MBS1202_Study.docx
Document Details
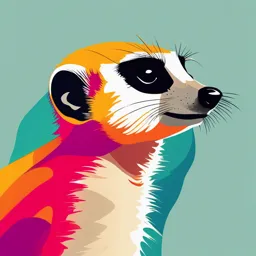
Uploaded by HandyConflict
Affiliated Hospital of Nanjing University of Chinese Medicine
Full Transcript
First week (deadline): We need to make both multiple-choice questions and open questions Week 1; Introduction Lecture: Week 1; Expert Lecture: Case 1 1. What are Biosimilars? Definition: Biosimilars are medications that are structurally and functionally similar to biologics, which are medicines made...
First week (deadline): We need to make both multiple-choice questions and open questions Week 1; Introduction Lecture: Week 1; Expert Lecture: Case 1 1. What are Biosimilars? Definition: Biosimilars are medications that are structurally and functionally similar to biologics, which are medicines made in a living system and used in the treatment of diseases. Biosimilars are developed to be highly similar but not identical to the original product aiming to be safe and effective. Biosimilars are approved by demonstrating their high similarity to an FDA-approved biologic reference product, showing no clinically meaningful difference in safety and effectiveness → The only difference is that biosimilars may have minor differences in clinically inactive components. The development process involves extensive analysis to confirm they have the same characteristics as the reference biologics, including: Purity Molecular structure Bioactivity Biosimilars must undergo FDA approval, including clinical trials, to ensure they are as safe and effective as the brand name biologics they are designed to replace. 1.1 Criteria to be considered a biosimilar: To be considered a biosimilar, a drug must meet several criteria established by regulatory bodies like the FDA, EMA, and WHO. These criteria ensure the biosimilar is highly similar to the reference product (RP) in terms of structure, function, and clinical effectiveness. The key criteria includes: Structural and Functional Similarity: The biosimilar needs to closely resemble the reference product in both structure and function, confirmed through thorough structural and functional analysis. Clinical Similarity: There should be no significant differences in purity, potency, and safety between the biosimilar and the reference product, requiring comparative clinical pharmacology studies. Comparative Bioequivalence Study: A comparative bioequivalence study involving pharmacokinetic (PK) and/or pharmacodynamic (PD) comparability is necessary to validate the similar safety and effectiveness of both the biosimilar and the reference product. Clinical Trial: Comparative clinical trials are carried out to verify that there are no clinically significant differences between the proposed biosimilar and the reference product. Nonclinical Trial: Depending on the local regulatory authority, an in vivo nonclinical evaluation may be required, including in vitro studies and, if necessary, in vivo animal studies to assess the safety and efficacy of the biosimilar. The FDA approves biosimilars through an abbreviated pathway, aiming to demonstrate biosimilarity between the proposed biosimilar and its reference product without independently establishing the safety and effectiveness of the proposed biosimilar. - This process helps reduce the time and cost of development without compromising safety and effectiveness. The Biologics Price Competition and Innovation Act of 2009 created this abbreviated approval pathway to provide patients with greater access to safe and effective biological products. An application for a proposed biosimilar generally must include information showing that the proposed product is biosimilar to a reference product → This includes analytical studies that provide the foundation of biosimilar development, supporting the structural and functional similarity of the proposed product to the reference product and evaluating the impact of any differences identified. Animal studies may provide toxicology or pharmacology information, and clinical studies may demonstrate that the proposed biosimilar moves through the body in the same way and provides the same effects as the reference product. An interchangeable biosimilar is a biosimilar that may be substituted for the reference product without the intervention of the prescribing health care provider, depending on state pharmacy laws. The approval process for interchangeable biosimilars has additional requirements related to the potential for substitution, including information on the effects of switching between the reference product and the interchangeable biosimilar. 1.2 How do biosimilars differ from the original drug? Biosimilars differ from the original drug (reference product) in several key aspects: Production Method: Biosimilars are produced using specialized biological processes involving living systems, unlike generic drugs which are chemically synthesized. This biological manufacturing process inherently leads to variation within and between lots, which is a natural aspect of the process. Structural and Functional Similarity: Biosimilars are highly similar to the reference product in terms of their primary amino acid sequence and mechanism of action. However, due to the biological manufacturing process, there are minor differences in clinically inactive components. The FDA requires biosimilar manufacturers to demonstrate that there are no clinically meaningful differences between the biosimilar and the reference product in terms of safety, purity, and potency. Immunogenicity: Biosimilars have a higher risk for immunogenicity compared to generic drugs. This is because the biological manufacturing process can lead to variations in the protein molecule, which may trigger immune responses. Immunological responses can include infusion reactions, neutralizing antibodies, and reduced efficacy. These reactions may occur months after initiation, making it important for clinicians to monitor and report suspected immunological responses. The approval process for biosimilars is different from that of generic drugs. Biosimilar manufacturers must demonstrate that the biosimilar is highly similar to the reference product, except for minor differences in clinically inactive components. They must also show that there are no clinically meaningful differences between the biosimilar and the reference product in terms of safety, purity, and potency: This requires more extensive data generation and evaluation compared to generic drugs, which can be approved through an abbreviated pathway. Biosimilars are generally more expensive to develop than generic drugs due to the complexity of the biological manufacturing process and the need for extensive testing. However, they offer the potential for cost containment and affordability compared to the original drug, especially after the patent expiration of the reference product. This can make biosimilars a more attractive option for patients, especially in areas where the original drug is expensive. The importance of generating biosimilars lies in their potential to provide patients with more treatment options, increase access to lifesaving medications, and potentially lower health care costs through competition. Biosimilars approved by the FDA are safe and effective biological medications, contributing to the diversity of treatment options available to patients. 1.3 Olizumab origin and structure: Omalizumab is a recombinant DNA-derived humanized and glycosylated IgG1 monoclonal antibody that targets human immunoglobulin E (IgE) and is produced by Chinese hamster ovary (CHO) cells. It consists of a human IgG1 Fc fragment and a mouse MAE 11 binding domain. The pharmacokinetics of omalizumab might indeed be affected by its Fc glycosylation, as glycosylation can significantly impact the pharmacokinetics (PK) of monoclonal antibodies (mAbs) and Fc-fusion proteins. Glycosylation is a common modification in therapeutic mAbs and Fc-fusion proteins, including omalizumab. It involves the attachment of carbohydrate molecules to the antibody, which can influence its distribution, metabolism, and clearance in the body. For instance, glycosylation can affect the interaction of the antibody with Fc receptors (FcRn), which plays a crucial role in the recycling of the antibody. This recycling can influence the half-life of the antibody, with glycosylation potentially affecting the rate at which the antibody is cleared from the body. Moreover, the charge of the antibody can also impact its PK, with variants having pI values of 1–2 unit difference likely to impact PK, with lower pI values being favorable for a longer half-life. This is because the charge can influence the antibody's interaction with Fc receptors and its distribution in the body. Omalizumab, being glycosylated, is likely to exhibit a similar PK profile to other glycosylated mAbs. However, the specific impact of its glycosylation on its PK would depend on the glycosylation pattern and the presence of any additional modifications. For example, some therapeutic mAbs bear additional glycosylation in the Fab domain, which can further influence their PK. In summary, the glycosylation of omalizumab, like other glycosylated mAbs, can significantly affect its pharmacokinetics, influencing its distribution, metabolism, and clearance in the body. This can have implications for the therapeutic efficacy and safety of the drug, requiring careful consideration during its development and clinical evaluation. 1.4 Omalizumab effector mechanism: Elevated total IgE levels are observed in various allergic and some non-allergic diseases. The molecular structure of IgE is complex, consisting of two identical light chains, each with a variable (VL) and a constant (CL) domain, paired with two identical heavy chains. Each heavy chain includes a variable portion (VH) and a constant fragment (Cε1, Cε2, Cε3, Cε4). The variable fragments (Fab) of IgE interact with specific antigens, while the constant region (Fc) binds to IgE receptors. In allergic diseases, the pathogenic role of IgE is attributed to its binding to high-affinity (FcεRI) and low-affinity (FcεRII/CD23) receptors expressed by many cells. The FcεRI receptor is a high-affinity receptor that can bind IgE and mediate its effector functions, such as antibody-dependent cell-mediated cytotoxicity (ADCC). The FcεRII/CD23 receptor is a low-affinity receptor that can also bind IgE but is less involved in ADCC. The binding of IgE to these receptors is allosterically regulated, meaning the binding of IgE can influence the receptor's activity and the receptor can influence IgE's binding and function. The crystal structures of IgE-Fc in complex with anti-IgE Fab arms and the schematic representations of FcεRI and CD23 provide insights into the molecular basis of IgE's interaction with its receptors. These structures are crucial for understanding how IgE's structure and glycosylation affect its binding to receptors and its role in allergic and potentially non-allergic diseases. IgE's effector functions, including its role in cancer immunotherapy, are also discussed, highlighting the importance of IgE in mediating immune responses against cancer cells. The interaction of IgE with its receptors and the cross-linking of IgE by soluble allergens are key mechanisms in the pathogenesis of allergic diseases and the potential for IgE to function against cancer cells. In summary, the structure of IgE, including its variable and constant domains, and its interaction with specific receptors, play a critical role in the pathogenesis of allergic and some non-allergic diseases. The detailed study of IgE's molecular structure and its interaction with its receptors is essential for understanding its role in immune responses and for the development of therapeutic strategies targeting IgE. Summary: Omalizumab is a humanized monoclonal antibody that specifically targets the Fc portion of the IgE molecule, particularly the two Cε3 domains. This binding forms trimeric or hexameric IgE/anti-IgE immune complexes. These complexes are soluble and do not bind complement, making them easily removable by the mononuclear phagocytic system. The Cε3 domain is crucial for IgE's interaction with high-affinity receptors (FcεRI) and low-affinity receptors (FcεRII/CD23), which are primarily found on eosinophils, basophils, and mast cells. By binding to IgE, omalizumab effectively lowers immunoglobulin levels and prevents them from interacting with these high-affinity receptors, thereby disrupting the allergic cascade. The stability of IgE/omalizumab aggregates is attributed to the high affinity of omalizumab for IgE. This stability prevents the aggregates from crossing vascular walls and accumulating in peripheral blood or mucosal membranes of the upper and lower airways. The total reduction of free IgE leads to decreased expression of the IgE high-affinity receptor on inflammatory cells and a reduction in peripheral eosinophilia. This mechanism of action of omalizumab is crucial for inhibiting all IgE-dependent cellular events, including mast cell degranulation, basophil expression of high-affinity IgE receptors, facilitation of antigen presentation to T-cells, eosinophil survival and IgE synthesis, as well as the release of growth factors from airway epithelial cells and production of extracellular matrix proteins by airway smooth muscle cells. These effects collectively result in a clinically significant inhibition of allergic inflammation and airway remodeling. Omalizumab's mechanism of action is based on its competitive interaction with IgE at the Cε3 domains. To be effective, omalizumab must overcome IgE numbers in a ratio within a range of 7:1–15:1. Proper dosing is essential, as it needs to be based on both blood IgE levels and body weight. Correct dosing can induce an 84–99% reduction in serum free IgE concentration after a single subcutaneous injection. In summary, omalizumab's specific binding to the Cε3 domains of IgE and the formation of stable immune complexes play a pivotal role in preventing the allergic cascade by blocking IgE's interaction with its high-affinity receptors. This mechanism not only inhibits allergic inflammation and airway remodeling but also contributes to a better understanding of the Th2 pathway and the concept of T2 inflammation. 2.5 Modification of Omalizumab: Omalizumab, a humanized monoclonal antibody used for treating allergic diseases, faces challenges such as chemical instability due to aspartic acid-glycine (Asp-Gly) sequences, potential immunogenicity risks from murine amino acids in the framework region, and limitations in clinical application based on body weight and serum total IgE level. To address these issues, modifications have been made to improve omalizumab's stability, reduce immunogenicity, and extend its half-life. These modifications include removing aspartic acid isomerization hotspots in the complementarity-determining regions (CDRs) to enhance chemical stability and replacing murine amino acids in the framework region with human equivalents to minimize immunogenicity risks. Additionally, affinity maturation using yeast display technology and Fc engineering, such as the M252Y/S254T/T256E(YTE) mutation, have been applied to increase the antibody's affinity to IgE and extend its half-life. A modified version, AB1904Am15, has shown greater stability and a longer half-life compared to other candidates, making it a promising lead for further study. 2.1 How to produce monoclonal antibodies (hybridoma technology)? Hybridoma technology is a widely used method for producing monoclonal antibodies (mAbs), which are highly specific and pure antibodies derived from a single B-cell. This technology begins with the immunization of laboratory animals, such as mice, with a chosen antigen through multiple injections over several weeks. This stimulates B-cell differentiation into plasma B-cells and memory B-cells. After the animal is sacrificed, the spleen is removed under aseptic conditions to isolate activated B-cells. These cells are then fused with immortal myeloma cell lines to form hybridoma cell lines, which can produce mAbs against a specific antigen. The fusion process can be facilitated by polyethylene glycol (PEG) or electrofusion, allowing the merging of the plasma membranes of B-cells and myeloma cells to create hybrid cells with multiple nuclei. Post-fusion, hybridoma cells are selected in HAT medium, which inhibits unfused cells' nucleotide synthesis while allowing hybrid cells with functional HGPRT genes from B-lymphocytes to thrive and divide. The process continues with the screening of hybridoma cells for desired antibody production against specific epitopes, followed by cloning and propagation of selected hybridomas. This can be achieved through in vivo methods involving mice or in vitro methods in controlled lab conditions to mitigate contamination risks. Hybridoma technology offers several advantages, including the production of highly pure and specific antibodies, high reproducibility and scalability, unlimited production of mAbs, and the ability to perform highly sensitive and specific assays. It is not labor-intensive and does not require the maintenance of animals in the laboratory for antibody production. The technology is widely used in various fields such as toxicology, animal biotechnology, medicine, pharmacology, and more, for diagnostic and therapeutic procedures, chemotherapeutic regimens, and vaccine production. 2.2. Structural characteristics and origin of drug: Evolocumab Evolocumab, marketed as Repatha®, is a human monoclonal immunoglobulin G2 (IgG2) lambda antibody that specifically targets the proprotein convertase subtilisin/kexin type 9 (PCSK9). PCSK9 is a protein produced by the liver that binds to low-density lipoprotein (LDL) receptors, facilitating their degradation and thus reducing the liver's ability to remove LDL cholesterol (LDL-C) from the blood. Evolocumab binds to PCSK9 and inhibits its ability to bind to LDL receptors on the liver surface, which results in an increase in LDL receptors on the liver surface, enhancing the removal of LDL-C from the blood. This mechanism makes Evolocumab effective in reducing LDL cholesterol levels in patients with conditions like familial hypercholesterolemia or those requiring additional LDL-C lowering beyond what can be achieved with diet and statin therapy alone. Evolocumab is a human IgG type 2 antibody, characterized by its shorter hinge and higher rigidity compared to other antibody types, which contributes to its less flexible structure. It has an effective half-life of 11-17 days and is developed and manufactured using recombinant DNA technology. It is indicated for the treatment of hyperlipidemia, particularly in patients with heterozygous familial hypercholesterolemia (HeFH) and clinical atherosclerotic cardiovascular disease (ASCVD), who require lowering of LDL-C levels. Evolocumab is administered as a subcutaneous injection and is approved for use in adults with established cardiovascular disease to reduce the risk of myocardial infarction, stroke, and coronary revascularization, as well as in adults with primary hyperlipidemia or in pediatric patients with heterozygous familial hypercholesterolemia to reduce LDL-C levels. 2.3. Working mechanism of the therapeutic antibody. Where does the target play a role in the disease? Cardiovascular diseases are a leading cause of morbidity and mortality worldwide, with low-density lipoprotein cholesterol (LDL-C) levels playing a significant role in their development. Monoclonal antibodies (mAbs) like Evolocumab (Repatha®) offer a solution for patients who cannot tolerate lipid-lowering agents such as statins. Evolocumab is a human monoclonal immunoglobulin G2 (IgG2) lambda antibody that targets the proprotein convertase subtilisin/kexin type 9 (PCSK9), a protein that regulates the expression of LDL-Rs on hepatic cells, leading to increased plasma LDL-C levels. By binding to PCSK9, Evolocumab prevents the internalization and degradation of LDL-Rs, thereby increasing their availability on the cell surface. This action leads to lower levels of LDL-C in the blood, making it effective in reducing LDL cholesterol in patients with conditions like familial hypercholesterolemia or those requiring additional LDL-C lowering beyond diet and statin therapy alone. The mechanism of action involves the recycling of LDL-Rs to the surface of normal hepatic cells, which regulates plasma LDL-C levels. PCSK9 binds to the epidermal growth factor-like repeat A (EGF-A) domain of LDL-Rs, stimulating their internalization and lysosomal degradation, reducing the number of receptors on the cell membrane and thus increasing plasma LDL-C levels. By blocking the interaction of PCSK9 with LDL-Rs, Evolocumab prevents this process, leading to an increase in LDL-Rs on the cell surface and a decrease in plasma LDL-C levels. This targeted therapy has been shown to be effective in lowering LDL-C levels in patients with hypercholesterolemia, making it a valuable treatment option for those who cannot tolerate traditional lipid-lowering agents. 2.4. Modifications to the antibody (biosimilar): how can we enhance their efficacy To enhance the efficacy of antibodies, several modification strategies are employed, including limiting hinge flexibility to increase binding affinity, increasing the half-life of antibodies, and using only the Fab fragment of the antibody to mitigate side effects. The clearance of IgG antibodies depends on the rate of fluid-phase pinocytosis and their binding affinity to the neonatal Fc receptor (FcRn). Mechanisms to increase antibody half-life include PEG modification, decreasing the pI of the variable region, and increasing the affinity to FcRn. PEG modifications increase hydrophilicity and reduce glomerular filtration, while decreasing the pI of the variable region reduces the rate of fluid phase pinocytosis. Increasing the affinity to FcRn can be achieved through substitution mutations in the constant region of the antibody, such as T250Q/M428L or M252Y/S254T/T256E, which can improve effector functions but may increase immunogenicity. Glycosylation is another post-translational modification that can influence antibody efficacy. It involves attaching glycans to specific amino acid residues in proteins, which can stabilize the antibody, increase binding affinity, and conceal antigenic epitopes, thereby decreasing immunogenicity. For example, Evocolumab has a N-linked glycan added to each heavy chain on asparagine 291, altering its effector functions and increasing its ability to lower LDL-C levels in the blood. In summary, antibody modifications aim to enhance binding affinity, increase half-life, and reduce immunogenicity, making them more effective and safer for therapeutic use. These modifications include PEG modification, decreasing the pI of the variable region, increasing FcRn affinity, using only the Fab fragment, and glycosylation, each contributing to the overall efficacy and safety of the antibody. 3.1 How do you produce monoclonal antibodies with the recombinant technique? Recombinant technique for producing monoclonal antibodies involves genetically engineering antibody-encoding DNA sequences, which are then inserted into host cells, such as mammalian cells, to produce the antibody. This process begins with obtaining the antibody-encoding DNA sequences, which can be achieved through various methods, including mass spectrometry and transcriptomic shotgun sequencing in hybridoma cells. These sequences are modified to optimize the antibody structure and are introduced into plasmids, which are then used to transfect mammalian cells, such as Chinese hamster ovary (CHO) cells. The signal peptide sequence is crucial for antibody secretion, and instead of encoding the entire heavy or light chain, only the variable region is inserted adjacent to the parent plasmid’s constant region. This approach typically involves two separate plasmids, one for the heavy chain and one for the light chain. After transfection, the antibody-encoding DNA is confirmed to be sufficiently translated into monoclonal antibodies, usually through ELISA on the cell media. Finally, the monoclonal antibody is purified for downstream applications, such as using a protein A Sepharose column to bind IgG antibodies. This method offers several advantages over traditional bacterial expression, including the requirement for glycosylation for antibody functionality and significantly reduced levels of endotoxin contamination. Advances in transient mammalian expression systems enable high yields, allowing for effective recombinant antibody production at a reasonable cost. The process involves designing antibody constructs by combining antibody variable domains with constant regions, expressing these constructs from mammalian vectors, secretion into culture media, purification by affinity chromatography, and characterization by biolayer interferometry. This approach allows for the expression and purification of endotoxin-free antibody reagents suitable for testing in animal models within a 3-week timeframe. 3.2 What are the structural characteristics and origin of Infliximab Structure and characteristics: Infliximab is a bivalent, monospecific IgG1k monoclonal antibody that targets tumor necrosis factor-alpha (TNF-α). It is a chimeric antibody, composed of 75% human-derived constant regions and 25% murine-derived variable regions. The antibody consists of two light chains (kappa) and two heavy chains, which are connected by disulfide bonds. The glycosylation pattern of the Fc region plays a crucial role in the antibody's effector mechanisms. TNF-α typically forms trimer complexes, and infliximab's Fab region interacts with a single TNF-α monomer within these trimer complexes. This interaction allows for the formation of higher-order antigen-antibody structures. For infliximab to effectively recognize TNF-α, all six complementary determining regions (CDRs) are required. These CDRs, derived from the murine variable region, show minimal conformational changes upon binding to the antigen. This specificity and the requirement for all CDRs to be engaged for effective antigen recognition are key to infliximab's therapeutic efficacy in treating various inflammatory conditions. 3.3 What are the working mechanisms of the therapeutic antibody? Where does the target play a role in the disease? Production: The creation of infliximab antibodies involves a two-step process: the initial development of a murine A2 hybridoma and the subsequent plasmid construction and SP2/0 transfection for chimeric antibody production. Murine A2 Hybridoma Development: This step begins with immunizing a mouse with injections containing recombinant human TNF-α, accompanied by an adjuvant. This process results in spleen cells that produce anti-TNF-α antibodies. These spleen cells are then fused with SP2/0 cells (mouse myeloma cells) to create hybridoma cells. The production of antibodies against the antigen is verified through radioimmunoassay, and the monoclonal antibody is purified from the hybridoma's supernatant using Protein A-Sepharose. Plasmid Construction and SP2/0 Transfection: Plasmids encoding the heavy chain or light chain are constructed, incorporating DNA sequences from the murine A2 hybridoma's variable genes along with DNA encoding human constant regions. These plasmids are then transfected into SP2/0 cells to produce chimeric antibodies against human TNF-α. This procedure leverages the specificity of the murine A2 hybridoma for human TNF-α and the ability of SP2/0 cells to produce large quantities of the chimeric antibody, facilitating the development of infliximab, a therapeutic monoclonal antibody used in the treatment of various inflammatory conditions. Working mechanism: Infliximab, a monoclonal antibody against tumor necrosis factor-alpha (TNF-α), is highly effective in the clinical management of inflammatory bowel disease (IBD), including Crohn's disease and ulcerative colitis. These conditions are characterized by chronic relapsing intestinal inflammation, thought to result from an aberrant immune response to gut microbes, influenced by individual genetic susceptibility. The inflammatory intestinal microenvironment is mediated by the production of proinflammatory cytokines like IL-1β, IL-6, and TNF-α by various cells, including intestinal epithelial cells, dendritic cells (DCs), and macrophages. Infliximab targets both soluble and transmembrane forms of TNF-α, a potent proinflammatory cytokine implicated in the dysregulation of the mucosal immune response in IBD. TNF-α has two receptors: TNFRI, which binds soluble TNF and activates a caspase-8-dependent cell death signaling pathway; and TNFRII, which binds membrane TNF (mTNF) and activates pro-survival and pro-inflammatory signaling pathways. By neutralizing TNF-α, infliximab helps suppress inflammation in the intestinal mucosa. Two major alternative mechanisms of action for infliximab include: An anti-apoptotic signal by mTNF, expressed on monocytes, to the TNFRII expressed on CD4+ T cells. This mechanism is inhibited by anti-TNFs, leading to apoptosis of CD4+ cells. Anti-TNFs bind to mTNF on activated T cells; the Fc part of the antibody is recognized by Fc receptors expressed by monocytes, triggering their differentiation to an M2-like or wound-healing phenotype. These mechanisms contribute to the full clinical, biochemical, and endoscopic response observed in patients treated with infliximab. The rapid induction of T cell apoptosis and the specific induction of M2-type wound-healing macrophages in responders to infliximab are key observations that may relate to these distinct mechanisms. 3.4 Modification to the antibody (biosimilar): How can we enhance their efficacy? The development of biosimilars, such as Remsima, a biosimilar of infliximab, aims to provide a cost-effective alternative to the original biologic drug. While the primary amino acid sequence of a biosimilar is precisely engineered to match the original drug, other structural features like three-dimensional folding, glycosylation, charge, and impurity presence can vary due to the manufacturing process. These variations can impact the antigen binding and immunogenicity of the drug, thereby affecting its efficacy and safety. To improve the efficacy of infliximab, modifications such as PEGylation can be applied. PEGylation reduces enzymatic degradation, helping to increase the half-life of the antibody. Although the central amino acid sequence cannot be changed, differences in terminal amino acid sequences and post-translational modifications can be introduced to enhance stability. The development of Remsima as a biosimilar of infliximab in 2013 demonstrated that it is possible to create a drug with comparable safety, immunogenicity, and pharmacokinetics to the original. The transition from infliximab to Remsima did not lead to disease worsening, and the monomer and aggregate contents of Remsima were found to be comparable to those of infliximab. Although Remsima contained slightly less basic variants than infliximab, this difference was largely attributed to the presence of C-terminal lysine. These findings highlight the potential of biosimilars to provide a cost-effective alternative to biologic drugs while maintaining comparable efficacy and safety profiles. The development and approval of biosimilars like Remsima represent significant advancements in the field of drug development, offering a promising solution to the financial challenges associated with the use of biologic drugs. 4.1 What steps are needed to commercialize biosimilars? Pre-clinical trials and clinical trials Biosimilars are biologically similar to commercially approved drugs, making them an attractive investment due to their cost-effective development process. Unlike new drugs, biosimilars can use the commercial drug as a reference for their own commercial approval. The European Medicines Agency (EMA) and the FDA require biosimilars to be similar to their commercial reference drugs in terms of efficacy, safety, and biological activation. The FDA also mandates interchangeability between the biosimilar and the reference drug, a requirement not uniformly enforced across European countries. To ensure the safety and efficacy of biosimilars, referential studies comparing the biosimilar to its commercial counterpart are conducted, covering pharmacodynamics, pharmacokinetics, functional and structural characteristics, toxicity assessments, and safety windows. The approval process for biosimilars is less rigorous than for new drugs, with a greater emphasis on comparative studies between the biosimilar and the reference drug. The biosimilar must have the same dosage and administered amount as the reference drug, and its functionality and safety intervals must be comparable. The production process of biosimilars is more complex than chemical synthesis but yields more market value due to the difficulty in producing generic copies. The company producing the biosimilar must inform the EMA 6-18 months prior to the submission of the report, which contains evidence of pre-clinical and clinical results obtained by comparing the biosimilar drug with a commercial reference. The EMA then analyzes the report and approves or disapproves the biosimilar based on its findings. 4.2 How does it differ from the original drug process? Compared with the approval pathway for a new biological, the biosimilarity exercise places more emphasis on data from the extensive physicochemical and biological characterisation of the candidate and the comparative analytical testing with the reference product and less on those from clinical trials. The nature and extent of each step of the clinical development depends on the level of evidence obtained in the previous steps of the comparability exercise. The clinical package generally consists of a phase 1 study followed by at least one phase 3 study for one of the approved indications of the reference product. In some cases, confirmatory PK and pharmacodynamic (PD) studies might be sufficient to demonstrate clinical biosimilarity. At the end of the process, the biosimilar is evaluated on the overall body of evidence for biosimilarity. The figure below provides a schematic overview of the differences in approach between the development of a new biological and a biosimilar. → Original drug commercialisation consists of 5 steps, which are shown in the figure below → Biosimilars: The process of commercialising a biosimilar is much more focused on similarity studies in their physicochemical and functional properties against the reference product. Because biosimilar development leverages the scientific evidence established by the reference product, initial development steps involving target identification and validation are eliminated, decreasing the risk of failures and the development time. Provided that manufacturers can demonstrate comparability, biosimilars can be developed with less time, investment, and risks than is associated with standard biologic development pathways. 4.3 Structural characteristics and origin of Trastuzumab: Trastuzumab (Herceptin) is a humanized IgG1 kappa monoclonal antibody Used to treat HER2-positive breast cancer, gastroesophageal, and gastric cancer It is produced by recombinant DNA technology in mammalian cell (Chinese Hamster ovary) culture 4.4 Working mechanisms of the therapeutic antibody. Where does the target play a role in the disease? Trastuzumab is a humanized monoclonal antibody against the extracellular domain IV of the human epidermal growth factor receptor 2 (HER2) HER2 is part of the family of epidermal growth factor receptors (EGFR), comprising HER1, HER2, HER3m, and HER4 → are tyrosine kinases and play a role in cellular growth and are up-regulated in numerous cancers including breast cancer (breast carcinoma) HER2 is expressed at a low level on the surface of epithelial cells and is necessary for the normal development of many tissues In breast cancer, there is a high level of HER2 → overexpression of HER2 is important because its activation triggers multiple downstream pathways required for the abnormal proliferation of cancer cells Activation of HER2: Formation of homodimers or heterodimers with other EGFR proteins. This dimerization leads to autophosphorylation and/or transphosphorylation of specific tyrosine residues in EGFR intracellular domains, which in turn leads to the activation of RAS/RAF/mitogen-activated protein kinase, the phosphoinositide 3-kinase/Akt, and the phospholipase Cγ (PLCγ)/protein kinase C (PKC) pathways HER2-HER3 heterodimer is the most potent stimulator of downstream pathways, particularly the PI3K/Akt, a master regulator of cell growth and survival The molecular mechanisms of actions of Trastuzumab are grouped into three major categories: 1. HER2 degradation Trastuzumab has been proposed to trigger HER2 internalization and degradation through promoting the activity of tyrosine kinase – ubiquitin ligase c-Cbl. It was observed that the binding of trastuzumab to HER2 recruits c-Cbl to its docking site, Tyr1112, where c-Cbl ubiquitinates HER2 and leads to its degradation. 2. Antibody-dependent cellular cytotoxicity As an antibody, one of the major mechanisms of trastuzumab is to attract immune cells to tumour sites that overexpress HER2, by a mechanism called antibody-dependent cellular cytotoxicity (ADCC). Research has also proven that NK cells can target HER2-overexpressing cells coated with trastuzumab via a CD16-mediated ADCC mechanism. This helps explain why cancer cells with high HER2 expression are more responsive to the drug. 3. MAPK and PI3K/Akt interference The most well known effect of trastuzumab is the inhibition of the MAPK and PI3K/Akt pathways, which leads to an increase in cell cycle arrest, and the suppression of cell growth and proliferation. It is widely accepted that by interfering with the dimerization of HER2, trastuzumab inhibits HER2 activation and suppresses Akt phosphorylation. In general, trastuzumab, by binding to the extracellular domain of HER2, can potently suppress cancer cells growth, proliferation, and survival in both direct and indirect manners. 4.5 Modifications to the antibody (biosimilar): how can we enhance their efficacy? In order to promote binding affinity, half-life in circulation, effectiveness toward target cells and, ultimately, to achieve overall improved efficacy, certain modifications could be induced. Common modification approaches to modify therapeutic antibodies: (a) Modified glycosylation Fc glycosylation plays a crucial role in mediating the interaction between antibodies and Fcγ receptors (FcγRs) on immune cells, such as natural killer (NK) cells, which is essential for triggering ADCC. Specifically, the presence or absence of fucose residues at the Asn297 glycosylation site of the Fc region can influence ADCC activity. Therefore, to enhance ADCC activity, modifying glycosylation can be considered. (b) Fc amino acid alterations Fc amino acid alterations can change the affinity and effector functions of target cells and the half-life of antibodies. (c) Cross-isotype or cross-subclass exchanges A cross-isotype antibody can integrate different effector functions elucidated by different isotypes of immunoglobulins. This category of modified antibodies aims to enhance ADCC and/or CDC by recombining and/or replacing sections of natural Fc domains with those from different isotypes/subclasses to form cross-isotype antibodies. (d) Antibody–drug conjugates (ADCs) An ADC is a specific antibody-guidance-based cytotoxic drug. Monoclonal antibodies (mAbs) can be conjugated with various effector molecules, such as cytotoxic agents, radiopharmaceuticals, and immunotoxins, to generate a new pattern of target therapy: ADCs. (e) Single chain of variable region fragment (scFv) for chimeric antigen receptor T (CAR-T) cells Autologous T cells from patients are genetically engineered to express CARs and are termed as CAR-T cells; they are subsequently infused back into the patients to recognize the specific antigens and kill the antigen-expressing cells, thereby serving as a cellular therapy. (f) Bispecific antibodies (bsAbs) bsAbs refer to a large family of recombinant molecules that are designed to recognize two disease-related targets or distinct epitopes within one molecule, which breaks up the natural IgG architectural format, since inhibiting a single target may fail to achieve significant efficacy. Some examples of applying these strategies to Trastuzumab: For Trastuzumab specifically, an example would be modifying its Fc region. This could enhance its binding affinity to Fc receptors on immune cells such as natural killer cells and macrophages, leading to improved ADCC, one of the mechanisms of action for Trastuzumab. Antibody-drug conjugates (ADCs), involves conjugating monoclonal antibodies like trastuzumab with cytotoxic drugs to create a targeted therapeutic agent. This approach combines the specificity of the antibody for HER2 with the cytotoxic effects of the drug, resulting in selective killing of cancer cells while sparing healthy tissues. Designing a bispecific antibody that targets both HER2 and another antigen or receptor on cancer cells can improve efficacy. Trastuzumab's HER2-binding domain can be retained in the bispecific antibody to maintain its specificity for HER2-positive cancer cells. The other arm of the bispecific antibody can be engineered to bind to an immune cell receptor or a second tumour antigen. Week 1; Practical: Recombinant antibody engineering 1. Generate DNA of antibody genes; CDR 1,2,3 of both HC and LC - Strategy 1: o Isolate RNA from hybridoma cells o RT-PCR of immunoglobulin - Strategy 2: o Search publications for sequences o Synthesis DNA sequences de novo (commercial service) → method of practical - Strategy 3: o Phage display technology, isolates antibody genes in E.coli 2. Clone HeavyC and LightC DNA sequences into expression vectors Needs “in frame” cloning between LP, VH and CH1 and between LP,VL and CL 3. Transfect HC and LC expression vectors in eukaryotic (often mammalian) cells + Purify antibodies (e.g. with protein A or G) Example Chinese hamster ovary cells (CHO), HEK293 cells or tabacco cells (plants) Steps practical (recombinant antibody generation) Find antibody sequences Decide whether to use bacterial, yeast, mammalian, or other expression systems Design DNA sequences encoding the antibody variable regions (VH and VL) and constant regions (CH and CL) → Look up patent to find protein sequences → use reverse translation tool to get the DNA sequences Find expression vector sequence Search for vector plasmid for heavy chain and one for light chain via the program invivogen Identify relevant restrictions sites Look at vector description E.g. Locate the Eco RI, Eco RV, Xho I, and Nhe I restriction sites within the sequences. Design insert sequences for DNA synthesis Insert whole DNA sequence of step 1 with the sequence of the restriction enzymes at correct site (5’ or 3’). Add sometimes 1 or 2 nucleotides after enzyme to make sure reading frame is maintained with signal sequence. Add also the whole vector sequences to get your recombinant plasmid! Otherwise you have a linear plasmid and not a circular. Perform in silico restriction and ligation Week 1; Journal club: Antibody C Region Influences TGN1412-like Functional Activity In Vitro Aim study monoclonal antibody TGN1412, developed to treat leukemia and autoimmune disorders by expanding regulatory T cells. the phase I trial of TGN1412 resulted in severe adverse reactions in human volunteers; all six volunteers receiving the Ab suffered systemic inflammatory response syndrome and life-threatening multiorgan failure. immuno-modulatory activity of TGN1412 is lacking → therefore this study Investigations of study Study compares binding to the TGN1412 in humans compared to macaques (monkeys). investigates the influence of the Fc domain and C region structure on the in vitro immunomodulatory activity of TGN1412. They generated F(ab) 2 and Fab fragments from TGN1412 by enzyme digestion and created recombinant subclass variants (IgG1, IgG2 and IgG4) and two mutant IgG4 mAbs containing the L chains and V region sequence of TGN1412. Investigate the ability of the mAbs and fragments to stimulate cytokine release from PBMCs and induce proliferation of PBMCs. Results of study Binding TGN1412 TGN1412 showed strong binding to the high-affinity FcγRIa receptor but minimal or no binding to other Fc receptors in both species, indicating specificity in its interactions. TGN1412 showed weaker binding compared to IgG1 but stronger binding than IgG4 to human Fc gamma receptor Ia (FcgRIa) → difference in binding affinity could be due to variations in dimer content and glycosylation. CD28 binding properties → to exclude the possibility that binding was significantly altered by the manipulations. They compared Aqueous phase and biotinylated F(ab’) 2 fragments bound slightly less biotinylated rCD28/Fc than TGN1412. Monovalent Fab fragment reduced ability to bind biotinylated rCD28/Fc when immobilized → CD28 binding: TGN1412 > F(ab’) 2 > Fab Subclasses: IgG4 displayed equivalent CD28 binding properties to TGN1412 The two IgG4 mutant showed identical binding properties to the IgG4 subtype IgG1 also showed comparable binding IgG2 showed brighter staining of lymphocytes than TGN1412 and igG4 using Flow cytometry. → Ab Fc did not significantly affect binding to cell surface CD28 with the exception of NIB(28SA)-G2 → TGN1412= IgG4= IgG4 mutants > IgG2 > IgG1 Binding to recombinant FcgRIa, FcgRIIa and FcgRIIIa FcgRIa: igG1 > TGN1412 > IgG4 + IgG4 mutants > IgG2 FcgRIIa: igG2 > igG1 > TGN1412> IgG4 + IgG4 mutants > IgG2 FcgRIIIa: igG1 > all the rest is at same level Functional activity of TGN1412 and other abs Immobilized TGN1412 induced significant release of inflammatory cytokines (TNF-a, IL-2, and IFN-g) and cell proliferation, with optimal responses observed at a specific mAb concentration. The F(ab)2 fragments showed a different response pattern, with lower cytokine release at lower doses but increasing responses at higher doses igG2 showed higher maximum responses compared to igG4 and IgG1 igG 4 higher maximum responses than igG4 mutants