosol. (c) Entry of nonenveloped virus by endocytosis Figure 6.11 Animal Virus Entry. Examples of animal virus attachment and entry into host cells. Enveloped viruses can (a) enter after fusion of the envelope with the plasma membrane or (b) escape from an endosome after endocytosis. (c) Nonenveloped viruses may be taken up by endocytosis and then insert their nucleic acid into the cytosol through the vesicle membrane. It also is possible that they insert the nucleic acid directly through the plasma membrane. MICRO INQUIRY Which of these mechanisms involves the production of a vesicle within the host cell? Which involves the interaction between viral proteins and host cell membrane proteins? wil11886_ch06_106-127.indd 115 23/10/18 9:12 am 116 CHAPTER 6 | Viruses and Other Acellular Infectious Agents Membranes of endoplasmic reticulum Vesicles of viral replication complex Figure 6.12 A Viral Replication Complex. An electron tomogram of cells infected with severe acute respiratory syndrome coronavirus (SARS-CoV). The vesicles of the viral replication complex have a double membrane derived from endoplasmic reticulum (ER) membranes. The membranes of vesicles are connected to the ER. From “SARS-Coronavirus Replication Is Supported by a Reticulovesicular Network of Modified Endoplasmic Reticulum” Knoops K, Kikkert M, Worm SH, Zevenhoven-Dobbe JC, van der Meer Y, Koster AJ, Mommaas AM, Snijder EJ - (2008) must carry in their nucleocapsids the enzymes needed to complete the synthesis stage, or the enzymes must be synthesized during the infection process. This is discussed in more detail in chapter 26. Some animal and plant viruses carry out the synthesis stage and subsequent assembly step within the host’s cytoplasm. To protect these processes from host defenses, some viruses bring about the reorganization of host cell membranes (e.g., membranes of the endoplasmic reticulum, Golgi apparatus, and lysosomes) to form membranous structures that enclose the machineries needed for genome replication, transcription, and protein synthesis (figure 6.12). The structures are called viral replication complexes, and they appear as vesicles, tubular structures, and other forms in electron micrographs of infected cells. Other viruses carry out synthesis and assembly in defined areas within the cytoplasm that are not enclosed by membranes. These areas of concentrated viral genomes, mRNAs, and proteins are called viroplasms, and they are also visible in electron micrographs of infected cells. Both viral replication complexes and viroplasms are sometimes referred to as virus factories. One important feature of the synthesis stage is the tight regulation of gene expression and protein synthesis. Genes are often referred to as early, middle, or late genes based on when they are expressed. The proteins they encode are likewise referred to as early, middle, or late proteins. Many early proteins are involved in taking over the host cell. Middle proteins often participate in replication of the viral genome or activation of expression of late genes. Late proteins usually include capsid proteins and other proteins involved in self-assembly and release. wil11886_ch06_106-127.indd 116 Assembly Several kinds of late proteins are involved in the assembly of mature virions. Some are nucleocapsid proteins, some are not incorporated into the nucleocapsid but participate in its assembly, and still other late proteins are involved in virion release. In addition, proteins and other factors synthesized by the host may be involved in assembling mature virions. The assembly process can be quite complex with multiple subassembly lines functioning independently and converging in later steps to complete nucleocapsid construction. As shown in figure 6.13, the baseplate, tail fibers, and head components of bacteriophage T4 are assembled separately. Once the baseplate is finished, the tail tube is built on it and the sheath is assembled around the tube. The phages prohead (procapsid) is constructed with the aid of scaffolding proteins that are degraded or removed after assembly is completed. DNA is incorporated into the prohead by a complex of proteins sometimes called the “packasome.” The packasome consists of a protein called the portal protein, which is located at the base of the prohead, and an enzyme called terminase, which moves DNA into the prohead. The movement of DNA consumes energy in the form of ATP, which is supplied by the metabolic activity of the host bacterium. After the head is completed, it spontaneously combines with the tail assembly. Baseplate proteins Head proteins Prohead Baseplate DNA Mature head with DNA Tube Tube and sheath Whiskers and neck Long tail fiber proteins Collar Figure 6.13 The Assembly of T4 Virions. Note the subassembly lines for the baseplate, tail tube and sheath, long tail fibers, and head. 23/10/18 9:12 am 6.3 Viral Life Cycles Have Five Steps 117 Virion Release Virus-associated pyramids Several release mechanisms have been identified. The two most common are release by lysing the host cell and release by budding. Release by lysis is especially common for bacterial viruses and some nonenveloped animal viruses. This process involves the activity of viral proteins. For instance, lysis of E. coli by T4 requires two specific proteins (figure 6.14a). One is lysozyme, an enzyme that attacks peptidoglycan in the host’s cell wall. The other, called holin, creates holes in E. coli’s plasma membrane, enabling T4 lysozyme to move from the cytoplasm to the peptidoglycan. A novel release mechanism has been elucidated for some archaeal viruses infecting Sulfolobus spp. The virions are assembled in the cell cytoplasm, and a seven-sided pyramid structure forms near the cell envelope. These virus-associated pyramids puncture the envelope and open up much like the petals of a flower (figure 6.14b). This allows escape of the progeny virions, leaving the empty cell ghosts behind. Budding is frequently observed for enveloped viruses; in fact, envelope formation and virion release are usually concurrent processes. When virions are released by budding, the host cell may survive and continue releasing virions for some time. All envelopes of animal viruses are derived from host cell membranes by a multistep process. First, virus-encoded proteins are incorporated into the membrane. Then the nucleocapsid is simultaneously released and the envelope formed by membrane budding (figure 6.15). In several virus families, a matrix (M) protein attaches to the plasma membrane and aids in budding. Most envelopes arise from the plasma membrane. The endoplasmic reticulum, Golgi apparatus, and other internal membranes also can be used to form envelopes. Mechanism for Releasing Enveloped Virions (a) (b) Figure 6.14 Viral Release Mechanisms. (a) Release of T4 virions by lysis of the host cell. The host cell has been lysed (upper left portion of the cell) and virions have been released. Progeny virions also can be seen in the cytoplasm. Empty capsids coat the outside of the cell. (b) Sulfolobus solfataricus infected with Sulfolobus turreted icosahedral virus (STIV). Virions are released through pyramid structures that form on the cell surface. (a) ©Lee D. Simon/Science Source; (b) Courtesy of Susan Brumfield and Mark Young MICRO INQUIRY Why do the empty capsids remain attached to the cell after the viral genome enters the host cell? Budding has been observed as the archaeal SSV1 enveloped virus exits its host, Sulfolobus shibatae. Recall the structure of an archaeal cell envelope: a monolayer membrane with ether-linked lipids and a proteinaceous S-layer. Mature SSV1 particles Viral nucleocapsid Hemagglutinin Budding virion Viral matrix protein Viral envelope proteins are inserted into host cell's plasma membrane. The viral matrix protein lines the cytoplasmic face of the plasma membrane. Free infectious virion with envelope Neuraminidase Nucleocapsids (only one is shown) are directed to the plasma membrane by host cell's microtubules (not shown). Plasma membrane protrudes outward and nucleocapsids are surrounded by matrixlined plasma membrane. Neck of the protruding membrane is pinched off and a mature virion is released. Figure 6.15 Release of Influenza Virus Virions by Budding. For simplicity, only one of the seven to eight possible nucleocapsids is shown. wil11886_ch06_106-127.indd 117 23/10/18 9:13 am 118 CHAPTER 6 | Viruses and Other Acellular Infectious Agents covered in a host-derived membrane are initially trapped inside the S-layer but ultimately freed by structural alterations in the S-layer. Studies on the viral path through the cell envelope may reveal novel mechanisms for viral budding. There are many different types of archaeal cell walls (section 4.2) Interestingly, some viruses are not released from their host cell into the surrounding environment. Rather, their virions move from one host cell directly to another host cell. Most fungal viruses lack an extracellular phase in their replicative cycles. Instead they are transmitted by cell division, spore formation, or during mating. Vaccinia viruses elicit the formation of long actin tails that propel nucleocapsids through the plasma membrane, directly into an adjacent cell. In this way, the virus avoids detection by the host immune system. The genomes or nucleocapsids of many plant viruses also move directly from cell to cell through small connections called plasmodesmata that link adjacent cells. This spread of the virus typically involves virus-encoded movement proteins. The eukaryotic cytoplasm contains a cytoskeleton and organelles (section 5.3) Comprehension Check 1. Explain why the receptors that viruses have evolved to use are host surface proteins that serve very important, and sometimes essential, functions for the host cell. 2. What probably plays the most important role in determining the tissue and host specificity of viruses? Give some specific examples. 3. How do you think the complexity of the viral assembly process correlates with viral genome size? 4. In general, DNA viruses can be much more dependent on their host cells than can RNA viruses. Why is this so? 5. Consider the origin of viral envelopes and suggest why enveloped viruses that infect plants and bacteria are rare. 6. Why are the proteins involved in virion assembly synthesized late in the viral life cycle? 6.4 There Are Several Types of Viral Infections After reading this section, you should be able to: a. Compare and contrast the major steps of the life cycles of virulent phages and temperate phages b. List examples of lysogenic conversion c. Differentiate among the types of viral infections of eukaryotic cells d. Summarize the current understanding of how oncoviruses cause cancer So far, our discussion has focused on viral structures and modes of multiplication with little mention of the cost to host cells. The dependence of viruses on their host cells has many consequences—as anyone who has ever had a cold or the flu well understands. Next we discuss the interaction between virus and host cell. wil11886_ch06_106-127.indd 118 Lytic and Lysogenic Infections Are Common for Bacterial and Archaeal Cells Most bacteriophages are either virulent or temperate. A virulent phages is one that has only one option: to begin multiplying immediately upon entering its bacterial host, followed by release from the host by lysis. T4 is an example of a virulent phages. Temperate phages have two options: upon entry into the host, they can multiply like virulent phages and lyse the host cell, or they can remain within the host without destroying it (figure 6.16). Bacteriophage lambda is an example of this type of phages. The life cycles of both T4 and lambda are described in more detail in chapter 26. Steps in the Replication of T4 Phages in E. coli; Lambda Phages Replication Cycle The relationship between a temperate phages and its host is called lysogeny. The form of the virus that remains within its host is called a prophage. A prophage is simply the viral genome either integrated into the bacterial chromosome or free in the cytoplasm. The infected bacteria are called lysogens or lysogenic bacteria. Lysogenic bacteria reproduce and in most other ways appear to be perfectly normal. However, they have two distinct characteristics. The first is that they cannot be reinfected by the same virus; that is, they have immunity to superinfection. The second is that as they reproduce, the prophage is replicated and inherited by progeny cells. This can continue for many generations until conditions arise that cause the prophage to initiate synthesis of phages proteins and to assemble new virions, a process called induction. Induction is commonly caused by changes in growth conditions or ultraviolet irradiation of the host cell. As a result of induction, the lysogenic cycle ends and the lytic cycle commences; the host cell lyses and progeny phages particles are released. Another important outcome of lysogeny is lysogenic conversion. This occurs when a temperate phages changes the phenotype of its host. Lysogenic conversion often involves alteration in surface characteristics of the host. For example, when a member of the genus Salmonella is infected by epsilon phages, the phages changes the activities of several enzymes involved in construction of the carbohydrate component of the bacterium’s lipopolysaccharide. This eliminates the receptor for epsilon phage, so the bacterium becomes immune to infection by another epsilon phages. Other lysogenic conversions give the host pathogenic properties. This is the case when Corynebacterium diphtheriae, the cause of diphtheria, is infected with phages β. The phages genome encodes diphtheria toxin, which is responsible for the disease. Thus only those strains of C. diphtheriae that are infected by the phages (i.e., lysogens) cause disease. Diphtheria (section 39.1) Clearly the infection of a bacterium by a temperate phages has significant impact on the host, but why would viruses evolve this alternate cycle? Two advantages of lysogeny have been recognized. The first is that lysogeny allows the viral nucleic acid to be maintained within its host. Bacteria often become dormant due to nutrient deprivation, and while in this state, they do not synthesize nucleic acids or proteins. In such situations, a prophage would survive but most virulent bacteriophages would not be replicated, as they require active cellular biosynthetic machinery. 23/10/18 9:13 am 6.4 There Are Several Types of Viral Infections 119 Phage DNA Bacterial chromosome Phage injects its DNA into cytoplasm. New phages can bind to bacterial cells. Lytic cycle Cell lyses and releases the new phages. Exposure to stress such as UV light triggers excision from host chromosome. Lysogenic cycle Phage DNA directs the synthesis of many new phages. Phage DNA integrates into host chromosome. Prophage DNA is copied when cell divides. Prophage Figure 6.16 Lytic and Lysogenic Cycles of Temperate Phages. Temperate phages have two phases to their life cycles. The lysogenic cycle allows the genome of the virus to be replicated passively as the host cell’s genome is replicated. Certain environmental factors such as UV light can cause a switch from the lysogenic cycle to the lytic cycle. In the lytic cycle, new virus particles are made and released when the host cell lyses. Virulent phages are limited to just the lytic cycle. MICRO INQUIRY Why is a lysogen considered a new or different strain of a given bacterial species? Furthermore, their genome would be degraded as the host cell entered dormancy. The second advantage arises when there are many more phages in an environment than there are host cells, a situation virologists refer to as a high multiplicity of infection (MOI). In these conditions, lysogeny enables the survival of infected host cells within a population that has few uninfected cells. When MOI is high, a virulent phages would rapidly destroy the available host cells in its environment. However, a prophage will be replicated as the host cell reproduces. Archaeal viruses can also be virulent or temperate. In addition, many archaeal viruses establish chronic infections. Unfortunately, little is known about the mechanisms they use to regulate their replicative cycles. Comprehension Check 1. Define the terms lysogeny, temperate phages, lysogen, prophage, immunity, and induction. 2. What advantages might a phages gain by being capable of lysogeny? 3. Describe lysogenic conversion and its significance. Infections of Eukaryotic Cells Viruses can harm their eukaryotic host cells in many ways. An infection that results in cell death is a cytocidal infection. As with bacterial and archaeal viruses, this can occur by lysis of the host (figure 6.17a). Infection does not always result in lysis of host cells. Some viruses (e.g., herpesviruses) can establish persistent infections lasting many years (figure 6.17b,c). Eukaryotic viruses can cause wil11886_ch06_106-127.indd 119 microscopic or macroscopic degenerative changes or abnormalities in host cells and in tissues that are distinct from lysis. These are called cytopathic effects (CPEs). Viruses use a variety of mechanisms to cause cytopathic and cytocidal effects. Some important examples are noted in chapter 38. One mechanism of particular note is that some viruses cause the host cell to be transformed into a malignant cell (figure 6.17d). This is discussed next. Viruses and Cancer Cancer is one of the most serious medical problems in developed nations, and it is the focus of an immense amount of research. A tumor is a growth or lump of tissue resulting from neoplasia— unregulated abnormal new cell growth and reproduction. Tumor cells have aberrant shapes and altered plasma membranes that may contain distinctive molecules (tumor antigens). These changes result from the tumor cells becoming less differentiated. Their unregulated proliferation and loss of differentiation result in invasive growth that forms unorganized cell masses. Two major types of tumor growth patterns exist. If the tumor cells remain in place to form a compact mass, the tumor is benign. In contrast, cells from malignant or cancerous tumors actively spread throughout the body in a process known as metastasis. Some cancers are not solid but cell suspensions. For example, leukemias are composed of undifferentiated malignant white blood cells that circulate throughout the body. Indeed, dozens of kinds of cancers arise from a variety of cell types and afflict all kinds of organisms. Some viruses have been shown to cause cancer in animals, including humans; it is estimated that about 10 to 20% of human 23/10/18 9:13 am 120 CHAPTER 6 | Viruses and Other Acellular Infectious Agents Figure 6.17 Types of Viral Infections and Their Effects on Animal Cells. (a) Lytic infections of host cells can lead to disease states in animal hosts called acute infections. There are two types of persistent infections: (b) latent infections and (c) chronic infections. (d) Some infections of animal cells cause the cell to be transformed into a malignant cell, which can cause cancer in the animal host. Adsorption to host Penetration Rapid multiplication Activation of host proto-oncogene (human cancers), insertion of oncogene (cancers in other animals), or inactivation of tumor suppressor protein Cell death and virus release (a) Acute infection Viral components are present (e.g., viral genome); host is not harmed. Activation (b) Latent infection (c) Chronic infection Persistent infections cancers have a viral etiology. To understand the role viruses play in cancer, we must begin by considering carcinogenesis when viruses are not involved. Carcinogenesis is a complex, multistep process caused by mutations in multiple genes. Some mutations lead to the unregulated proliferation that is a major characteristic of a cancer cell. Other mutations prevent cells from repairing DNA damage, thereby making the cells more inclined to mutate further. This leads to additional genotypic changes. For example, mutations that promote metastasis may occur, allowing the tumor to invade other tissues. Mutations: heritable changes in a genome (section 16.1) Considerable research into the causes of cancer has focused on the mutations that allow cancerous cells to grow uncontrollably. Those wil11886_ch06_106-127.indd 120 Slow release of virus without cell death (d) Transformation into malignant cell studies show that there are two types of genes that must be mutated: proto-oncogenes and tumor suppressor genes. Their names suggest that these genes are somehow abnormal. But this is not the case. Proto-oncogenes and tumor suppressor genes are normal cellular genes. Proto-oncogenes must be expressed if cell division is to occur. However, they normally are expressed only if the cell receives an appropriate signal, such as the binding of a growth factor to a receptor on the cell surface. When such a signal is received, the cell initiates the cell cycle, but it cannot continue past a checkpoint controlled by the activity of proteins encoded by tumor suppressor genes. These proteins are called tumor suppressor proteins. When tumor suppressor proteins are active, 23/10/18 9:13 am 6.5 Cultivation and Enumeration of Viruses 121 they prevent progression through the cell cycle. Thus, for cell division to occur, proto-oncogene proteins (sometimes called protooncoproteins) must be active, and tumor suppressor proteins must be inactive. During carcinogenesis, mutations arise that disrupt the normal activity of these two types of genes. The first type of mutation allows proto-onocogenes or their protein products to function without an appropriate signal. Thus the proto-oncogene or its protein is active even when it should not be. The second type of mutation prevents expression of tumor suppressor genes, yields nonfunctional tumor suppressor proteins, or in some other way leads to inactivation of a tumor suppressor protein. Thus the cell can continue cell division even when it shouldn’t, such as in the presence of severe DNA damage. Numerous proto-oncogenes and tumor suppressor genes have been identified. An important protooncogene encodes a protein called Myc. Myc controls transcription of many genes, including some involved in DNA replication and other cell-cycle-related functions. Two of the most important tumor suppressor proteins are Rb and p53. The protein p53 is often referred to as “the guardian of the genome” because not only does it cause cell cycle arrest, but it also initiates programmed cell death in response to DNA damage. Programmed cell death, also called apoptosis, is a process that causes cells to kill themselves. Thus, when p53 is inactivated, the cell reproduces in an unregulated fashion, avoiding programmed cell death even when it should destroy itself. This is a catastrophic event for the cell because it can lead to the accumulation of additional mutations that contribute to carcinogenesis. Tumor suppressor genes We now turn our attention to viruses and their role in causing cancer. These viruses are called oncoviruses. As you can see in table 6.1, most human oncoviruses have dsDNA genomes, and most of these viruses cause cancer in a similar way—by interacting with and inactivating either Rb or p53. Oncoviruses that are retroviruses (e.g., HTLV-1) exert their oncogenic powers in a different manner. Some carry genes called oncogenes that stimulate the activity of cellular proto-oncogenes. For example, HTLV-1 infects immune system cells called T cells. During infection HTLV-1 produces a regulatory protein that activates expression of numerous cellular proto-oncogenes. Other retroviruses transform cells into cancerous Table 6.1 wil11886_ch06_106-127.indd 121 cells when the viral genome integrates into the host chromosome such that strong, viral regulatory elements are near a cellular protooncogene. These elements cause the nearby proto-oncogene to be transcribed at a high level, causing the cellular gene to be considered an oncogene. Comprehension Check 1. How does a latent infection differ from a chronic infection? 2. What is a cytocidal infection? What is a cytopathic effect? 3. Define the following terms: tumor, neoplasia, anaplasia, metastasis, proto-oncogene, oncogene, and tumor suppressor gene. 4. Distinguish the mechanism by which dsDNA viruses cause cancer from that of retroviruses. Why do you think there is an environmental component to many kinds of cancer? 6.5 Cultivation and Enumeration of Viruses After reading this section, you should be able to: a. List the approaches used to cult