CAD for Additive Manufacturing - IIT Kanpur
Document Details
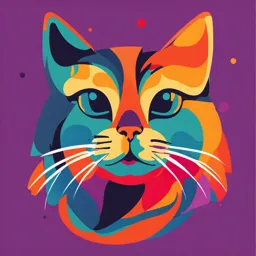
Uploaded by EfficaciousMinotaur
IIT Kanpur
Dr. J. Ramkumar
Tags
Summary
These lecture notes cover CAD for Additive Manufacturing, focusing on file formats, software, design considerations, and other key aspects of AM.
Full Transcript
EL PT N Dr. J. Ramkumar Professor Department of Mechanical Engineering and Design IIT Kanpur ▪ Printing process ▪ CAD file formats EL ▪ CAD CAM software ▪ Modelling and Data Processing ▪ AMF file format ▪ Slicing...
EL PT N Dr. J. Ramkumar Professor Department of Mechanical Engineering and Design IIT Kanpur ▪ Printing process ▪ CAD file formats EL ▪ CAD CAM software ▪ Modelling and Data Processing ▪ AMF file format ▪ Slicing PT ▪ Issues with STL file format N ▪ Design consideration ▪ Machine setup N PT EL ▪ In AM, the part to be manufactured is designed by a CAD tool EL that contains all the printing information. ▪ All AM processes begin with CAD conceptualization and model conversion into a machine-readable file. After sending ▪ PT the file to the machine, the part is printed. An experienced designer can create a CAD file via user interface, 3D scanning an existing part, or both. N ▪ CAD models used in AM are sliced and stacked to form the desired part. SAT (or ACIS), DXF, STP, and STL are the most common 3D CAD model formats. ▪ STL, which stands for "stereolithography," is the most common AM format. EL ▪ This file format specifies ASCII or binary representations of 2D and 3D object surface geometry, but not color or texture. ▪ PT The STL file contains triangular facets identified by a unit normal and three vertices (corners) using a three- dimensional Cartesian coordinate system. N ▪ Two non-standard variations of the binary STL file can be used to include color information. ▪ As geometry changes, triangle facet density changes. STL files EL can't represent colors, textures, materials, and other printed object properties. ▪ This standard is known as Additive Manufacturing File Format PT (AMF) and it is an XML-based format. Any CAD/CAM software to describe the geometry, composition, color, materials, and lattices of any object to be fabricated with AM technologies can use it N EL PT N Typical STL file. E. Toyserkani, A. Khajepour, and S. Corbin, Laser cladding, 1st edition, CRC Press, 2004. https://doi.org/10.1201/9781420039177. ▪ There are many AM software solutions available. The software EL can be categorized by purpose. Software is used for 3D printing, scanning, improving part performance, and process simulation. ▪ ▪ PT Powermill, NX Cam, and Camufacturing/Master-cam are used. The PowerMiLL CAM solution programs toolpaths for 3- and 5- axis CNC machines, produces smooth surface finishes, and N simulates toolpaths. ▪ Siemens' NX Cam is a high-end CAD/CAM/CAE design, engineering analysis, and manufacturing solution. Robotics software is plentiful. ▪ PoweMill introduced "PowerMill Additive" It's a plugin for DED- EL driven additive or hybrid manufacturing. ▪ It uses PowerMill's multi-axis CNC and robot capabilities to create additive toolpaths with detailed process parameter ▪ control. PT These process parameters enable you to finely control the operations of deposition at a toolpath point level”. N ▪ Despite packages, AM's software hierarchy has major flaws. Lack of intelligence and process-based recipes is a problem. EL 3D CAD model Geometrical information STL model Point cloud data Topological information Functional requirements PT Refinement and Optimization of meshes/facets Material feature database N Design requirements Material information Colored HEO model Heterogeneous Object Geometrical information Model Topological information Data processing Subsystem EL From Model Support Model modelling orientation generation slicing subsystem PT N To control and Process Process Process file parameter Mechanical simulation generation Design TESSELLATION IN CAD MODEL EL PT N https://additivemanufacturingindia.blogspot.com/2018/04/cad-conversion-and-stl-file-in-additive.html GAPS OR MISSING FACETS: EL ▪ During tessellation of surfaces having large curvatures, gaps or holes can be generated at the intersection of these surfaces. ▪ This results in errors at these intersections which leave gaps PT N and holes along the edges as shown in fig. Gaps or missing facets E. Toyserkani, A. Khajepour, and S. Corbin, Laser cladding, 1st edition, CRC Press, 2004. https://doi.org/10.1201/9781420039177. DEGENERATE FACETS: EL ▪ During tessellation, open spaces are created due to unequal tessellation of two adjacent surface patches along a common mating curve. ▪ The stitching of these open spaces is carried by STL file correction software's resulting in geometrical PT N degeneracies. ▪ This is particularly when all edges of the facet are collinear, but vertices are distinctly shown in fig. Degenerate facets. E. Toyserkani, A. Khajepour, and S. Corbin, Laser cladding, 1st edition, CRC Press, 2004. https://doi.org/10.1201/9781420039177. ▪ Degenerate facets provide essential topological information (indicating mating of two surfaces). EL ▪ Even though it does not contain valid surface normal. ▪ Thisvital information is stored before neglecting the degenerate facet. PT N OVERLAPPING FACETS: General ASCII format for defining a facet EL ▪ In STL file Vertices are denoted as floating numbers resulting in a Solid name roundoff error. PT Facet normal ni nj nk Outer loop Vertex v1 v1 v1 Vertex v2 v2 v2 N Vertex v3 v3 v3 end loop endfacet endsolid name N PT EL NON-MANIFOLD GEOMETRIES: EL ▪ Non-manifold geometries are geometric topology terms that allow all disjoint lumps to existing in their own logical body. It can be simply understood with manufacturability. ▪ These are mainly generated due to a round of errors during the PT tessellation of thin features. N EL Name Information included Each file must contain at least one object element to describe the object PT Define single or multiple material IDs for printing Define single or multiple texture mapping N Define displacement parameters, rotation parameters, which are collections of instances Specify additional information about the objects and elements contained in the file FILE FORMAT STL format OBJ format PLY format EL Development 3D Systems Wavefront Stanford Graphics company Technologies Lab Description A face that supports PT Triangular color value Straight lines, Vertices, patch, vertex polygons, surfaces, related free-form curves groups faces, data, N more than three No Yes No vertices The compression ratio of PNG pictures 44.7:1 32.2:1 87.9:1 TECHNOLOGY INDEPENDENCE: EL ▪ AMF files use XML structure to describe the information of model objects in a general way. ▪ It can be not only processed by computers but also understandable by humans ▪ PT The new standard not only records a single material, but also assigns different materials to different parts, and can change the proportion of the two materials in stages. N ▪ It is possible to specify an image to be printed on the surface of the model, and it is also possible to specify the most efficient print path for 3d printing ▪ Raw data such as the author’s name, model name, etc. Can be recorded. SIMPLICITY: EL ▪ For ease of understanding and application, the file format should be able to read and edit in a simple text viewer, and the same information should not be stored in multiple places in the file. ▪ EXTENSIBILITY: PT ▪ As the complexity and size of components increase, it can be expanded with the increasing resolution and accuracy of manufacturing equipment, including large arrays capable of N handling the same objects, complex repetitive internal features (for example, a grid), a smooth surface with fine print resolution, and many components for printing that are placed in the ideal fill material package HIGH PERFORMANCE: EL ▪ It allocates reasonable duration (interaction time) for reading and writing and uses reasonable file size for typical large volume objects. PT STRONG COMPATIBILITY: ▪ AMF format is not only backward compatible, but also compatible with existing STL and PLY file formats and can be adapted to future N developmental needs to achieve forward compatibility. File format STL OBJ PLY AMF 3MF EL Geometric Straight Triangular information, Geometric lines, Fixed points, Description patch colors, materials, information, polygons, facets, author colors, surfaces, related data, materials, PT information, etc., free-form groups can be textures, etc. curves. expanded. Whether it contains No No Yes Yes Yes N color information Whether it contains No No No Yes Yes material information 3D Company 3D Wavefront Stanford ASTM committee manufacturin g ▪ The processing of geometric data for additive manufacturing mainly compromises two stages. EL 1. Slicing the information into layers for path generation. 2. Generating process-specific rastering patterns for each layer. PT ▪ There are a number of slicing methodologies that are being used by various software. These can be basically classified into N two generic methodologies 1. Uniform life 2. Adaptive slicing Input Model Uniform Slicing Adaptive Slicing EL PT N ▪ COMMON ISSUES: EL ▪ Feature resolution ▪ Surface finish ▪ Geometrical accuracy. ▪ ‘STAIRCASE EFFECT’ in angled features. PT N (a) Vertical Features (b) Angled Features (c) Angled Feature – Material extrusion (d) Angled Feature – Material Stereolithography (e) Angled Feature – Powder Bed Fusion ▪ Consider a planner volume. The stair stepping volume is the sum of EL each stair step (Vstep) which is a function of the surface area and Inclined Surface 1 inclination angle that the surface N PT makes with the build plane. 𝐴𝑖 𝐿 cos(𝜃) θ Inclined Surface 2 N step= 2 L cosθ Where, Ai, L, and θ are planners surface area, layer thickness, and angle between normal vector of plane and building directional vector respectively. ▪ If only one feature is present in the component it is preferred to have EL θ=0. Inclined Surface 1 ▪ If more number of features are N PT present it is the designer's responsibility to select between features and decide the optimal orientation. θ Inclined Surface 2 N L cosθ ▪ LAYER HEIGHT: EL ▪ Simplest parameter to influence printed part. ▪ With a decrease in height, finer details with high accuracy and surface finish can be printed. ▪ Most 3d printers have default layer height. (a) An ideal sphere PT N (b) Sphere sliced at 0.8 mm layer height (c) Sphere sliced at 0.25 mm layer height Amza, C.G., Zapciu, A. and Popescu, D., 2017. Paste extruder—hardware add-on for desktop 3D printers. Technologies, 5(3), p.50. EL Thermal stress resisted by PT Build plate (no contraction) Explained layer placed N On stressed expanded layer Additional layers add more Stress but it is uniformly distributed (no wrapping) http://3dp-engineering.com/why-3d-prints-warp/ EL Heating Cooling PT N Solution: By controlling the in-situ surface temperature of the material. http://3dp-engineering.com/why-3d-prints-warp/ SHRINKAGE AND WARPING EL ▪ Associated with Temperature and curing. ▪ Temperature- thermal stresses and residual stresses cause shrinkage and warping. warping occur. PT ▪ Curing- As the layer of photopolymers is cured shrinkage and ▪ Mitigating shrinkage and warping- Printing in a temperature- controlled build environment, avoiding large flat surfaces, and N providing supports. ▪ FILLETS: EL ▪ Helps to reduce stress concentration at corners or edges. ▪ It also helps in assisting the removal of parts from the built platform. ▪ Should be included in 3D design of printed parts wherever possible. PT ▪ Most technology produces “natural fillet” on edges and corners. ▪ SUPPORT STRUCTURES. N ▪ Need for support structures as each layer requires a build platform. ▪ Most support structures have a negative effect on the printed part. ▪ Some process (like FFF and material jetting) offer dissolvable support while some process (like Powder or Sand based) doesn’t require support. EL PT N Fillet compared with a chamfer at Support generation for a bolt to be printed. edges in contact with the built plate 1. Digimat-RP EL 2. RP-Photonics 3. AdditiveLab 4. 5. 6. Procast Arena Automode PT N 7. SimProcess 1. Checking the geometry input as per standard file format. Checking for file errors and rectifying the errors, if any. EL 2. Checking part orientation considering build time, support requirements, stair stepping error, etc. 3. Addition of support structures in automatic/semi-automatic 4. PT mode by providing the support type, criteria for support generation, type of support, etc. as input. Nesting of the same or multiple components as per the requirements. N 5. Slicing the part and support geometry by providing layer thickness as input. 6. Generation of tool path and selection of scanning strategies. 7. Input the process parameters specific to the process. Generally, separate process parameters are provided for the support structure to allow easy removal. 8. Rapidly review the geometry and laser tool path slice by slice. ▪ The qualityof the additive manufactured products also depends on various parameters which are not part of pre- EL processing. ▪ These parameters are machine, material, and application- desired results. PT specific, and their due consideration is required to achieve ▪ The machine setup primary includes the following N 1. Build plate preparation 2. Feedstock preparation 3. Built environment preparation 1. BUILD PLATE PREPARATION: EL It is the base for fabricating the components and the preparation of the build plate is important to obtain high-quality components. some important considerations during build plate preparation are ▪ Selection of build plate material ▪ Preparation of build plate PT N ▪ Levelling of the build plate. https://manufactur3dmag.com/a-movable-3d-printing-build-platform-to-reduce-waste-and-save-time/ 2. FEEDSTOCK PREPARATION : EL ▪ Many feedstock materials tend to absorb moisture from the atmosphere. ▪ During handling, inappropriate storage, and manual sieving/recycling moisture, there are chances to pick up moisture. PT ▪ These moisture pickup can result in poor oxide formation in the additive manufacturing build component. N ▪ The moisture also affects the fluidity of the powder and yields agglomeration and nonuniform spreading during additive manufacturing. ▪ Preheating of the powder is required to remove the moisture. 3. BUILD ENVIRONMENT ▪ The component is generally a built-in controlled atmosphere to prevent oxidation of the melt pool. EL ▪ Argon is generally used for highly sensitive materials like aluminum and titanium while nitrogen is used for processing other metals and alloys. PT ▪ In the case of polymer-based additive manufacturing the melting environment provides uniform temperature to the build component during the process N EL PT N Guo, J., Zhou, Y., Liu, C., Wu, Q., Chen, X. and Lu, J., 2016. Wire arc additive manufacturing of AZ31 magnesium alloy: Grain refinement by adjusting pulse frequency. Materials, 9(10), p.823. ▪ Different file formats used for AM. EL ▪ Errors associated with STL file format. ▪ Slicing and Design consideration for modeling PT ▪ Steps for file preparation for printing ▪ Machine set-up N ▪ Make a flow chart for preparing CAD files for printing. EL ▪ Think and list some of the errors that can occur while machine set up. PT N N PT EL EL PT N Dr. J. Ramkumar Professor Department of Mechanical Engineering and Design IIT Kanpur 1. Powder Bed Fusion (PBF) EL 2. Directed Energy Deposition (DED) 3. Binder Jetting (BJ) 4. i. PT Emerging Metal AM Processes Material Extrusion ii. Material Jetting iii. Sheet Lamination N ▪ Gain an understanding of various commercial AM machines and technology developments EL ▪ Understand the major process parameters for main metal AM processes and parameter linkages PT ▪ Learn about metal AM alloys N EL PT N ▪ A moving heat source selectively sinters or melts powder particles as it scans a specified area matching the build layer to construct complex parts layer-by-layer. Layer-by-layer powder distribution uses hoppers and recoaters. Moylan, Shawn & Whitenton, Eric & Lane, Brandon & Slotwinski, John. (2014). Infrared Thermography for Laser-Based Powder Bed Fusion Additive Manufacturing Processes. AIP Conference Proceedings. 1581. 10.1063/1.4864956. ▪ A roller or recoater blade is used to level powder particles in the build compartment. EL ▪ After one layer, the build compartment is lowered a few hundred microns and a fresh layer is deposited. PT ▪ Next, particles are fused to the previous layer. ▪ Process continues until the part is finished. ▪ The part cools in the build chamber. N ▪ This method prevents geometric distortions. ▪ After cooling, the leftover powder is sucked off the substrate and/or support structures and portions are cut. ▪ Lasers and electron beams are typical heat sources. ▪ When a laser beam is employed, the procedure is called laser PBF (LPBF) ▪ When an electron beam is employed, the procedure is called EL electron beam PBF (EB-PBF or EPBF). PT N Schematic of (a) LPBF and (b) EB-PBF Toyserkani, Ehsan, et al. "Metal additive manufacturing." (2021). ▪ Different nomenclatures have been used to describe different commercial/technical variations of PBF technology, including EL selective laser sintering (SLS), selective laser melting (SLM), direct metal laser sintering (DMLS), etc. PT ▪ SLS sinters particles at high temperatures in solid-state or through diffusion by partial melting. ▪ In SLM lasers induce full melting and coalescence of powder particles followed by solidification. N EL PT N A view of melt pool and ejected spatters in LPBF Toyserkani, Ehsan, et al. "Metal additive manufacturing." (2021). ▪ Cantilever constructions need support to prevent warping from residual tension. ▪ With optimal process settings, support-less printing with PBF is an emerging trend. EL ▪ VELO3D is a startup company that offers support-less printing with optimized process recipes. lightweight structures PT ▪ Topology optimization helps designers create complicated, with great mechanical qualities N Toyserkani, Ehsan, et al. "Metal additive manufacturing." (2021). ▪ Post-processing may include machining, polishing, coating, or hot isostatic pressing (HIP) to reduce manufacturing faults. EL PT N Fig: The CT scan results of (i) cylindrical, (ii) triangular prism, and (iii) magnified edge of a cuboid sample made using EB-PBF from Ti6Al4V (a) before HIP treatment and (b) after HIP treatment. (c) The effect of the micro-machining process (MMP) on the surface properties of a PBF-made part Toyserkani, Ehsan, et al. "Metal additive manufacturing." (2021). EL PT N High precision tool with complex embossing of the inner surface made from Aluminum (left) and Lightweight titanium hip implant (right). Toyserkani, Ehsan, et al. "Metal additive manufacturing." (2021). ▪ PBF can manufacture high-resolution parts (>30 m), making it suited for organic designs with thin walls, fine details, internal EL channels, etc. DED systems have >250 m resolution. ▪ Due to bigger particle sizes and concentrated beam spot sizes, PT EB-PBF has a coarser resolution than L-PBF (>60 m). ▪ EB-PBF printed items also have increased powder agglomeration. PBF is a mid-speed procedure. Laser scanners can reach 5 m/s, whereas EBs can reach 10 km/s. N ▪ Long heat-up/cool-down cycles before and after printing also slow production. These are major challenges. ▪ Increasing the number of lasers and enhancing beam scanning technologies are AM industry options for production speed. ▪ GE Additive (Lichtenfels, Germany) has created modularized systems with removable building chambers to reduce machine EL idle time. ▪ In such systems, cool-down, powder resupply, powder removal, PT etc. are done offline and don't affect production. ▪ PBF is currently limited to 800 mm (width and length) and 500 mm (height), rendering it unsuitable for generating bigger functional pieces in one piece. For R&D or jewelry production, N manufacturers provide smaller build chambers. ▪ In the future, PBF systems with bigger building envelopes are expected ▪ More than 100 process parameters regulate PBF printing. Many may be insignificant. Over 20 criteria affect printed part EL quality. Understanding how to combine these parameters produces prints with the best part qualities. PT ▪ Process optimization is currently manual and relies heavily on designer and operator discretion. ▪ Machine developers provide instructions and optimum recipes for their proprietary materials. N ▪ Extending those to new alloys requires trial and error or statistical experiments. This is a costly and time-consuming process. This will EL remain a fundamental limitation for all AM classes, including PBF unless data sets relating to process conditions and part attributes are created. Once developed, PBF can be widely used in the industry. PT ▪ Manufacturers are developing smarter AM machines with sensors and closed-loop quality control algorithms. AI-based strategies may solve this issue in the near future by improving process control and data collection at different manufacturing phases. N ▪ Current efforts for developing more intelligent systems with real- time monitoring/control are also important for improving repeatability and reliability of production, an important step in the industrialization of AM, especially for critical applications where high quality is required and for part certification where the procedure is scrutinized. ▪ PBF can process alloys, ceramics, and composites EL ▪ Due to the inert printing situation, it can also fabricate highly reactive materials and high entropy alloys. ▪ Various research groups have studied and optimized many PT alloys for LPBF and EB-PBF, however, the number of materials treated by these techniques is few. ▪ Due to similarities between the two processes, any weldable N alloy, especially highly reactive ones, can be used in PBF. ▪ However, certain chemistry, morphology, and particle size distribution criteria must be met. ▪ Because EB-PBF runs under full vacuum, it has less reactive material concerns than LPBF. EL ▪ PBF uses very spherically, uniform-sized particles. ▪ With low-energy-efficient atomization processes dominating minimized. PT powder feedstock production, high raw material costs must be ▪ Recent AM research includes water-atomized low-cost alloys N for PBF. This trend adds new alloys to PBF's material inventory. ▪ Current PBFs only support one material. Multi-material parts require advanced powder delivery methods, which aren't commercially available until 2020. ▪ If tuned, PBF can produce near full-density (99.99% for Ti-6Al- 4V) parts for industrial applications. EL ▪ Printed parts can be heat treated to remove build faults such as anisotropy, partial fusion, and contaminants. PT ▪ Parts created with PBF have a different microstructure than their conventionally made counterparts from a similar alloy due to their differing thermal history. This produces mechanical differences between PBF and wrought parts. N ▪ PBF products sometimes have superior mechanical properties due to finer microstructures than cast components and properties near to wrought parts. ▪ Porosity in PBF sections reduces fatigue resistance. EL ▪ PBF-made parts have a moderate surface smoothness with a roughness of tens to a few hundred microns depending on powder quality, part orientation, process core parameters, skin parameters, etc. PT ▪ PBF manufacturing is often followed by surface treatments like polishing, sandblasting, coloring, coating, etc., which increase production time and expense. N ▪ DED is a metal AM class based on laser and electron beam welding, material feeding/injection, and CNC. EL ▪ DED melts deposited/fed materials to metallurgically attach them to the substrate ▪ In PT this process, the raw material (powder or wire) is deposited/fed into a melt pool produced at the incident point of the energy source and materials. N ▪ The filler melts in the pool and solidifies as the energy source passes, simulating PBF. ▪ By articulating the nozzle or substrate (or both), the filler material's confocal point follows a predefined 3D path to EL deposit neighboring tracks. ▪ The nozzle or substrate moves by one layer's thickness between layers. PT ▪ A thick 3D geometry with little porosity is created. ▪ Inert chambers are used for fabrication. N ▪ DED is mechanically superior to PBF. a) Powder-fed laser DED with lateral EL nozzle b) Powder-fed laser c) DED with a co-axial nozzle Wire-fed EB-DED. PT N E. Toyserkani, A. Khajepour, and S. Corbin, Laser cladding, CRC Press, 2004. W. Y. Y. S.L. Sing, C.F. Tey, J.H.K. Tan, and S. Huang, Rapid prototyping of biomaterials, Elsevier Books, 2020. ▪ HEAT SOURCE EL ▪ Laser ▪ Electron beam ▪ Electrical arc (EA) PT ▪ Plasma transferred arc (PTA) ▪ FEEDSTOCK FEEDING MECHANISM ▪ Powder injection N ▪ Wire feeding ▪ Paste feeding through extrusion ▪ APPLICATION EL a) Production of near-net- shape structures that may need post-machining. b) Freeform fabrication of selective features on pre- build structures c) Repair components. of high-value PT N E. Toyserkani, A. Khajepour, and S. Corbin, Laser cladding, CRC Press, 2004. a) Broken gear teeth EL b) After LENS printed repair c) Machined to specification a) PT b) c) N T. Cobbs, L. Brewer, and J. L. Crandall, “How 3D metal printing saves time and lowers costs: DED for repair of industrial components,” OPTOMEC, pp. 1–38. ▪ Resolution varies widely among DED sub-categories, from 0.25 mm for LDED to a few millimeters for EB-DED and PTA-DED. ▪ This method may not be able to deposit fine features, internal EL channels, and intricate shapes. ▪ Complexity constraints may apply to arc-based designs. ▪ The high cost of filling a powder bed with pricey specialty PT powders and the technical requirements of controlling articulation and temperature limit PBF build size. ▪ Since the powder is deposited by a nozzle in DED, the created environment is larger. N ▪ This property is desirable in tooling, automotive, and aerospace. ▪ DED's robotic systems enable infinite build areas. If a Cartesian robot is employed, the building envelope may be 30 m X10 m. ▪ DED systems have the highest deposition rates among AM classes. EL ▪ Depending on the energy source, powder- and wire-based DED systems can deposit 500–4000 cm3/h. In PBF, it's 5–20 cm3/h. PT ▪ Consumers or manufacturers must know each technology's strengths to choose the best solution for their application. ▪ DED's excellent printing speed and resolution can be used for N low-complexity parts and repairs. ▪ DED has dozens of process parameters. Controlling the nozzle and/or baseplate motions adds to the difficulty of this EL procedure. ▪ The construction portion is visible in DED, and open- architecture DED systems make it easier to integrate PT metrology/control systems. ▪ In settings where the energy source is in the powder delivery nozzle, beam scanning is slower and less accurate than utilizing galvanometers or an electromagnetic system in PBF. N ▪ This causes printing faults like over-deposition at corners (similar to overfilling in extrusion systems), where the nozzle speed is reaching zero or trying to reach the stable printing speed, but the powder delivery volume is fixed. ▪ Addressing such faults during the print needs high-end motion systems, sophisticated powder delivery apparatus, and EL integrated closed-loop control systems. ▪ The powder-based DED's low catchment efficiency wastes material and is hard to fix. PT ▪ R&D centers are developing innovative nozzles and auxiliaries to increase catchment. ▪ Large-scale wire-feeding mechanisms can be difficult. N ▪ For a successful print, various elements affecting filler delivery must be optimized. ▪ Similar to PBF, future generations of DED systems are getting smarter via sensors and closed-loop control systems. ▪ DED uses powder for laser and arc-based devices and wire for all others. EL ▪ DED has more materials than PBF. EB-DED can handle reactive metals in a vacuum. PT ▪ In-situ material grading is another advantage of DED. ▪ Adding a secondary powder hopper or wire coil feeder makes adding a second material easier than PBF. N ▪ Powder delivery employing nozzles has less rigorous morphology, particle size distribution, etc. criteria than powder EL bed, lowering DED material costs. ▪ To broaden this technology's industrial uses, cost-effective PT supplies of DED powder similar to PBF are needed. ▪ Wires are widely available for many alloys, making wire-based DED a suitable method, where reduced geometrical accuracy or 100% density is required. N ▪ Uniform beads require high-quality, consistent-diameter wires. ▪ DED systems have lower dimensional accuracy and surface finish than PBF, however, this may not be an issue since the parts will be EL finished in post-processing. ▪ Large melt pools produce residual stress and distortion that require HIP and other thermal treatments. ▪ ▪ PT Powder-based DED parts have little porosity, while wire-based DED parts are dense even before heat treatments. L-DED has quick cooling rates like PBF, resulting in fine microstructure and enhanced strength. N ▪ EB- and PTA-DED have bigger microstructures due to slower cooling. ▪ This is especially important in arc technologies, where heat buildup during the operation contributes to a prolonged cool- down cycle and residual strains. ▪ BJ involves three steps: green part manufacture, drying/debinding, and sintering/infiltration. EL ▪ First, like PBF, a powder layer is deposited. The binder printhead runs over the powder bed and distributes minute PT droplets of a polymer-based binder to bond the particles. ▪ After a layer, the powder bed is lowered by one layer and recoated. N ▪ Continue till the part is finished. EL PT N 3DHubs, “Material Extrusion.” [Online]. Available: 3dhubs.com ▪ BJ machines can regulate the build chamber temperature, which can be used to partially dry printed items, to prevent slippage EL when depositing a new layer. ▪ The parts stay in the machine until the binder cures. ▪ Since the binder has filled the powder's pores, the product is PT virtually entirely dense at this stage. ▪ Next, the green portions are removed from the powder bed, cleaned to remove excess powder, and sent to a furnace to N evaporate the binder followed by partial particle sintering (debinding). This stage produces brittle, porous green portions. ▪ Either the sintering process is extended to close pores and increase mechanical qualities, or the green section is infiltrated with bronze or copper to fill internal voids. ▪ First option shrinks pieces, which should be considered during design. EL ▪ The second alternative shrinks less and can be utilized to make a two-material part with tailored qualities. PT ▪ BJ-made parts can be beyond 90% dense, although PBF and DED items are difficult to make with this method. ▪ HIP reduces porosity. BJ is a good production process for N implants or structural pieces that require porosity. ▪ Porosity can be adjusted by adjusting process factors like powder bed density or binder saturation. ▪ This AM technique resembles PBF and MJ. It's similar to PBF, which layers powder. EL ▪ Powder particles aren't fused with heat, unlike PBF. They're bound utilizing a binding liquid. This is economically beneficial PT because lasers and electron beams are expensive and require severe safety procedures. ▪ The binder is dispensed using a printhead similar to the MJ technique, although it only makes up a portion of the part, N which is evaporated during post-processing. ▪ BJ works similarly to home inkjet printers but glues powder particles together instead of spraying ink on paper. EL ▪ This technology offers fast printing, and the ability to stack parts for efficiency, scalability, and printing composites. ▪ BJ PT is used for visual prototypes, low-/medium-quantity functional parts, and big parts with conformal cooling channels for casting and tooling. N EL PT N Binder jetting technology in Action Toyserkani, Ehsan, et al. "Metal additive manufacturing." (2021). ▪ Multi-Jet Fusion EL ▪ In this method, which was created for nonmetal powders and later used to metal systems in HP's new line of machines (Metal Jet), two binders are placed on each layer: the fusing agent and detailing agent. ▪ ▪ PT Fusing agent is deposited where particles must fuse to produce the part’s cross-section. Detailing agent is put around fusing agent to define cross-section borders. N ▪ The build platform is then heated. ▪ It selectively fuses fusing agent-coated particles while leaving detailing agent-coated parts unfused to generate finer details and sharper edges than normal BJ procedures and control binder leakage outside the specified part shape. ▪ Multi-Jet Fusion technique steps EL PT N “Desktop Metal.” [Online]. Available: https://www.desktopmetal.com/products/studio EL PT N Sample part made using BJ technology 3DHubs, “Material Extrusion.” [Online]. Available: 3dhubs.com. ▪ BJ's great vertical resolution is attributed to tiny powder layers. This technology's lateral resolution depends on the droplet EL size, quality, and uniformity on one side and their wetting properties on the other. layer thickness. PT ▪ After sintering, the resolution of BJ procedures depends on the ▪ Antistrophic correction factors applied to the original CAD model can compensate for sintering shrinkage. N ▪ As green components are not sturdy, printing parts with fine details like cellular and architectural structure may not be possible as they may shatter when being transferred to the furnace for curing. So, design freedom is limited. ▪ BJ's pieces are confined by a powder bed, therefore there's no need for support structures to transfer heat to the baseplate. EL ▪ To improve productivity, pieces can be stacked as high as build dimensions and material availability. PT ▪ BJ is a high-speed AM process because, unlike PBF and DED, it doesn't follow a raster path to fuse particles into parallel lines until the part’s cross-section is completed. Instead, nozzle arrays bind particles linearly. N ▪ One or a few passes of a printhead generate each layer's silhouette. BJ is the best technology for economically effective process scaling up by increasing the number of arrays of nozzles or printheads. ▪ BJ is a complex AM system where numerous factors affect part quality. EL ▪ Even though no beam/optics parameters are involved, additional parameters need to be optimized to ensure appropriate bonding, acquire goal porosity levels, and maintain geometrical correctness. ▪ ▪ PT Even with adjusted process parameters, test layers are frequently needed to stabilize printing. BJ fabrication difficulties include nozzle clogging and poor binder dispensing. N ▪ Commercial BJ systems commonly include nozzle cleaning/printhead health checking stations with automatic cleanings. ▪ Part shrinkage is an inherent feature of this technique. Evaluate the shrinkage rate during design to ensure the final product fulfills dimensional requirements. ▪ Identifying and constructing master sinter curves increases process complexity and initial setup time. ▪ Most BJ materials are ceramics and polymers, and commercial metallic materials are restricted compared to PBF and DED. EL ▪ Binders can treat metals that cannot be thermally processed. As a powder bed-based technique, BJ may only use one type of powder. PT ▪ Dispensing a binder with specified qualities like electrical conductivity, color, elasticity, etc., or infiltrating components with lower melting points than the underlying powder material can create functionally graded structures. N ▪ ExOne offers an annealed 420 stainless steel matrix infiltrated with bronze at a 60:40 ratio, which delivers exceptional mechanical performance (ultimate strength of 682 MPa and elastic modulus of 147 GPa), machinability, weldability, and wear resistance. ▪ BJ-increased AM's porosity causes stress concentration, cracking, and fatigue failure in BJ-made parts compared to PBF EL and DED. ▪ BJ's surface roughness is similar to PBF's, but it's less accurate. PT ▪ HP's metal jet technology is a recent trial to improve BJ printing sharp corners and well-defined edges. ▪ Desktop Metal (Burlington, MA, USA) employs printheads that can deposit droplets as small as 1 pL to increase dimensional N accuracy and surface polish. ▪ Since BJ components aren't formed by fusing particles with heat, anisotropic characteristics don't induce property non- uniformity in the x–y direction. ▪ Weak vertical (print) mechanical qualities remain a problem. Comparison of traditional binder jetting and Desktop Metal’s binder jetting with controllable droplet volume to avoid EL under/overprinting PT N “Desktop Metal.” [Online]. Available: https://www.desktopmetal.com/products/studio. ▪ ME is a class of AM technologies that dispenses a fluidic or semi-fluidic material through a nozzle to build each layer of a EL part. ▪ ME approaches range from a single syringe attached to an XYZ ▪ It PT gantry system distributing ingredients using compressed gas back pressure to bioprinters. is comprised of complex dispensing units in an environmentally controlled chamber to systems fed with N thermoplastic polymer filaments. ▪ Fused deposition modeling (FDM) is utilized by hobbyists for prototypes and industries for high-end products. ▪ FDM is the preferred ME technology for metal parts. EL PT N Schematic of material extrusion system 3DHubs, “Material Extrusion.” [Online]. Available: 3dhubs.com. ▪ Some companies have developed FDM systems capable of processing metals to benefit this process. EL ▪ Metal FDM filaments are a metal-polymer combination. Without loose powder, handling is safer. PT ▪ After printing, the part goes through debinding and sintering steps similar to BJ technique to remove the binding polymer and become entirely metal. N Metal filament “Desktop Metal.” [Online]. Available: https://www.desktopmetal.com/products/studio EL PT N Visual prototypes made using (a) 17-4 PH stainless steel on Markforged ME system by Shukia Medical (b) Desktop Metal’s Studio System “Desktop Metal.” [Online]. Available: https://www.desktopmetal.com/products/studio ▪ In MJ technology, fluid droplets are deposited on a substrate to make a component. MJ's AM is comparable to BJ’s. EL ▪ The material needed to make a part is deposited from a printhead, and the part is created in an empty space rather than a powder bed. PT ▪ This method is cheaper than AM systems using lasers and electron beams. N ▪ MJ is a non-contact method that fires droplets at the substrate from a distance, unlike ME. Adding printheads makes the technique scalable and affordable. ▪ This technology can create programmable voxel-level digital materials. EL PT N Schematic of material jetting technology. 3DHubs, “Material Extrusion.” [Online]. Available: 3dhubs.com. ▪ The print material is transferred from a container to the printhead. EL ▪ If the target material isn't already liquid, it must be melted or dissolved/suspended in a fluidic medium. produce a droplet. PT ▪ Backpressure then ejects material from the nozzle aperture to ▪ A heater attached to the fluid reservoir evaporates adjacent material to generate a bubble. N ▪ In piezoelectric jetting, the expansion/contraction of a piezoelectric ceramic driven by an electrical current deforms the fluid reservoir and ejects droplets. ▪ MJ printheads deposit material quickly with hundreds to thousands of nozzles. ▪ Drop-on-demand (DOD) printing releases droplets as needed. EL ▪ The droplets agglomerate on the substrate and solidify through cooling, solvent evaporation, chemical reaction, or photopolymerization. Continue until a component is complete. PT ▪ A solitary printhead prints sacrificial support materials. ▪ Academic research organizations have developed low-cost MJ systems. This system's straightforward assembly contributes to N its widespread use in R&D. ▪ These organizations have tested, optimized, and described MJ polymers, ceramics, composites, functional inks, and metals. ▪ The print material is transferred from a container to the printhead. EL ▪ Stratasys and 3D Systems provide high-end MJ systems that can print colored items. PT ▪ XJET (Rehovot, Israel) is the first commercial MJ metals firm. ▪ In their Nanoparticle Jetting technique, inks made of suspended metal nanoparticles in a liquid media are jetted onto a substrate with support material. N ▪ Each printer pass forms the part, which is subsequently support-removed and sintered to provide the necessary mechanical characteristics. ▪ After sintering, the technology reportedly dispenses tiny layers and detailed features with 99.9% density. EL PT N XJET’s Nanoparticle Jetting technique is one of the emerging metal AM technologies. “XJet.” [Online]. Available: www.xjet3d.com. EL PT N Sample parts made using XJET system “XJet.” [Online]. Available: www.xjet3d.com. ▪ SL binds thin sheets of basic materials in AM. EL ▪ Before solid-state joining, these sheets can be trimmed to reflect a layer’s cross-section, or extra materials can be eliminated after the sheets are linked to generate a particular height. PT ▪ Ultrasonic consolidation or brazing binds metal SL. ▪ In this process, two metal sheets are stacked and an ultrasonic tool travels over them to remove surface oxide through high N friction. ▪ Once the surfaces are oxidation-free, two sheets bind. ▪ Repeat until the near-net-shape section is generated. ▪ The final geometry is created by machining away surplus material. ▪ This process is done at temperatures considerably below the melting point of the build material; therefore, it doesn't change EL the microstructure or mechanical properties of the raw material. PT ▪ This method can combine dissimilar metals more efficiently than fusion, which creates a weak transition zone between the alloys. ▪ It can be used to make FGMs and sensors. N ▪ Few ultrasonic consolidation-compatible material pairs. Al alloys can combine to Be, Cu, Au, Mg, Mo, Ni, Ti, Si, Ta, etc. EL PT N Ultrasonic consolidation mechanism Toyserkani, Ehsan, et al. "Metal additive manufacturing." (2021). EL PT N FGM parts are made using Fabrisonic’s ultrasonic consolidation system (a) and (b) parts composed of two materials, (c) smart structures with an embedded thermocouple. “Fabrisonic.” [Online]. Available: https://fabrisonic.com. ▪ Process, material, advantages, and disadvantages of EL 1. Powder Bed Fusion (PBF) 2. Directed Energy Deposition (DED) 3. PT Binder Jetting (BJ) N 4. Emerging Metal AM Processes i. Material Extrusion ii. Material Jetting iii. Sheet Lamination ▪ Google and List application sector of each process discussed in the lecture. EL ▪ Compare discussed AM processes with each other. PT N N PT EL