Investigating Campylobacter jejuni Transcriptional Response to Host Intestinal Extracts - PDF
Document Details
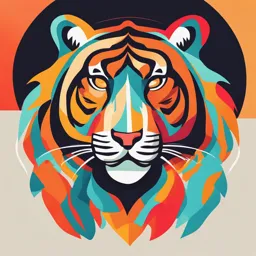
Uploaded by StylishAntigorite8669
University of British Columbia
Martha M. Liu, Christine J. Boinett, Anson C. K. Chan, Julian Parkhill, Michael E. P. Murphy, Erin C. Gaynor
Tags
Related
- FC11-GRATTARD-Les-infections-digestives-10.10-1.pdf Infections digestives PDF
- Campylobacter, Helicobacter & Vibrio Genera PDF
- Clase 15, Vibrio, Campylobacter y Helicobacter PDF
- Vibrio, Campylobacter y Helicobacter PDF
- Acinetobacter, Pseudomonas, Yersinia, Campylobacter PDF
- Vibrio, Campylobacter, and Helicobacter PDF
Summary
This research article investigates the transcriptional response of Campylobacter jejuni to host intestinal extracts. The study compares the responses of the bacteria to human fecal and chicken cecal extracts. Researchers found that C. jejuni displayed differing levels of gene expression related to various processes such as iron uptake, metabolism, chemotaxis, and energy production when exposed to the different extracts. The article specifically investigates a new iron uptake system implicated in C. jejuni survival and infection in the human intestinal environment.
Full Transcript
RESEARCH ARTICLE crossm Investigating the Campylobacter jejuni Transcriptional...
RESEARCH ARTICLE crossm Investigating the Campylobacter jejuni Transcriptional Downloaded from http://mbio.asm.org/ on October 28, 2020 at UNIVERSITY OF READING Response to Host Intestinal Extracts Reveals the Involvement of a Widely Conserved Iron Uptake System Martha M. Liu,a Christine J. Boinett,b Anson C. K. Chan,a Julian Parkhill,b Michael E. P. Murphy,a Erin C. Gaynora a Department of Microbiology and Immunology, University of British Columbia, Vancouver, BC, Canada b Wellcome Sanger Institute, Wellcome Genome Campus, Hinxton, Cambridgeshire, United Kingdom ABSTRACT Campylobacter jejuni is a pathogenic bacterium that causes gastroenteri- tis in humans yet is a widespread commensal in wild and domestic animals, particu- larly poultry. Using RNA sequencing, we assessed C. jejuni transcriptional responses to medium supplemented with human fecal versus chicken cecal extracts and in extract-supplemented medium versus medium alone. C. jejuni exposed to extracts had altered expression of 40 genes related to iron uptake, metabolism, chemotaxis, energy production, and osmotic stress response. In human fecal versus chicken cecal extracts, C. jejuni displayed higher expression of genes involved in respiration (fdhTU) and in known or putative iron uptake systems (cfbpA, ceuB, chuC, and CJJ81176_1649 –1655 [here designated 1649 –1655]). The 1649 –1655 genes and downstream overlapping gene 1656 were investigated further. Uncharacterized ho- mologues of this system were identified in 33 diverse bacterial species representing 6 different phyla, 21 of which are associated with human disease. The 1649 and 1650 (p19) genes encode an iron transporter and a periplasmic iron binding protein, respectively; however, the role of the downstream 1651–1656 genes was unknown. A ⌬1651–1656 deletion strain had an iron-sensitive phenotype, consistent with a pre- viously characterized ⌬p19 mutant, and showed reduced growth in acidic medium, increased sensitivity to streptomycin, and higher resistance to H2O2 stress. In iron- restricted medium, the 1651–1656 and p19 genes were required for optimal growth when using human fecal extracts as an iron source. Collectively, this implicates a function for the 1649 –1656 gene cluster in C. jejuni iron scavenging and stress sur- vival in the human intestinal environment. IMPORTANCE Direct comparative studies of C. jejuni infection of a zoonotic com- mensal host and a disease-susceptible host are crucial to understanding the causes of infection outcome in humans. These studies are hampered by the lack of a Received 21 June 2018 Accepted 27 June disease-susceptible animal model reliably displaying a similar pathology to human 2018 Published 7 August 2018 campylobacteriosis. In this work, we compared the phenotypic and transcriptional Citation Liu MM, Boinett CJ, Chan ACK, Parkhill J, Murphy MEP, Gaynor EC. 2018. Investigating responses of C. jejuni to intestinal compositions of humans (disease-susceptible host) the Campylobacter jejuni transcriptional and chickens (zoonotic host) by using human fecal and chicken cecal extracts. The response to host intestinal extracts reveals the involvement of a widely conserved iron uptake mammalian gut is a complex and dynamic system containing thousands of metabo- system. mBio 9:e01347-18. https://doi.org/10 lites that contribute to host health and modulate pathogen activity. We identified.1128/mBio.01347-18. C. jejuni genes more highly expressed during exposure to human fecal extracts in Editor Martin J. Blaser, New York University comparison to chicken cecal extracts and differentially expressed in extracts com- Copyright © 2018 Liu et al. This is an open- access article distributed under the terms of pared with medium alone, and targeted one specific iron uptake system for further the Creative Commons Attribution 4.0 molecular, genetic, and phenotypic study. International license. Address correspondence to Michael E. P. Murphy, [email protected], or Erin C. KEYWORDS Campylobacter jejuni, RNA-seq, acid resistance, chicken cecal extract, Gaynor, [email protected]. human fecal extract, iron transport, p19, streptomycin resistance, transcriptome ® July/August 2018 Volume 9 Issue 4 e01347-18 mbio.asm.org 1 ® Liu et al. C ampylobacter jejuni is a leading bacterial cause of human foodborne illness world- wide in both developed and developing nations (1, 2). This zoonotic bacterium asymptomatically colonizes the digestive tracts of wild and domestic animals, with population colonization rates measured as high as 86% (3–6). The most common reservoir of C. jejuni transmission to humans is poultry, particularly chickens. C. jejuni can colonize chicken ceca, which are two blind pouches at the junction of the small and large intestines, to concentrations as high as 2.5 ⫻ 109 CFU/g cecal material without Downloaded from http://mbio.asm.org/ on October 28, 2020 at UNIVERSITY OF READING signs of pathology (7, 8). In humans, C. jejuni infection can result in sporadic cases or localized outbreaks of disease due to ingestion of contaminated meat, water, or unpasteurized milk and improper handling of animals (1, 9, 10). Accidental ingestion of as little as a few hundred bacterial cells can cause severe but typically self-limiting acute gastrointestinal illness ranging from mild to bloody diarrhea, nausea, and vomiting as early as 17 h postingestion and lasting from days to weeks (11–13). During human infection, C. jejuni colonizes the large intestine and can replicate to concentrations as high as 3.0 ⫻ 108 CFU/g fecal material (12). C. jejuni infection can also cause long-term chronic inflammatory bowel diseases (e.g., Crohn’s disease, ulcerative colitis, and colorectal cancer) and autoimmune inflammatory demyelination diseases (e.g., Guillain- Barré and Miller-Fisher syndromes) in a small percentage of infected individuals (14, 15). Despite extensive study, it remains enigmatic why C. jejuni causes such acute human disease but harmlessly colonizes its zoonotic hosts, such as chickens. The intestinal metabolome is a complex and dynamic system consisting of thou- sands of metabolites that both contribute and respond to intestinal health (16–18). The known metabolites, including short-chain fatty acids (SCFAs), organic acids, bile salts, lipids, amino acids, vitamins, and trace minerals (17), only account for a small fraction of the metabolome, with thousands of as yet unidentified metabolites that may play a role in intestinal health (19). Salmonella enterica serovar Typhimurium was recently shown to respond to human fecal metabolites by increasing the expression of genes related to metabolism, motility, and chemotaxis, reducing the expression of genes involved in host cell invasion, and reducing pathogen invasion of HeLa cells (19). The metabolomes of the chicken ceca and the human large intestine are expected to be dissimilar due to factors such as differences in diet, digestive system structure and function, host defense peptides, and the resident microbiome (20–22). These factors also contribute to the availability of trace metals and micronutrients, such as iron, that are essential for bacterial cell growth and host colonization (23–25). We thus hypoth- esized that C. jejuni exposed to human fecal extracts would respond by altering expression of genes specifically required for human infection. In this study, we compared phenotypic and gene expression differences in C. jejuni cells exposed to medium containing sterile intestinal extracts of the disease-susceptible host (humans) versus the commensal host (chickens). Among the differentially ex- pressed genes, we selected one putative and as yet uncharacterized iron uptake gene cluster (CJJ81176_1649 –1656) for further study, as it was significantly more highly expressed during growth in medium with extracts compared to medium alone, and in particular in medium containing human fecal compared to chicken cecal extracts. This gene cluster is here referred to as “1649 –1656,” and all genes discussed throughout the article will use the CJJ81176 gene locus numbers and protein designations. Collectively, this work shows that C. jejuni responds differentially to metabolites present in the intestinal lumen of commensal versus susceptible hosts, provides a broad database repository of the C. jejuni transcriptional gene responses to these conditions, and shows that the 1649 –1656 iron uptake system participates in numerous key phenotypes and thus may be integral to host infection, especially in humans. RESULTS C. jejuni cultured in medium containing chicken cecal or human fecal extracts compared to medium alone exhibited comparable levels of logarithmic growth, enhanced late-stage survival, and decreased biofilm formation. Sterile pooled chicken cecal extract from 35 chickens (chicken pool [CP]) and three unique pools of July/August 2018 Volume 9 Issue 4 e01347-18 mbio.asm.org 2 ® C. jejuni, Host Gut Extracts, and Iron Downloaded from http://mbio.asm.org/ on October 28, 2020 at UNIVERSITY OF READING FIG 1 C. jejuni growth and biofilm formation in the presence of prepared extracts. (A) The four sterile pooled extracts (chicken pool [CP] and human pools 1, 2, and 3 [HP1, HP2, and HP3]) were prepared from 35 individual chicken cecal extracts and 9 human fecal extracts (H1C, H2, etc., human fecal extract numerical identifier; M/F, male/female). (B) C. jejuni growth in MH, MH plus 30% CP, and MH plus 30% HP1, HP2, and HP3 as measured by dilution plating. (C) C. jejuni biofilm formation over time with and without 10% extract as measured by the crystal violet assay (top) compared to the viable planktonic cell count (bottom). Error bars represent the standard deviation from 3 replicates. Statistical analysis for growth and biofilm formation was performed using the Student’s t test with Welch’s correction and compares MH plus extract conditions (CP, pink; HP1, light blue; HP2, medium blue; HP3, dark blue) to the MH control (black) for each time point. *, P ⬍ 0.05; **, P ⬍ 0.005; ***, P ⬍ 0.0005; ****, P ⬍ 0.0001. human fecal extracts from 9 volunteers (human pools 1, 2, and 3 [HP1, HP2, and HP3]) were prepared by dilution of cecal/fecal material in sterile H2O and sterilization by filtration (Fig. 1A). Wild-type C. jejuni strain 81-176 was grown in Mueller-Hinton medium (MH) alone or MH supplemented with 30% CP, HP1, HP2, or HP3 to investigate the effects of extract exposure on in vitro growth and biofilm formation (Fig. 1B and C). The 30% supplement condition was selected for these analyses based on limited extract volume and C. jejuni sensitivity to high osmotic stress (26). C. jejuni exponential growth rates were comparable under each condition for the first 12 h after inoculation, with doubling times ranging between 1.9 and 2.2 h (Fig. 1B). At 24 h, C. jejuni cells grown in medium alone showed reduced viability, as expected under this growth condition, while C. jejuni cells incubated in medium containing either chicken cecal or human fecal extracts maintained significantly higher viability (Fig. 1B). Growth in extracts did not impact C. jejuni cell morphology (data not shown). Biofilm formation was assessed for C. jejuni exposed to medium alone, and to medium containing 10% chicken cecal or human fecal extracts (Fig. 1C). A concentration of 10% extract was selected to conserve extract, as preliminary testing showed no difference between levels of C. jejuni biofilm formation in medium containing 10% or 30% extracts (data not shown). After 12 h, biofilm formation in medium containing extracts was comparable to or slightly higher than that in medium alone. However, at 24 and 36 h, C. jejuni cells grown in medium containing extracts exhibited lower biofilm abundance than cells grown in medium alone. One exception was C. jejuni cells grown in medium containing 10% CP at 36 h, which did not show a significant difference. The reduced biofilm formation in medium containing extracts was not caused by poor growth, since planktonic cell concentra- July/August 2018 Volume 9 Issue 4 e01347-18 mbio.asm.org 3 ® Liu et al. TABLE 1 C. jejuni genes showing higher expression (⬎2-fold) in medium containing extract than in medium alone 20 min 5h Fold Fold Locus tag Gene name Gene product function change P value change P value Metabolism, nutrient uptake, and energy production CJJ81176_0122 aspA Aspartate ammonia-lyase 3.2 6.13E⫺08 2.5 4.61E⫺05 Downloaded from http://mbio.asm.org/ on October 28, 2020 at UNIVERSITY OF READING CJJ81176_0123 dcuA Anaerobic C4-dicarboxylate membrane 2.9 2.79E⫺09 2.5 1.31E⫺06 transporter DcuA CJJ81176_0438 CJJ81176_0438 Putative oxidoreductase subunit 4.5 1.97E⫺54 11.8 8.63E⫺149 CJJ81176_0439 CJJ81176_0439 Oxidoreductase, putative 4.4 4.54E⫺28 12.1 2.71E⫺80 CJJ81176_0697 dcuB Anaerobic C4-dicarboxylate membrane 2.1 3.52E⫺08 3.1 4.10E⫺19 transporter DcuB CJJ81176_0884 CJJ81176_0884 Cytochrome c family protein, degenerate 2.1 2.47E⫺08 2.9 5.25E⫺19 CJJ81176_0885 CJJ81176_0885 Cytochrome c 2.4 5.34E⫺10 4.1 3.16E⫺27 CJJ81176_1389 CJJ81176_1389 DNA-binding protein 2.1 2.81E⫺05 CJJ81176_1390 CJJ81176_1390 Reactive intermediate/imine deaminase 2.4 3.87E⫺02 CJJ81176_1391 CJJ81176_1391 C4-dicarboxylate ABC transporter 2.5 1.31E⫺02 CJJ81176_1392 metC Cystathionine -lyase 2.5 6.81E⫺03 CJJ81176_1393 purB-2 Adenylosuccinate lyase 2.2 2.57E⫺02 CJJ81176_1570 CJJ81176_1570 Anaerobic dimethyl sulfoxide reductase 2.4 8.60E⫺03 chain A Iron uptake CJJ81176_1649 CJJ81176_1649 Iron permease, FTR1 family 3.2 1.19E⫺04 CJJ81176_1650 p19 Periplasmic iron binding protein 3.8 1.01E⫺05 CJJ81176_1651 CJJ81176_1651 Membrane protein, putative 2.9 9.05E⫺04 CJJ81176_1652 CJJ81176_1652 ABC transporter, permease protein 3.5 6.02E⫺05 CJJ81176_1653 CJJ81176_1653 ABC transporter, permease protein 3.9 5.62E⫺06 CJJ81176_1654 CJJ81176_1654 ABC transporter, ATP-binding protein 4.3 1.14E⫺06 CJJ81176_1655 CJJ81176_1655 Thioredoxin, homologue 4.2 4.84E⫺06 Hypothetical proteins CJJ81176_0204 CJJ81176_0204 Hypothetical protein 2.9 1.85E⫺12 4.4 3.99E⫺26 CJJ81176_0440 CJJ81176_0440 Conserved hypothetical protein 2.4 1.69E⫺11 2.1 1.12E⫺08 CJJ81176_1005 CJJ81176_1005 Membrane protein, putative 2.1 1.11E⫺03 tions were comparable or higher under these conditions, similar to observations in shaking broth noted above. C. jejuni exposed to host intestinal extracts responded by altering the expres- sion of metabolism and iron uptake-related genes. We used RNA sequencing to assess the transcriptional response of C. jejuni upon exposure to extracts. C. jejuni cells were incubated in medium alone, medium plus 30% CP, and medium plus 30% HP1, HP2, or HP3. RNA was collected after 20 min to assess transient differences in gene expression and after 5 h to assess adaptive homeostatic differences. Sample data from the 20 different conditions were grouped as outlined in Table S1 in the supplemental material, and two different evaluation conditions were analyzed in detail: (i) exposure to medium containing extract versus medium alone (referred to as extract versus medium— discussed in this section) and (ii) exposure to human fecal extracts versus chicken cecal extract (referred to as human versus chicken— discussed in the next section). C. jejuni cells exposed to medium containing either human or chicken extracts versus medium alone revealed 23 genes with ⬎2-fold higher expression (Table 1) and 17 genes with ⬎2-fold lower expression (Table 2). Of the genes showing higher expression after exposure to extracts, 13 were related to metabolism, nutrient uptake, and energy production (aspA, dcuA, dcuB, 0438, 0439, 0884, 0885, 1389 –1391, metC, purB-2, and 1570) (27–29), and 3 encoded hypothetical proteins with no annotated function (0204, 0440, and 1005). Also demonstrating higher expression was a 7-gene cluster (1649 –1655) that includes a homologue of an iron transporter in Escherichia coli (1649) (30), and p19 (1650), encoding a periplasmic iron binding protein involved in iron transport (25, 31) (Table 1). Genes 0438 and 0439 showed the largest increase in July/August 2018 Volume 9 Issue 4 e01347-18 mbio.asm.org 4 ® C. jejuni, Host Gut Extracts, and Iron TABLE 2 C. jejuni genes showing lower expression (⬎2-fold) in medium containing extract versus medium alone 20 min 5h Fold Fold Locus tag Gene name Gene product function change P value change P value Adhesin CJJ81176_0315 peb3 Major antigenic peptide PEB3 ⫺3.0 2.93E⫺06 ⫺6.2 2.33E⫺17 Downloaded from http://mbio.asm.org/ on October 28, 2020 at UNIVERSITY OF READING Metabolism, nutrient uptake, and energy production CJJ81176_0033 gltB Glutamate synthase, large subunit ⫺2.1 5.06E⫺04 CJJ81176_0035 gltD Glutamate synthase, small subunit ⫺2.1 8.27E⫺31 CJJ81176_0580 CJJ81176_0580 C4-dicarboxylate ABC transporter ⫺3.4 5.77E⫺21 CJJ81176_0581 CJJ81176_0581 Amidohydrolase ⫺2.0 3.04E⫺07 CJJ81176_0685 CJJ81176_0685 Di/tripeptide transporter ⫺2.9 1.81E⫺26 CJJ81176_0912 CJJ81176_0912 Amino acid carrier protein ⫺2.5 1.88E⫺08 CJJ81176_0941 CJJ81176_0941 Sodium:alanine symporter ⫺2.5 7.05E⫺07 CJJ81176_0942 CJJ81176_0942 Sodium:alanine symporter ⫺2.6 9.79E⫺07 CJJ81176_1386 CJJ81176_1386 Fumarate reductase ⫺2.6 4.37E⫺02 Chemotaxis CJJ81176_0109 CJJ81176_0109 Methyl-accepting chemotaxis protein ⫺2.4 1.13E⫺03 Oxidative response CJJ81176_0266 herA Hemerythrin ⫺2.1 2.08E⫺02 CJJ81176_1656 CJJ81176_1656 Thioredoxin family protein ⫺2.0 2.88E⫺02 Hypothetical proteins CJJ81176_1006 CJJ81176_1006 Hypothetical protein ⫺2.3 1.43E⫺03 CJJ81176_1184 CJJ81176_1184 Hypothetical protein ⫺2.5 3.58E⫺04 CJJ81176_1185 CJJ81176_1185 Hypothetical protein ⫺2.6 9.42E⫺04 CJJ81176_1657 CJJ81176_1657 Hypothetical protein ⫺2.6 5.54E⫺31 ⫺2.7 1.72E⫺32 expression both at 20 min (4.5- and 4.4-fold, respectively) and 5 h (11.8- and 12.1-fold, respectively). These genes (originally annotated by NCTC11168 locus tags cj0414 and cj0415) are required for gluconate dehydrogenase activity, allowing C. jejuni to use gluconate as an electron donor (32). Of the 17 genes showing reduced expression after incubation in medium supplemented with extracts in comparison to medium alone, one was a cell surface adhesin (peb3), 9 were involved in metabolism, nutrient uptake, and energy production (gltB, gltD, 0580, 0581, 0685, 0912, 0941, 0942, and 1386), 2 were involved in oxidative stress (herA and 1656), and 4 encoded hypothetical proteins with no annotated function (1006, 1184, 1185, and 1657) (Table 2). The gene exhibiting the greatest decrease during incubation in extracts was peb3 at both 20 min (3.0-fold) and 5 h (6.2-fold). It encodes a cell surface glycoprotein adhesin that also imports phos- phorylated compounds (33). PEB3 is a major antigenic protein during human infection, so rapid downregulation of this gene may be advantageous to host colonization (34, 35). A list of differentially expressed genes in C. jejuni cultured in medium with either human or chicken extracts in comparison to medium alone at each sampling time is in Table S2 in the supplemental material, and a Venn diagram of these genes is presented in Fig. S1 in the supplemental material. C. jejuni exposed to human fecal extracts versus chicken cecal extract had higher expression of iron uptake and formate dehydrogenase genes. As noted above, we also compared C. jejuni gene expression during growth in medium supple- mented with human fecal extracts to expression during growth in medium supple- mented with chicken cecal extract. This identified 2 genes with ⬎2-fold higher expres- sion after 20 min and 12 genes with higher expression after 5 h (Table 3). No genes showing reduced expression were identified. The 2 genes with higher expression in human extracts after 20 min of exposure (fdhT, 2.4-fold; fdhU, 2.9-fold) increased further at 5 h (6.4- and 3.3-fold higher, respectively). FdhTU is involved in formate dehydro- genase activity and contributes to the invasion and intracellular survival of C. jejuni in intestinal epithelial cells (36, 37). The remaining 10 genes with 2.5- to 3.4-fold higher July/August 2018 Volume 9 Issue 4 e01347-18 mbio.asm.org 5 ® Liu et al. TABLE 3 C. jejuni genes showing higher expression in medium containing human fecal extract in comparison to medium containing chicken cecal extract 20 min 5h Fold Fold Locus tag Gene name Gene product function change P value change P value Respiration CJJ81176_1492 fdhT Membrane protein, putative 2.9 8.27E⫺03 6.4 1.29E⫺10 Downloaded from http://mbio.asm.org/ on October 28, 2020 at UNIVERSITY OF READING CJJ81176_1493 fdhU Conserved hypothetical protein 2.4 3.00E⫺02 3.3 8.96E⫺05 Iron uptake CJJ81176_0211 cfbpA Iron ABC transporter, periplasmic iron-binding protein 2.8 1.56E⫺02 CJJ81176_1351 ceuB Enterochelin ABC transporter, permease protein 2.9 1.58E⫺02 CJJ81176_1603 chuC Hemin ABC transporter, ATP-binding protein, putative 3.4 2.63E⫺02 CJJ81176_1649 CJJ81176_1649 Iron permease, FTR1 family 2.7 3.44E⫺03 CJJ81176_1650 p19 Periplasmic iron binding protein 2.9 2.05E⫺03 CJJ81176_1651 CJJ81176_1651 Membrane protein, putative 2.6 7.86E⫺03 CJJ81176_1652 CJJ81176_1652 ABC transporter, permease protein 2.7 7.86E⫺03 CJJ81176_1653 CJJ81176_1653 ABC transporter, permease protein 2.7 7.86E⫺03 CJJ81176_1654 CJJ81176_1654 ABC transporter, ATP-binding protein 2.5 1.58E⫺02 CJJ81176_1655 CJJ81176_1655 Thioredoxin, homologue 2.5 3.59E⫺02 expression after 5 h are known or hypothesized to be involved in iron uptake and/or utilization (Table 3). The cfbpA, ceuB, and chuC genes encode parts of three different C. jejuni iron uptake systems: cfbpA encodes the periplasmic iron binding protein for the ferri-transferrin uptake system (38), ceuB encodes the periplasmic permease for the ferri-enterochelin uptake system (39), and chuC encodes part of the ABC transporter system for the heme uptake system (40, 41). None of the other components in these iron uptake systems showed higher expression in human fecal extracts versus chicken cecal extract (41). The remaining 7 genes were 1649 –1655, which were also more highly expressed in C. jejuni cells exposed to extracts in comparison to those exposed to medium alone and are described in more detail in the following sections. Human fecal extracts contain higher total iron content than chicken cecal extract. Since the majority of the genes more highly expressed during growth in human fecal extracts were related to iron uptake, we hypothesized that cells grown in human fecal extracts were more iron starved than cells growing in chicken cecal extract. Quantification of the total iron present in extracts in all its forms was carried out using inductively coupled plasma mass spectrometry (ICP-MS). Unexpectedly, human extracts were found to contain approximately four times more iron than the chicken cecal extract (Fig. 2), suggesting the iron present is tightly chelated and requires specialized uptake systems. Homologues of 1649 –1655 are widely conserved in the bacterial kingdom. The increased expression of the 1649 –1655 genes suggested that they are important in the C. jejuni response to extracts, especially human fecal extracts. The Escherichia coli homologue (FetM) of 1649 is a ferrous iron permease (30), and the periplasmic protein 1650 (P19) has been characterized as an iron transporter in C. jejuni (25), E. coli (30), FIG 2 Iron concentration of pooled chicken and human extracts. The iron concentration of each pooled extract was measured using ICP-MS. Error bars represent the standard deviation from 2 duplicate tests. The significance was calculated by ANOVA. ***, P ⬍ 0.005. July/August 2018 Volume 9 Issue 4 e01347-18 mbio.asm.org 6 ® C. jejuni, Host Gut Extracts, and Iron Downloaded from http://mbio.asm.org/ on October 28, 2020 at UNIVERSITY OF READING FIG 3 The 1649 –1656 operon in C. jejuni and other bacterial species. (A) Proposed operon structure in C. jejuni wild-type strain 81-176, including flanking genes 1648 and 1657. TSS, transcription start site. (B) Homologues found within representative bacterial species identified with blastp are shown to scale. The colored bars correspond to the genes described in the legend. Red and pink circles designate that the organism is associated with human disease or colonization, respectively. Organisms listed in alphabetical order: Actinobacillus succinogenes 130Z, Aggregatibacter actinomycetemcomitans NUM4039, Bifidobacterium kashiwanohense PV20-2, Brenneria goodwinii, Campylobacter coli RM4661, Campylobacter fetus 82-40, Campylobacter jejuni 81-176, Campylobacter lari RM2100, Citrobacter koseri ATCC BAA-895, Escherichia coli VR50(pVR50B), Filifactor alocis ATCC 35896, Flexistipes sinusarabici DSM 4947, Frischella perrara PEB0191, Haemophilus parainfluenzae T3T1, Jonquetella anthropi DSM 22815, Klebsiella pneumoniae B2(pB2-A/C), Leminorella grimontii ATCC 33999 (⫽ DSM 5078), Mobiluncus curtisii ATCC 43063, Paracoccus halophilus, Parascardovia denticolens DSM 10105, Parvimonas micra KCOM 1535, Pasteurella multocida Pm70, Pectobacterium carotovorum PC1, Pelosinus sp. strain UFO1, Rhodospirillum rubrum ATCC 11170, Salmonella enterica serovar Typhimurium T000240, Selenomonas sputigena ATCC 35185, Shigella dysenteriae 80-547(p80-547), Spirochaeta thermophila DSM 6578, Sulfurospirillum multivorans DSM 12446, Treponema sp. strain OMZ 838, Wolinella succinogenes DSM 1740, and Yersinia pestis CO92. Bordetella (42), and Yersinia pestis (43). However, no prevalence or functional studies have been reported on the downstream genes (1651–1656) or homologues. As deter- mined by conserved domain analyses and genome annotation, 1651–1655 were pre- dicted to consecutively encode an inner membrane protein (1651), two inner mem- brane permeases (1652 and 1653), a cytoplasmic ATPase (1654), and a periplasmic thioredoxin (1655) (Fig. 3A). Also, one downstream gene (1656) overlaps with 1655 by 35 bp, encodes a second putative periplasmic thioredoxin (1656), and is assumed to be part of the same gene cluster (Fig. 3A). This set of 8 genes (1649 –1656) is carried directly downstream of a Fur box and has one putative primary transcription start site located 54 bases upstream of the 1649 start codon, suggesting that these genes are transcribed as part of an operon (Fig. 3A) (44). The gene upstream of 1649 (1648, encoding a hypothetical protein) ends 350 bp before the start of 1649, and the gene downstream of 1656 (1657, also encoding a hypothetical protein) is carried on the opposite strand. Each of these flanking genes has its own primary transcription start sites and is thus unlikely part of the proposed 1649 –1656 operon (44). Protein BLAST analyses showed that the 1649 –1656 system is widely conserved in the bacterial kingdom. Components of the system are found in 22 of 28 fully sequenced Campylobacter species. Of the species lacking components of this system, C. avium, C. helveticus, C. hepaticus, and C. ornithocola have not been associated with human July/August 2018 Volume 9 Issue 4 e01347-18 mbio.asm.org 7 ® Liu et al. illness, and C. lanienae and C. sputorum are infrequently found by PCR in stools of both healthy and diarrhetic humans (45, 46). We also identified representative homologues of 1649 –1656 in 33 phylogenetically diverse bacterial species with available complete genome sequences (Fig. 3B; see Table S3 in the supplemental material). Homologous gene products were most commonly found in members of the Proteobacteria, but were also present in Actinobacteria, Firmicutes, Deferribacteres, Spirochaetes, and Synergistes. Genes making up the operon were chromosomally carried in 30 of the 33 bacterial Downloaded from http://mbio.asm.org/ on October 28, 2020 at UNIVERSITY OF READING species and on plasmids in Escherichia, Klebsiella, and Shigella. Homologues of 1649 – 1656 were found in both Gram-positive and Gram-negative bacteria colonizing and infecting humans (22 out of 33 species identified), cows (Actinobacillus succinogenes and Wolinella succinogenes [47, 48]), honey bees (Frischella perrara ), and plants (Brenneria goodwinii and Pectobacterium carotovorum [50, 51]), as well as environmental isolates from water (Flexistipes sinusarabici, Paracoccus halophilus, and Rhodospirillum rubrum [52–54]), soil (Pelosinus sp. and Sulfurospirillum halorespirans [55, 56]), and thermal sites (Spirochaeta thermophila ). With respect to human colonizers, most bacterial species identified were associated with diseases such as gastroenteritis (e.g., Campylobacter jejuni), periodontal disease (e.g., Filifactor alocis ), urinary tract infection (e.g., Leminorella grimontii ), vaginosis (e.g., Mobiluncus curtisii ), and spondylodiscitis (e.g., Parvimonas micra ). The organization of the operon and predicted gene products in each of the 33 bacterial species were examined. All 33 species carried homologues of the first six genes (1649 –1654) with the same gene order, similar gene lengths, and comparable spacing between genes (Fig. 3B). While most members of the Proteobacteria carried a predicted thioredoxin gene as the seventh gene in the cluster, members of the Actinobacteria, Firmicutes, Spirochaetes, and Synergistetes encoded a predicted flavin mononucleotide (FMN) binding protein. Although the thioredoxins may not be directly involved in iron uptake, both thioredoxins and FMN binding proteins can serve as a source of periplasmic or extracytoplasmic reduction potential to reduce ferric iron to the ferrous form for transport into the cell. In Proteobacteria without a thioredoxin encoded by the eighth gene, a gene coding for either a cytochrome or a hypothetical protein was present. The high genetic conservation of this system in multiple bacterial phyla suggests that, minimally, the first 7 gene products form one functional system, which is further investigated in the following sections. The 1651–1656 genes are involved in iron acquisition. To determine if the 1651–1656 genes were involved in iron acquisition similar to 1649 and p19, deletion (⌬1651–1656) and complemented (1651–1656C) C. jejuni strains were constructed (Fig. 4). The p19 (1650) deletion mutant (⌬p19) and complemented (p19C) strains were used as controls since P19 is well conserved and is known to be involved in iron transport (25). The ⌬p19 and ⌬1651–1656 mutants each exhibited lower growth rates than the wild-type 81-176 strain or the corresponding complements during growth in MH medium, but reached comparable final viable cell concentrations at 32 h (Fig. 4D). Iron depletion by addition of 20 M desferroxamine (DFO), an iron chelator, resulted in a similar initial growth rate for the ⌬1651–1656 mutant, a modestly reduced initial growth rate for the ⌬p19 mutant, and significantly reduced viability for both mutants after 12 h compared to the wild-type and complemented strains (Fig. 4E). Growth rates in MH for each mutant could be restored to wild-type and complement levels by supplementation with 100 M iron(III) citrate (Fig. 4F). The 1649 –1656 iron uptake system is involved in the C. jejuni response to acid, streptomycin, and H2O2-mediated oxidative stress. C. jejuni wild-type strain 81-176, the ⌬p19 and ⌬1651–1656 mutant strains, and the respective complements were exposed to acid (pH 5), antibiotic, and oxidative stress (1 mM H2O2) conditions to determine whether this iron uptake system is important for C. jejuni stress survival (Fig. 5). To test acid tolerance, cells were inoculated into MH at neutral pH or MH at pH 5 with and without supplementation with 100 M iron(III) citrate and grown for 24 h. The ⌬p19 and ⌬1651–1656 mutants exhibited a 2- to 3-log reduction in growth at pH 5 in July/August 2018 Volume 9 Issue 4 e01347-18 mbio.asm.org 8 ® C. jejuni, Host Gut Extracts, and Iron Downloaded from http://mbio.asm.org/ on October 28, 2020 at UNIVERSITY OF READING FIG 4 Construction of the ⌬1651–1656 mutant and complemented strains (1651–1656C) and their growth in iron-limiting and iron-supplemented media compared to the ⌬p19 and p19C strains. (A) The wild-type 1649 –1656 gene locus, including the upstream and downstream genes as well as the 16S rRNA region used for complementation. TSS, transcription start site; blue box, Fur binding sequence (Fur box). (B) The ⌬1651–1656 mutant was constructed by replacing 1651–1656 with a kanamycin resistance cassette (Kmr). The region deleted is indicated. (C) Using the integrative vector pRRC (85), the 1651–1656 gene cluster was inserted into a noncoding region between the 16S rRNA and tRNA-Ala to generate the 1651–1656C complement. The 1651–1656 genes in the complemented strain are under the control of a chloramphenicol promoter (Pcm) upstream of the chloramphenicol resistance marker (Cmr) used for selection. Growth over time of the C. jejuni wild-type (black solid line), ⌬1651–1656 (red dotted line), 1651–1656C (red solid line), ⌬p19 (blue dotted line), and p19C (blue solid line) strains in MH (D), iron-depleted MH containing 20 M DFO (E), and iron-supplemented MH containing 100 M iron(III) citrate (F) was measured by dilution plating. Error bars represent the standard deviations from 3 replicates from a total of 3 experiments. Statistical comparison of the ⌬1651–1656 and ⌬p19 mutants versus the wild-type control was performed using the Student’s t test with Welch’s correction, where indicated. ****, P ⬍ 0.0001. comparison to neutral pH, while growth of the wild-type and complement strains was unaffected by the pH 5 condition (Fig. 5A). The acid sensitivity phenotype of the mutants was observed in media both with and without 100 M iron(III) citrate supple- mentation; however, iron supplementation partially restored growth of the mutants (Fig. 5A). Iron is more soluble at lower pH, which may contribute to the improved growth of mutants in acidic medium supplemented with iron(III) citrate. To assess whether this iron uptake system was required for antibiotic resistance, the MICs of different antibiotics representing multiple classes (aminoglycosides, ampheni- cols, -lactams, cationic peptides, fluoroquinolones, and macrolides) were screened for wild-type and ⌬1651–1656 deletion mutant strains using standard doubling dilution assays. Most classes showed ⱕ2-fold differences from wild type, except aminoglyco- sides, the most notable of which was streptomycin (STM) (data not shown). To test STM susceptibility in more depth, cells were inoculated into MH or MH supplemented with 100 M iron(III) citrate containing no STM or doubling dilutions of STM from 0.02 to 16 g/ml and grown for 48 h. In iron-unsupplemented MH, the MICs observed for the ⌬1651–1656 (2 g/ml) and ⌬p19 (2 g/ml) mutants were 8 times lower than the STM MIC for the wild-type and complemented strains (16 g/ml). Supplementation of MH with excess iron restored the MICs of the ⌬1651–1656 and ⌬p19 mutants (8 and 16 g/ml, respectively) to wild-type levels (16 g/ml). To expand our analysis of STM sensitivity, cell densities (optical density at 600 nm [OD600]) were measured under increasing concentrations of STM both with and without iron supplementation (Fig. 5B and C). A bimodal growth phenotype was observed for wild-type and complemented strains both with and without iron supplementation (Fig. 5B and C). This bimodal phenotype consisted of STM-sensitive growth between 0 and 1 g/ml, where cell density decreased rapidly from 100% to ⬍40% compared to growth with no STM, and then STM-tolerated growth between 1 and 8 g/ml. Unlike the wild-type and comple- mented strains, the ⌬1651–1656 and ⌬p19 mutants exhibited monomodal growth July/August 2018 Volume 9 Issue 4 e01347-18 mbio.asm.org 9 ® Liu et al. Downloaded from http://mbio.asm.org/ on October 28, 2020 at UNIVERSITY OF READING FIG 5 The role of 1651–1656 in C. jejuni survival under acid, oxidative (H2O2), and antibiotic (streptomycin) stress in comparison to P19. (A) Acid stress: comparison of growth in MH or in MH at pH 5 with and without 100 M iron(III) citrate supplementation after 24 h (B and C) of antibiotic stress. Shown is the percentage of cell growth (based on OD600) in doubling dilutions of streptomycin compared to the no-streptomycin control when incubated in MH (B) and MH supplemented with 100 M iron(III) citrate (C) for 48 h. (D and E) Oxidative stress. Shown is the viability of C. jejuni strains over 6 h after H2O2 addition in MH alone (D) or MH supplemented with iron(III) citrate (E). C. jejuni strains are shown as the wild type (black solid line), the ⌬1651–1656 mutant (red dotted line), the 1651–1656C complement (red solid line), the ⌬p19 mutant (blue dotted line), and the p19C complement (blue solid line). Error bars represent the standard deviation from 3 replicates from a total of 3 experiments. Statistical comparison of the ⌬1651–1656 and ⌬p19 mutants to the wild-type 81-176 strain was performed using Student’s t test with Welch’s correction. ***, P ⬍ 0.0005; ****, P ⬍ 0.0001. under increasing STM concentrations in iron-limited MH, with both strains becoming undetectable at 2 g/ml STM (Fig. 5B). Iron supplementation restored bimodal growth for both mutants (Fig. 5C). To examine if this operon had an effect on oxidative stress, wild-type strain 81-176, the ⌬p19 and ⌬1651–1656 mutants, and their respective complements were grown in medium alone or medium supplemented with 100 M iron(III) citrate for 6 h, at which time H2O2 was added to a final concentration of 1 mM. Cell viability was monitored at July/August 2018 Volume 9 Issue 4 e01347-18 mbio.asm.org 10 ® C. jejuni, Host Gut Extracts, and Iron Downloaded from http://mbio.asm.org/ on October 28, 2020 at UNIVERSITY OF READING FIG 6 Growth of C. jejuni strains in iron-depleted medium and with extract supplementation. Shown is C. jejuni growth in control medium (MH; black), iron-limited medium (MH plus 15 M DFO [gray]), and iron-limited medium supplemented with extracts (10% CP/HP1/HP2/HP3 [CP, pink; HP1, light blue; HP2, medium blue; HP3, dark blue) or iron(III) citrate (10 M [black dashed/striped). (A) Growth of wild-type C. jejuni over 48 h. Statistical significance was calculated in comparison to the MH control. (B) Growth of wild-type, deletion mutant, and complemented strains at 24 h. Statistics were calculated in comparison to wild-type strain 81-176 for each growth condition. For both panels A and B, P values are as follows: *, P ⬍ 0.05; **, P ⬍ 0.005; ***, P ⬍ 0.0005; ****, P ⬍ 0.0001. 3 h and 6 h after H2O2 addition. The mutants were more resistant to H2O2 stress than the wild-type or complemented strains when grown in MH and in iron-supplemented MH, remaining viable at 3 h and 6 h post-H2O2 addition, whereas the wild-type and complemented strains were no longer recoverable by 3 h (Fig. 5D and E). The 1649 –1656 system is required for optimal iron acquisition from human and chicken extracts. To determine whether the 1649 –1656 system is involved in acquisition of iron found in extracts, we grew the wild-type and mutant strains in iron-limited MH (15 M DFO) or iron-limited MH supplemented with 10% chicken or human extracts. Growth in MH and iron-limited MH supplemented with 10 M iron(III) citrate was included as a control. The DFO concentration of 15 M was selected to allow optimal C. jejuni responsiveness to iron supplementation based on optimization testing, and the iron(III) citrate concentration of 10 M was selected to approximate the maximum amount of iron expected in the extract samples. After 24 h, wild-type and complemented C. jejuni strains showed reduced growth under iron restriction in comparison to the MH control, which was rescued by supplementation with human extracts or iron(III) citrate (Fig. 6A; see Fig. S2A to F in the supplemental material). Notably, supplementation with chicken cecal extract was not sufficient to restore wild-type growth at 24 h, but did improve wild-type growth at 48 h in comparison to iron-limited medium alone (Fig. 6A; Fig. S2C and F). These results suggest that wild-type C. jejuni can utilize iron present in the extracts and that more accessible iron is present in human fecal than in chicken cecal extracts. The ⌬p19 and ⌬1651–1656 deletion strains were also deficient for growth in iron-restricted MH. However, supplementation with human fecal extracts did not restore bacterial growth to levels observed in MH July/August 2018 Volume 9 Issue 4 e01347-18 mbio.asm.org 11 ® Liu et al. alone or iron-limited MH supplemented with iron(III) citrate (Fig. 6B; Fig. S2A to F). This demonstrated that the 1649 –1656 system is required for optimal acquisition of iron from human fecal extracts. DISCUSSION The ability of C. jejuni to colonize both chicken ceca and human large intestines to high concentrations (7, 8, 12) is reflected in this study, where supplementation of MH Downloaded from http://mbio.asm.org/ on October 28, 2020 at UNIVERSITY OF READING with extracts prolonged C. jejuni survival in broth culture. The lower biofilm formation in extracts, which may provide additional nutrients, is consistent with studies indicating that C. jejuni biofilm formation is less abundant in richer media (62). We collected intestinal extracts using water as a diluent, which preferentially extracts polar com- pounds such as amino acids, carbohydrates, alcohols, and short-chain fatty acids (63). Accordingly, C. jejuni cells exposed to these extracts responded by modulating expres- sion of many genes related to metabolism, nutrient uptake, energy production, and chemotaxis. Other diluents used to prepare fecal extracts, such as methanol, acetoni- trile, and ethyl acetate, preferentially extract aromatic compounds and lipids (16, 19, 63, 64). Future assessments may benefit from using different diluents to determine whether additional biologically active metabolites also impact pathogen response. In this study, C. jejuni exposed to extracts, and in particular human fecal extracts, showed higher expression of genes related to iron uptake and utilization than C. jejuni in medium alone. A recent study of C. jejuni gene expression in human feces postcolo- nization also found that genes involved in iron acquisition were more highly expressed: notably, 6 out of 8 genes in the 1649 –1656 operon were identified (65). Iron is an essential micronutrient for most organisms, with many producing high-affinity iron chelating proteins and siderophores to scavenge iron. C. jejuni does not produce siderophores, but does possess at least 5 distinct systems to import iron from exoge- nous chelators (41). These systems transport iron bound to enterobactin (CfrA, CfrB, CeuBCDE [66, 67]), heme (ChuABCDZ ), lactoferrin/transferrin (CtuA, CfbpABC, and ChaN ), ferrous ions (FeoB ), and potentially bound to rhodotorulic acid (Cj1658⫺1663 in strain 11168, which are homologues of 1649 –1654 in strain 81-176 ). C. jejuni globally regulates expression of the majority of its iron uptake systems through the iron-responsive Fur regulator (67, 70, 71). The specific increase in 1649 – 1655 expression during exposure to human extracts and during human colonization, rather than a global response to low iron availability, suggests that other regulatory mechanisms target this system in addition to the Fur-based response. Furthermore, 1656, which shares an overlapping reading frame with 1655, actually showed a small decrease in expression during C. jejuni exposure to extracts, which may suggest even more complex regulation mechanisms at play. It remains to be determined why the 1649 –1655 operon was more highly expressed during C. jejuni exposure to extracts, especially human extracts, which contain more iron than the chicken cecal extracts. One hypothesis is that the iron present in the extracts, particularly human fecal extracts, is chelated to a compound that is specifically recognized by this iron uptake system. Indeed, in medium depleted of iron, supple- mentation with human extracts was able to fully restore growth of wild-type C. jejuni but not of the ⌬p19 or ⌬1651–1656 mutant. The 1649 –1656 system was suggested to import iron bound to rhodotorulic acid, a fungal hydroxamate siderophore (reported as unpublished data in reference 31). However, other studies show that C. jejuni is unable to utilize iron bound to rhodotorulic acid for growth (72, 73); therefore, other chelators must be considered. In Yersinia pestis, mutations in fetMP (homologues of C. jejuni 1649 and p19) resulted in iron-deficient growth that could only be restored when the downstream genes y2367 to y2362 (homologues of C. jejuni 81-176 genes 1651–1655) were included in the complementation strain (43). Here, genes downstream of p19 (1651–1656) were also required for C. jejuni growth under iron-restricted conditions. Furthermore, the wide- spread distribution and genetic conservation of the 1649 –1656 cluster in bacteria with a variety of host preferences (e.g., humans, plants, cows, and bees) and ecologic niches July/August 2018 Volume 9 Issue 4 e01347-18 mbio.asm.org 12 ® C. jejuni, Host Gut Extracts, and Iron (e.g., freshwater, seawater, soil, and thermal site) suggests that this entire system is used for iron uptake in many contexts. An analysis of the diversity and phylogenetic evolution of this iron uptake system, together with follow-up genetic and biochemical analyses, will allow better understanding of its role in bacteria. Iron uptake in C. jejuni may be generally related to acid tolerance and antibiotic survival. Transcriptomic and proteomic studies have shown that this iron uptake system, in addition to several other iron uptake genes (cfbpA/B, ceuE, and chuZ), was Downloaded from http://mbio.asm.org/ on October 28, 2020 at UNIVERSITY OF READING more highly expressed upon C. jejuni exposure to acid stress (74, 75). In addition, deletion of fur increased C. jejuni sensitivity to acidic conditions (76). Paradoxically, however, C. jejuni acid sensitivity caused by deletion of p19, 1651–1656, and fur was independent of iron availability in the growth medium, further indicating that addi- tional regulatory mechanisms must be considered. Variability in iron availability and disturbance of bacterial iron homeostasis have also been shown to impact antibiotic efficacy in various bacteria (77). However, since the C. jejuni 81-176 strain has not been reported to carry streptomycin resistance genes, the mechanism of streptomycin tolerance and potential contribution of iron to this extended tolerance are unknown. The interplay between acid stress and iron homeostasis remains an interesting avenue for future research. Additional information pertaining to how C. jejuni responds to these stresses and the implications for resistance to streptomycin can be gained from genomewide expression analyses of C. jejuni under a combination of acid and iron stresses. Unexpectedly, the 1649 –1656 system adversely affected the C. jejuni response to H2O2 independent of the addition of iron. C. jejuni responds to oxidative stress and iron limitation by inducing expression of a common subset of genes (e.g., katA, sodB, and ahpC) that are controlled by both the Fur and PerR regulons (78, 79). Thus, loss of the 1649 –1656 iron uptake system may cause constitutive cellular stress that elevated the baseline expression of genes contributing to oxidative stress tolerance. Alternatively, the presence of iron and copper binding proteins in the 1649 –1656 system may directly render C. jejuni more sensitive to H2O2-mediated cell death by formation of highly reactive and damaging intermediates upon exposure to H2O2 (25, 80). Exploring whether related conditions such as nitrosative stress yield similar results would be interesting, as would examining the effects of combining the ⌬1651–1656 or ⌬p19 mutations with mutations in genes known to be required for oxidative stress survival. An updated model of the 1649 –1656 iron uptake system is proposed based on bioinformatic analyses, showing the presence of signal peptides, transmembrane re- gions, and protein domains (Fig. 7). The 1649 –1656 gene products are hypothesized to form one functional system based on comparable phenotypes observed between the ⌬1651–1656 and ⌬p19 mutants and conservation of the gene cluster in multiple bacterial phyla. Of note, the 1651 membrane protein has a periplasmic DUF2318 domain, encoding a conserved CxxC-x(13)-CxxC-x(14,15)-C motif (see Fig. S3A and B in the supplemental material). Conserved cysteines with regular spacing are often observed in iron-sulfur cluster binding domains, such as those seen in the Fer2 and Fer4 families (Fig. S3C and D). Furthermore, we found that genes encoding DUF2318-containing proteins almost always mapped to gene clusters with homo- logues of 1649 –1656 in different bacterial species, indicating association with this bacterial iron uptake system. Ongoing experiments aimed toward single gene deletions and domain or residue specific substitutions should help determine the identity of transported substrates, protein-protein interactions, and mechanism(s) of iron trans- port. In sum, this study is the first to compare the bacterial transcriptional and functional responses to gut extracts from a disease-susceptible host (humans) and a zoonotic host (chicken). RNA sequencing identified genes that may be important for C. jejuni to colonize both chickens and humans, with subsequent biological analyses demonstrat- ing that the 1649 –1656 iron uptake system was necessary for C. jejuni growth under iron-depleted conditions, impacted growth and survival under specific stress-related conditions, and was required for acquisition of iron from human fecal extracts. Given July/August 2018 Volume 9 Issue 4 e01347-18 mbio.asm.org 13 ® Liu et al. Downloaded from http://mbio.asm.org/ on October 28, 2020 at UNIVERSITY OF READING FIG 7 Model of the C. jejuni 1649 –1656 iron uptake system. We hypothesize that this iron acquisition system imports iron (yellow stars) that is bound to a specific, but as yet unidentified, chelator (light blue hexagons). Gray hexagons represent other iron chelators. Since the gene cluster does not appear to encode an outer membrane receptor, Chan et al. suggested that iron is transported across the outer membrane by an as yet unidentified receptor (gray box) before binding to P19 (dark blue) in the periplasm (25). During iron uptake, iron-bound P19 may interact with the periplasmic metal binding domains of 1651 (brown), followed by an iron reduction step involving the 1655 and 1656 thioredoxins (light and dark green), prior to being transported into the cytoplasm via the 1649 inner membrane transporter (black). The substrates transported by the 1651 membrane protein and the 1652 and 1653 permeases (pink and red), with the help of the 1654 ATPase (purple), are unknown. We hypothesize that the chelated form of iron acquired by this system is more abundant in human than in chicken intestinal environments. the system’s conservation in a wide range of bacteria, the majority of which colonize and infect humans, this work should be broadly relevant to the general bacteriology and pathogenesis communities as well. Collectively, we showed that investigation of pathogen gene expression in response to exposure to the host metabolome, together with follow-up genotypic and phenotypic analyses, is not only an informative method of studying host-pathogen interactions but should improve our understanding of the mechanisms employed by pathogens to cause disease. MATERIALS AND METHODS Ethics statement. Written and informed consent was obtained from all human fecal sample donors as described in ethics application H14-00859, which was approved by the University of British Columbia Clinical Research Ethics Board in Vancouver, Canada. Bacterial strains and growth conditions. C. jejuni strain 81-176, a pathogenic and common laboratory reference strain, was used in this study (9). The C. jejuni ⌬1651–1656 mutant strain and corresponding 1651–1656C complemented strain were constructed as described in Text S1 in the supplemental material, using primers listed in Table S4 in the supplemental material. The ⌬p19 deletion and complemented (p19C) strains were obtained from reference 25. C. jejuni strains were grown in MH (Oxoid) broth or agar (1.5% [wt/vol]). MH was supplemented with antibiotics where appropriate: vancomycin (V; 10 g/ml), trimethoprim (T; 5 g/ml), kanamycin (Km; 50 g/ml), and chloramphenicol (Cm; 20 g/ml). Agar plates and standing cultures were incubated at 38°C under microaerobic and capnophilic conditions (12% CO2 and 6% O2 in N2) in a Sanyo tri-gas incubator. Shaking broth cultures (including 15- to 18-h overnight cultures) were incubated microaerobically in airtight containers with the Oxoid CampyGen atmosphere generation system at 38°C and shaken at 200 rpm. Cell concentration was measured by dilution plating; the limit of detection for this method is 103 CFU/ml. Escherichia coli strain DH5␣ (Invitrogen) was used for cloning. E. coli was grown aerobically at 37°C and shaken at 200 rpm in Luria-Bertani broth (LB; Sigma) or incubated aerobically at 37°C on LB agar (1.5% [wt/vol]) supplemented with Cm (20 g/ml) and Km (50 g/ml) for selection, as required. July/August 2018 Volume 9 Issue 4 e01347-18 mbio.asm.org 14 ® C. jejuni, Host Gut Extracts, and Iron Growth and biofilm formation in extracts. Human fecal and chicken cecal extracts were prepared as described in Text S1. For liquid growth cultures, overnight log-phase C. jejuni cells were inoculated at an OD600 of 0.005 in a final volume of 1 ml in MH supplemented with trimethoprim and vancomycin (MH-TV) or MH-TV plus 30% extract. MH-TV plus 30% extract conditions were prepared by combining 0.5 ml of 2⫻ MH-TV containing C. jejuni at an OD600 of 0.01, 0.3 ml of extract (CP, HP1, HP2, or HP3), and 0.2 ml of sterile ultrapure H2O (0.5 ml for the control MH-TV condition). Cultures were incubated microaerobically at 38°C and shaken. The cell concentration was measured by dilution plating at the indicated times. For biofilm formation, overnight log-phase C. jejuni cells were inoculated at an OD600 of Downloaded from http://mbio.asm.org/ on October 28, 2020 at UNIVERSITY OF READING 0.02 into 1 ml of MH-TV or MH-TV containing 10% extract (CP, HP1, HP2, HP3, or H2O for the control) in borosilicate glass tubes. The cultures were incubated microaerobically at 38°C without agitation. The planktonic cell concentration was measured by dilution plating 20 l of culture sampled from 2 mm below the liquid surface. The biofilms were quantified as detailed in Text S1. Experiments were performed in triplicate. RNA preparation. C. jejuni overnight cultures were reinoculated into fresh MH-TV broth at an OD600 of 0.04 and incubated microaerobically shaking at 38°C for 4 to 5 h in order to obtain optimal log growth (approximate OD600 of 0.2). C. jejuni cells were inoculated into MH-TV or MH-TV plus 30% extract (CP, HP1, HP2, or HP3) to a final volume of 1 ml at an OD600 of 0.25 for the 20-min conditions and OD600 0.06 for the 5-h conditions in order to ensure comparable numbers of cells during harvest and RNA preparation. RNA was extracted from these 10 independent conditions performed in duplicate on separate days as outlined in Text S1. RNA from all samples was extracted by adding 1:10 (vol/vol) stop solution (5% phenol in ethanol) and was extracted as described previously (81). Samples were shipped to the Wellcome Trust Sanger Institute (WTSI), and RNA was sequenced and analyzed as described in Text S1. Total iron content of extracts. ICP-MS was used to measure the iron content in human fecal and chicken cecal extracts. Briefly, 0.5 ml each of CP, HP1, HP2, and HP3 was added in duplicate into microcentrifuge tubes and dried overnight using a SpeedVac concentrator (DNA 120; Thermo) at low power. Samples were then analyzed using ICP-MS. Briefly, samples were digested in 1% nitric acid and heated in a closed Savillex vessel using a hot plate. Scandium (Sc) and indium (In) at 100 ppb were added as internal standards. ICP-MS was conducted using the PerkinElmer NexIon 300D ICP-MS instrument. Protein domains and homologues. The Simple Modular Architecture Research Tool (SMART) in Genomic mode was used to determine protein domains, signal peptides, and PFAM domains for the amino acid sequences of 1649 –1656 (http://smart.embl.de/) (82). Protter was used to visualize the protein placement in two-dimensional (2D) space (83). Homologues of 1649 –1656 were identified using the NCBI online database with the blastn and blastp suites. To identify homologues in non- Campylobacter organisms, the “Campylobacter (taxid: 194)” group was excluded, and fully sequenced and annotated genomes of bacteria from each unique genus listed were downloaded from GenBank and visualized using Artemis. The amino acid sequences for each protein were aligned using MUSCLE and assessed for homology using MEGA 7.0.21 and BioEdit Sequence Alignment Editor, and conserved residues were visualized using the online tool Skylign (http://skylign.org/). Measuring growth under iron depletion, acid, streptomycin, and H2O2 stresses. Log-phase C. jejuni cells of the 81-176, ⌬1651–1656, 1651–1656C, ⌬p19, and p19C strains from 15- to 18-h overnight cultures were inoculated at an OD600 of 0.005 into 3 ml of medium unless otherwise indicated. For growth under iron-depleted versus replete conditions, cells were inoculated into MH (unsupplemented), MH containing 20 M ferric iron chelator DFO (iron poor), or MH supplemented with 100 M iron(III) citrate (iron rich). For H2O2 stress, cells were inoculated into MH or MH plus 100 M iron(III) citrate and incubated for 6 h, and then H2O2 (Sigma) was added to a final concentration of 1 mM H2O2. For acid stress, cells were inoculated into MH or MH at pH 5, prepared by adding 1 N HCl into MH and verified using a pH meter (SB20; VWR), with and without supplementation with 100 M iron(III) citrate. For these growth experiments, cells were incubated shaking microaerobically at 38°C, and cell viability was measured by dilution plating at the indicated times. For antibiotic testing, cells were inoculated into 96-well plates at a final OD600 of 0.02 in 0.2 ml of MH or MH plus 100 M iron(III) citrate containing doubling dilutions of streptomycin from 0.03 to 16 M. The plates were incubated at 38°C for 48 h. The wells were mixed by pipetting and measured using the Varioscan Flash spectrophotometer at OD600. All experiments were performed in triplicate, with each experiment including three technical replicates. Measuring growth in iron-depleted medium supplemented with extracts. Log-phase C. jejuni cells of the 81-176, ⌬1651–1656, 1651–1656C, ⌬p19, and p19C strains were inoculated at a final OD600 of 0.005 into 200 l of MH, MH plus 15 M DFO, MH plus 15 MDFO plus 10% CP/HP1/HP2/HP3, or MH plus 15 M DFO plus 10 M iron(III) citrate in 96-well plates. Plates were incubated at 38°C microaerobically. At each time point, the wells were mixed, cell density was measured at OD600, and CFU measured by dilution plating 10 l of each culture. Experiments were performed in triplicate. Statistics. The P values for gene expression fold change derived from RNA sequencing data were calculated by the DESeq2 package. Briefly, DESeq2 uses the Benjamini-Hochberg (BH) adjustment to calculate the padj value, which represents the false-discovery rate of genes should the null hypothesis (i.e., no genes are affected) be true (84). Genes with padj values (shown as P values in Tables 1 to 3; see Table S2) lower than 0.05 were selected for this study. C. jejuni growth and biofilm data were analyzed and graphed using Graphpad Prism 7, and statistical differences were calculated using the Student’s t test. Statistical analysis of the ICP-MS data was calculated using analysis of variance (ANOVA). Availability of data. The transcriptome (RNA-seq) sequence data for this study have been submitted to the European Nucleotide Archive (ENA). The accession numbers can be found in Table S1. July/August 2018 Volume 9 Issue 4 e01347-18 mbio.asm.org 15 ® Liu et al. SUPPLEMENTAL MATERIAL Supplemental material for this article may be found at https://doi.org/10.1128/mBio.01347-18. TEXT S1, DOCX file, 0.1 MB. TEXT S2, DOCX file, 0.1 MB. FIG S1, TIF file, 2.2 MB. FIG S2, EPS file, 1.2 MB. Downloaded from http://mbio.asm.org/ on October 28, 2020 at UNIVERSITY OF READING FIG S3, TIF file, 2.5 MB. TABLE S1, DOCX file, 0.1 MB. TABLE S2, DOCX file, 0.1 MB. TABLE S3, DOCX file, 0.1 MB. TABLE S4, DOCX file, 0.1 MB. ACKNOWLEDGMENTS We acknowledge and thank all the members of the Gaynor lab and Charles Thompson for discussions and technical support, especially Emilisa Frirdich and Jenny Vermeulen for critical reviews and Reuben Ha for support with sample processing. We thank Neil Ambrose (Sunrise Farms) for providing us with chicken gut sacks and all volunteers for their donation of samples and time. We thank the core sequencing teams at the Sanger Institute for performing the RNA sequencing, and Mariko Ikehata for processing the ICP-MS samples. This work was funded by Canadian Institutes of Health Research (CIHR) grants MOP-68981 to E.C.G. and MOP-49597 to M.E.P.M., CIHR grant GSM-136327 to M.L., Canada Graduate Scholarship—Michael Smith Foreign Study Supplement 2014-338587 to M.L, and Medical Research Council grant G1100100/1 to J.P. Sequencing was supported by Wellcome Trust grant WT098051 to J.P. The funders had no role in study design, data collection and interpretation, or the decision to submit the work for publication. The authors declare that they have no conflicts of interest with the contents of this article. REFERENCES 1. Kaakoush NO, Castaño-Rodríguez N, Mitchell HM, Man SM. 2015. Global point-source outbreak of campylobacteriosis associated with consump- epidemiology of Campylobacter infection. Clin Microbiol Rev 28: tion of raw milk. J Infect Dis 152:592–596. https://doi.org/10.1093/infdis/ 687–720. https://doi.org/10.1128/CMR.00006-15. 152.3.592. 2. Thomas MK, Murray R, Flockhart L, Pintar K, Pollari F, Fazil A, Nesbitt A, 10. Moffatt CR, Greig A, Valcanis M, Gao W, Seemann T, Howden BP, Kirk MD. Marshall B. 2013. Estimates of the burden of foodborne illness in Canada 2016. A large outbreak of Campylobacter jejuni infection in a university for 30 specified pathogens and unspecified agents, circa 2006. Food- college caused by chicken liver pate, Australia, 2013. Epidemiol Infect borne Pathog Dis 10:639 – 648. https://doi.org/10.1089/fpd.2012.1389. 144:2971–2978. https://doi.org/10.1017/S0950268816001187. 3. Mohan V. 2015. Faeco-prevalence of Campylobacter jejuni in urban wild 11. Black RE, Levine MM, Clements ML, Hughes TP, Blaser MJ. 1988. Exper- birds and pets in New Zealand. BMC Res Notes 8:1. https://doi.org/10 imental Campylobacter jejuni infection in humans. J Infect Dis 157:.1186/1756-0500-8-1. 472– 479. https://doi.org/10.1093/infdis/157.3.472. 4. Ramonaitė S, Novoslavskij A, Zakarienė G, Aksomaitienė J, Malakauskas 12. Tribble DR, Baqar S, Scott DA, Oplinger ML, Trespalacios F, Rollins D, M. 2015. High prevalence and genetic diversity of Campylobacter jejuni Walker RI, Clements JD, Walz S, Gibbs P, Burg EF, III, Moran AP, Applebee in wild crows and pigeons. Curr Microbiol 71:559 –565. https://doi.org/ L, Bourgeois AL. 2010. Assessment of the duration of protection in 10.1007/s00284-015-0881-z. Campylobacter jejuni experimental infection in humans. Infect Immun 5. Pintar KDM, Christidis T, Thomas MK, Anderson M, Nesbitt A, Keithlin J, 78:1750 –1759. https://doi.org/10.1128/IAI.01021-09. Marshall B, Pollari F. 2015. A systematic review and meta-analysis of the 13. Kirkpatrick BD, Lyon CE, Porter CK, Maue AC, Guerry P, Pierce KK, Campylobacter spp. prevalence and concentration in household pets Carmolli MP, Riddle MS, Larsson CJ, Hawk D, Dill EA, Fingar A, Poly F, and petting zoo animals for use in exposure assessments. PLoS One Fimlaid KA, Hoq F, Tribble DR. 2013. Lack of homologous protection 10:e0144976. https://doi.org/10.1371/journal.pone.0144976. 6. Wright SL, Carver DK, Siletzky RM, Romine S, Morrow WEM, Kathariou S. against Campylobacter jejuni CG8421 in a human challenge model. Clin 2008. Longitudinal study of prevalence of Campylobacter jejuni and Infect Dis 57:1106 –1113. https://doi.org/10.1093/cid/cit454. Campylobacter coli from turkeys and swine grown in close proximity. J 14. Keithlin J, Sargeant J, Thomas MK, Fazil A. 2014. Systematic review and Food Prot 71:1791–1796. https://doi.org/10.4315/0362-028X-71.9.1791. meta-analysis of the proportion of Campylobacter cases that develop 7. Bahrndorff S, Garcia AB, Vigre H, Nauta M, Heegaard PMH, Madsen M, chronic sequelae. BMC Public Health 14:1203. https://doi.org/10.1186/ Hoorfar J, Hald B. 2015. Intestinal colonization of broiler chickens by 1471-2458-14-1203. Campylobacter spp. in an experimental infection study. Epidemiol Infect 15. Heikema AP, Islam Z, Horst-Kreft D, Huizinga R, Jacobs BC, Wagenaar JA, 143:2381–2389. https://doi.org/10.1017/S0950268814003239. Poly F, Guerry P, van Belkum A, Parker CT, Endtz HP. 2015. Campylobac- 8. Pielsticker C, Glünder G, Aung YH, Rautenschlein S. 2016. Colonization ter jejuni capsular genotypes are related to Guillain-Barre syndrome. Clin pattern of C. jejuni isolates of human and avian origin and differences in Microbiol Infect 21:852.e1– 852.e9. https://doi.org/10.1016/j.cmi.2015.05 the induction of immune responses in chicken. Vet Immunol Immuno-.031. pathol 169:1–9. https://doi.org/10.1016/j.vetimm.2015.11.005. 16. Antunes LC, Han J, Ferreira RB, Lolić P, Borchers CH, Finlay BB. 2011. 9. Korlath JA, Osterholm MT, Judy LA, Forfang JC, Robinson RA. 1985. A Effect of antibiotic treatment on the intestinal metabolome. Antimi- July/August 2018 Volume 9 Issue 4 e01347-18 mbio.asm.org 16 ® C. jejuni, Host Gut Extracts, and Iron crob Agents Chemother 55:1494 –1503. https://doi.org/10.1128/AAC host cell receptor. BMC Microbiol 14:141. https://doi.org/10.1186/1471.01664-10. -2180-14-141. 17. Vernocchi P, Del Chierico F, Putignani L. 2016. Gut microbiota profiling: 36. Pryjma M, Apel D, Huynh S, Parker CT, Gaynor EC. 2012. FdhTU- metabolomics based approach to unravel compounds affecting human modulated formate dehydrogenase expression and electron donor health. Front Microbiol 7:1144. https://doi.org/10.3389/fmicb.2016 availability enhance recovery of Campylobacter jejuni following host.01144. cell infection. J Bacteriol 194:3803–3813. https://doi.org/10.1128/JB 18. Jansson J, Willing B, Lucio M, Fekete A, Dicksved J, Halfvarson J, Tysk C,.06665-11. Schmitt-Kopplin P. 2009. Metabolomics reveals metabolic biomarkers of 37. Shaw FL, Mulholland F, Le Gall G, Porcelli I, Hart DJ, Pearson BM, van Vliet Crohn’s disease. PLoS One 4:e6386. https://doi.org/10.1371/journal.pone AHM. 2012. Selenium-dependent biogenesis of formate dehydrogenase Downloaded from http://mbio.asm.org/ on October 28, 2020 at UNIVERSITY OF READING.0006386. in Campylobacter jejuni is controlled by the fdhTU accessory genes. J 19. Antunes LC, McDonald JA, Schroeter K, Carlucci C, Ferreira RB, Wang M, Bacteriol 194:3814 –3823. https://doi.org/10.1128/JB.06586-11. Yurist-Doutsch S, Hira G, Jacobson K, Davies J, Allen-Vercoe E, Finlay BB. 38. Tom-Yew SA, Cui DT, Bekker EG, Murphy ME. 2005. Anion-independent 2014. Antivirulence activity of the human gut metabolome. mBio iron coordination by the Campylobacter jejuni ferric binding protein. J 5:e01183-14. https://doi.org/10.1128/mBio.01183-14. Biol Chem 280:9283–9290. https://doi.org/10.1074/jbc.M412479200. 20. Pan D, Yu Z. 2014. Intestinal microbiome of poultry and its interaction 39. Richardson PT, Park SF. 1995. Enterochelin acquisition in Campylobacter with host and diet. Gut Microbes 5:108 –119. https://doi.org/10.4161/ coli: characterization of components of a binding-protein-dependent gmic.26945. transport system. Microbiology 141:3181–3191. https://doi.org/10.1099/ 21. Kościuczuk EM, Lisowski P, Jarczak J, Strzałkowska N, Jóźwik A, Horbańczuk 13500872-141-12-3181. J, Krzyżewski J, Zwierzchowski L, Bagnicka E. 2012. Cathelicidins: family of 40. Ridley KA, Rock JD, Li Y, Ketley JM. 2006. Heme utilization in Campylo- antimicrobial peptides. A review. Mol Biol Rep 39:10957–10970. https:// bacter jejuni. J Bacteriol 188:7862–7875. https://doi.org/10.1128/JB doi.org/10.1007/s11033-012-1997-x..00994-06. 22. Lei F, Yin YS, Wang YZ, Deng B, Yu HD, Li LJ, Xiang C, Wang SY, Zhu BL, 41. Miller CE, Williams PH, Ketley JM. 2009. Pumping iron: mechanisms for Wang X. 2012. Higher-level production of volatile fatty acids in vitro by iron uptake by Campylobacter. Microbiology 155:3157–3165. https://doi chicken gut microbiotas than by human gut microbiotas as determined.org/10.1099/mic.0.032425-0. by functional analyses. Appl Environ Microbiol 78:5763–5772. https://doi 42. Brickman TJ, Armstrong SK. 2012. Iron and pH-responsive FtrABCD.org/10.1128/AEM.00327-12. ferrous iron utilization system of Bordetella species. Mol Microbiol 86: 23. Stahl M, Butcher J, Stintzi A. 2012. Nutrient acquisition and metabolism 580 –593. https://doi.org/10.1111/mmi.12003. by Campylobacter jejuni. Front Cell Infect Microbiol 2:5. https://doi.org/ 43. Fetherston JD, Mier I, Jr, Truszczynska H, Perry RD. 2012. The Yfe and Feo 10.3389/fcimb.2012.00005. transporters are involved in microaerobic growth and virulence of Yer- 24. Andreini C, Bertini I, Cavallaro G, Holliday GL, Thornton JM. 2008. Metal sinia pestis in bubonic plague. Infect Immun 80:3880 –3891. https://doi ions in biological catalysis: from enzyme databases to general principles..org/10.1128/IAI.00086-12. 44. Dugar G, Herbig A, Förstner KU, Heidrich N, Reinhardt R, Nieselt K, J Biol Inorg Chem 13:1205–1218. https://doi.org/10.1007/s00775-008 Sharma CM. 2013. High-resolution transcriptome maps reveal strain- -0404-5. specific regulatory features of multiple Campylobacter jejuni isolates. 25. Chan AC, Doukov TI, Scofield M, Tom-Yew SA, Ramin AB, Mackichan JK, PLoS Genet 9:e1003495. https://doi.org/10.1371/journal.pgen.1003495. Gaynor EC, Murphy ME. 2010. Structure and function of P19, a high- 45. Inglis GD, Boras VF, Houde A. 2011. Enteric campylobacteria and RNA affinity iron transporter of the human pathogen Campylobacter jejuni. J viruses associated with healthy and diarrheic humans in the Chinook Mol Biol 401:590 – 604. https://doi.org/10.1016/j.jmb.2010.06.038. health region of southwestern Alberta, Canada. J Clin Microbiol 49: 26. Cameron A, Frirdich E, Huynh S, Parker CT, Gaynor EC. 2012. Hyperos- 209 –219. https://doi.org/10.1128/JCM.01220-10. motic stress response of Campylobacter jejuni. J Bacteriol 194: 46. Levesque S, Lemay F, Bekal S, Frost EH, Michaud S. 2016. First reported 6116 – 6130. https://doi.org/10.1128/JB.01409-12. case of Campylobacter lanienae enteritis in a human. JMM Case Rep 27. Wösten MM, van de Lest CH, van Dijk L, van Putten JP. 2017. Function 3:e005045. https://doi.org/10.1099/jmmcr.0.005045. and regulation of the C4-dicarboxylate transporters in Campylobacter 47. Guettler MV, Rumler D, Jain MK. 1999. Actinobacillus succinogenes sp. jejuni. Front Microbiol 8:174. https://doi.org/10.3389/fmicb.2017.00174. nov., a novel succinic-acid-producing strain from the bovine rumen. Int 28. Guccione E, Leon-Kempis MDR, Pearson BM, Hitchin E, Mulholland F, van J Syst Bacteriol 49:207–216. https://doi.org/10.1099/00207713-49-1-207. Diemen PM, Stevens MP, Kelly DJ. 2008. Amino acid-dependent growth 48. Baar C, Eppinger M, Raddatz G, Simon J, Lanz C, Klimmek O, Nandakumar of Campylobacter jejuni: key roles for aspartase (AspA) under microaero- R, Gross R, Rosinus A, Keller H, Jagtap P, Linke B, Meyer F, Lederer H, bic and oxygen-limited conditions and identification of AspB (Cj0762), Schuster SC. 2003. Complete genome sequence and analysis of Wo- essential for growth on glutamate. Mol Microbiol 69:77–93. https://doi linella succinogenes. Proc Natl Acad Sci U S A 100:11690 –11695. https://.org/10.1111/j.1365-2958.2008.06263.x. doi.org/10.1073/pnas.1932838100. 29. Ferla MP, Patrick WM. 2014. Bacterial methionine biosynthesis. Microbi- 49. Engel P, Kwong WK, Moran NA. 2013. Frischella perrara gen. nov., sp. ology 160:1571–1584. https://doi.org/10.1099/mic.0.077826-0. nov., a gammaproteobacterium isolated from the gut of the honeybee, 30. Koch D, Chan AC, Murphy ME, Lilie H, Grass G, Nies DH. 2011. Charac- Apis mellifera. Int J Syst Evol Microbiol 63:3646 –3651. https://doi.org/ terization of a dipartite iron uptake system from uropathogenic Esche- 10.1099/ijs.0.049569-0. richia coli strain F11. J Biol Chem 286:25317–25330. https://doi.org/10 50. Denman S, Brady C, Kirk S, Cleenwerck I, Venter S, Coutinho T, De Vos P..1074/jbc.M111.222745. 2012. Brenneria goodwinii sp. nov., associated with acute oak decline in 31. Stintzi A, van Vliet AH, Ketley JM. 2008. Iron metabolism, transport, and the UK. Int J Syst Evol Microbiol 62:2451–2456. https://doi.org/10.1099/ regulation, p 591– 610. In Nachamkin I, Szymanski CM, Blaser MJ (ed), ijs.0.037879-0. Campylobacter, 3rd ed. ASM Press, Washington, DC. 51. Lee DH, Kim JB, Lim JA, Han SW, Heu S. 2014. Genetic diversity of 32. Pajaniappan M, Hall JE, Cawthraw SA, Newell DG, Gaynor EC, Fields JA, Pectobacterium carotovorum subsp. brasiliensis isolated in Korea. Plant Rathbun KM, Agee WA, Burns CM, Hall SJ, Kelly DJ, Thompson SA. 2008. Pathol J 30:117–124. https://doi.org/10.5423/PPJ.OA.12.2013.0117. A temperature-regulated Campylobacter jejuni gluconate dehydroge- 52. Fiala G, Woese CR, Langworthy TA, Stetter KO. 1990. Flexistipes sinusara- nase is involved in respiration-dependent energy conservation and bici, a novel genus and species of eubacteria occurring in the Atlantis II chicken colonization. Mol Microbiol 68:474 – 491. https://doi.org/10 deep brines of the Red Sea. Arch Microbiol 154:120 –126. https://doi.org/.1111/j.1365-2958.2008.06161.x. 10.1007/BF00423320. 33. Min T, Vedadi M, Watson DC, Wasney GA, Munger C, Cygler M, Matte A, 53. Liu ZP, Wang BJ, Liu XY, Dai X, Liu YH, Liu SJ. 2008. Paracoccus halophilus Young NM. 2009. Specificity of Campylobacter jejuni adhesin PEB3 for sp. nov., isolated from marine sediment of the South China Sea, China, phosphates and structural differences among its ligand complexes. and emended description of genus Paracoccus Davis 1969. Int J Syst Evol Biochemistry 48:3057–3067. https://doi.org/10.1021/bi802195d. Microbiol 58:257–261. https://doi.org/10.1099/ijs.0.65237-0. 34. Pei ZH, Ellison RT, III, Blaser MJ. 1991. Identification, purification, and 54. Munk AC, Copeland A, Lucas S, Lapidus A, Del Rio TG, Barry K, Detter JC, characterization of major antigenic proteins of Campylobacter jejuni. J Hammon N, Israni S, Pitluck S, Brettin T, Bruce D, Han C, Tapia R, Gilna P, Biol Chem 266:16363–16369. Schmutz J, Larimer F, Land M, Kyrpides NC, Mavromatis K, Richardson P, 35. Rubinchik S, Seddon AM, Karlyshev AV. 2014. A negative effect of Rohde M, Göker M, Klenk HP, Zhang Y, Roberts GP, Reslewic S, Schwartz Campylobacter capsule on bacterial interaction with an analogue of a DC. 2011. Complete genome sequence of Rhodospirillum rubrum type July/August 2018 Volume 9 Issue 4 e01347-18 mbio.asm.org 17 ® Liu et al. strain (S1). Stand Genomic Sci 4:293–302. https://doi.org/10.4056/sigs and intracellular survival. Infect Immun 74:5433–5444. https://doi.org/.1804360. 10.1128/IAI.00052-06. 55. Brown SD, Utturkar SM, Magnuson TS, Ray AE, Poole FL, Lancaster WA, 70. Holmes K, Mulholland F, Pearson BM, Pin C, McNicholl-Kennedy J, Ketley Thorgersen MP, Adams MW, Elias DA. 2014. Complete genome sequence JM, Wells JM. 2005. Campylobacter jejuni gene expression in response to of Pelosinus sp. strain UFO1 assembled using single-molecule real-time iron limitation and the role of Fur. Microbiology 151:243–257. https:// DNA sequencing technology. Genome Announc 2:e00881-14. https://doi doi.org/10.1099/mic.0.27412-0..org/10.1128/genomeA.00881-14. 71. Butcher J, Handley RA, van Vliet AH, Stintzi A. 2015. Refined analysis of 56. Luijten ML, de Weert J, Smidt H, Boschker HT, de Vos WM, Schraa G, the Campylobacter jejuni iron-dependent/independent Fur- and PerR- Stams AJ. 2003. Description of Sulfurospirillum halorespirans sp. nov., an transcriptomes. BMC Genomics 16:498. https://doi.org/10.1186/s12864 Downloaded from http://mbio.asm.org/ on October 28, 2020 at UNIVERSITY OF READING anaerobic, tetrachloroethene-respiring bacterium, and transfer of Deha- -015-1661-7. lospirillum multivorans to the genus Sulfurospirillum as Sulfurospirillum 72. Baig BH, Wachsmuth IK, Morris GK. 1986. Utilization of exogenous multivorans comb. nov. Int J Syst Evol Microbiol 53:787–793. https://doi siderophores by Campylobacter species. J Clin Microbiol 23:431– 433..org/10.1099/ijs.0.02417-0. 73. Field LH, Headley VL, Payne SM, Berry LJ. 1986. Influence of iron on 57. Aksenova HY, Rainey FA, Janssen PH, Zavarzin GA, Morgan HW. 1992. growth, morphology, outer membrane protein composition, and Spirochaeta thermophila sp. nov, an obligately anaerobic, polysaccha- synthesis of siderophores in Campylobacter jejuni. Infect Immun rolytic, extremely thermophilic bacterium. Int J Syst Bacteriol 42: 54:126 –132. 175–177. https://doi.org/10.1099/00207713-42-1-175. 74. Varsaki A, Murphy C, Barczynska A, Jordan K, Carroll C. 2015. The acid 58. Aruni AW, Mishra A, Dou Y, Chioma O, Hamilton BN, Fletcher HM. 2015. adaptive tolerance response in Campylobacter jejuni induces a global Filifactor alocis—a new emerging periodontal pathogen. Microbes Infect response, as suggested by proteomics and microarrays. Microb Biotech- 17:517–530. https://doi.org/10.1016/j.micinf.2015.03.011. nol 8:974 –988. https://doi.org/10.1111/1751-7915.12302. 59. Blekher L, Siegman-Igra Y, Schwartz D, Berger SA, Carmeli Y. 2000. 75. Birk T, Wik MT, Lametsch R, Knøchel S. 2012. Acid stress response and Clinical significance and antibiotic resistance patterns of Leminorella protein induction in Campylobacter jejuni isolates with different acid spp., an emerging nosocomial pathogen. J Clin Microbiol 38:3036 –3038. tolerance. BMC Microbiol 12:174. https://doi.org/10.1186/1471-2180-12 60. Meltzer MC, Desmond RA, Schwebke JR. 2008. Association of Mobilun- -174. cus curtisii with recurrence of bacterial vaginosis. Sex Transm Dis 35: 76. Askoura M, Sarvan S, Couture JF, Stintzi A. 2016. The Campylobacter 611– 613. https://doi.org/10.1097/OLQ.0b013e318167b105. jejuni ferric uptake regulator promotes acid survival and cross- 61. Uemura H, Hayakawa K, Shimada K, Tojo M, Nagamatsu M, Miyoshi- protection against oxidative stress. Infect Immun 84:1287–1300. https:// Akiyama T, Tamura S, Mesaki K, Yamamoto K, Yanagawa Y, Sugihara J, doi.org/10.1128/IAI.01377-15. Kutsuna S, Takeshita N, Shoda N, Hagiwara A, Kirikae T, Ohmagari N. 77. Ezraty B, Barras F. 2016. The “liaisons dangereuses” between iron and 2014. Parvimonas micra as a causative organism of spondylodiscitis: a antibiotics. FEMS Microbiol Rev 40:418 – 435. https://doi.org/10.1093/ report of two cases and a literature review. Int J Infect Dis 23:53–55. femsre/fuw004. https://doi.org/10.1016/j.ijid.2014.02.007. 78. Butcher J, Stintzi A. 2013. The transcriptional landscape of Campylobac- 62. Reeser RJ, Medler RT, Billington SJ, Jost BH, Joens LA. 2007. Character- ter jejuni under iron replete and iron limited growth conditions. PLoS ization of Campylobacter jejuni biofilms under defined growth condi- One 8:e79475. https://doi.org/10.1371/journal.pone.0079475. tions. Appl Environ Microbiol 73:1908 –1913. https://doi.org/10.1128/ 79. Palyada K, Sun YQ, Flint A, Butcher J, Naikare H, Stintzi A. 2009. Char- AEM.00740-06. 63. Jacobs DM, Deltimple N, van Velzen E, van Dorsten FA, Bingham M, acterization of the oxidative stress stimulon and PerR regulon of Cam- Vaughan EE, van Duynhoven J. 2008. 1H NMR metabolite profiling of pylobacter jejuni. BMC Genomics 10:481. https://doi.org/10.1186/1471 feces as a tool to assess the impact of nutrition on the human micro- -2164-10-481. biome. NMR Biomed 21:615– 626. https://doi.org/10.1002/nbm.1233. 80. Pham AN, Xing GW, Miller CJ, Waite TD. 2013. Fenton-like copper redox 64. Deda O, Gika HG, Wilson ID, Theodoridis GA. 2015. An overview of fecal chemistry revisited: hydrogen peroxide and superoxide mediation of sample preparation for global metabolic profiling. J Pharm Biomed Anal copper-catalyzed oxidant production. J Cat 301:54 – 64. https://doi.org/ 113:137–150. https://doi.org/10.1016/j.jpba.2015.02.006. 10.1016/j.jcat.2013.01.025. 65. Crofts AA, Poly FM, Ewing CP, Kuroiwa JM, Rimmer JE, Harro C, Sack D, 81. Gaynor EC, Cawthraw S, Manning G, MacKichan JK, Falkow S, Newell DG. Talaat KR, Porter CK, Gutierrez RL, DeNearing B, Brubaker J, Laird RM, 2004. The genome-sequenced variant of Campylobacter jejuni NCTC Maue AC, Jaep K, Alcala A, Tribble DR, Riddle MS, Ramakrishnan A, 11168 and the original clonal clinical isolate differ markedly in coloni- McCoy AJ, Davies BW, Guerry P, Trent MS. 2018. Campylobacter jejuni zation, gene expression, and virulence-associated phenotypes. J Bacte- transcriptional and genetic adaptation during human infection. Nat riol 186:503–517. https://doi.org/10.1128/JB.186.2.503-517.2004. Microbiol 3:494 –502. https://doi.org/10.1038/s41564-018-0133-7. 82. Letunic I, Doerks T, Bork P. 2015. SMART: recent updates, new develop- 66. Naikare H, B