DM308 Production Techniques 2 Lecture 1 – Additive Manufacturing (Part 2) PDF
Document Details
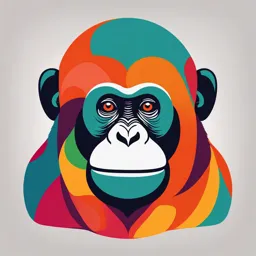
Uploaded by SafeDiscernment
University of Strathclyde
Vassili Vorontsov
Tags
Summary
This document is a lecture on additive manufacturing (AM), specifically focusing on Direct Metal Deposition (DMD) and Material Jetting. It covers feasibility assessments, benchmarking, CAD interfaces, and other aspects of AM.
Full Transcript
DM308 Production Techniques 2 Lecture 1 – Additive Manufacturing (Part 2) Dr. Vassili Vorontsov Acknowledgements: Prof. Jonathan Corney, Mr. Colin Andrews Department of Design, Manufacture and Engineering management, Faculty of Engineering, University of Strathclyde [email protected] To...
DM308 Production Techniques 2 Lecture 1 – Additive Manufacturing (Part 2) Dr. Vassili Vorontsov Acknowledgements: Prof. Jonathan Corney, Mr. Colin Andrews Department of Design, Manufacture and Engineering management, Faculty of Engineering, University of Strathclyde [email protected] Today’s lecture Additive Manufacture (AM) Lecture 2 1) Direct Metal Deposition (DMD) Systems 2) Material Jetting Systems 3) Feasibility assessments: Technical, Economic, Environmental (Energy), Material 4) Benchmarking : Key Parameters and Measures of Performance 5) CAD Interface 6) Herring Bone Diagram for ALM Direct energy deposition Direct energy deposition Direct metal deposition DMD processes directly fabricate metal hardware by injecting the metal powder of choice into the focal point of a powerful laser as it traces the perimeter and fills of a part. Metals currently available include 316 stainless steel, H13 tool steel and Titanium-6-4 (An alloy containing 6 wt.% Aluminium and 4 wt.% Vanadium). Ins Inc uffici en on (wa siste t hea n vy t sur t mel fac t e) Exc ess ive h ea t (p ud dle s) Exc (rip essiv ple e v s in olu sur me fac e) Ins uffi cie (sp nt vo utt l er) ume DMD process window Functionally graded materials with DMD Metallographic cross-sections from the graded transition region between differing titanium aluminide alloys. Any shape, any material, any mix of materials at any point! A few years yet! Material jetting Material jetting Material jetting Material jetting is one of the fastest and most accurate 3D printing technologies. Schematically it looks very similar to “Binder Jet Processes” with printer heads being used to deposit precise droplets of material. But the difference is that the jet delivers the build material (rather than glue). The most common commercial system builds parts using liquid photopolymer droplets, which are cured (made solid) with UV light. In other words it’s a drier form of SLA where the photopolymer resin is sprayed in droplets before it's solidified. Material jetting capabilities ALM feasibility A rapidly changing picture Two questions (FAQs) on ALM? What components make sense (economically and technically) to manufacture on particular additive layer systems? How do I compare such different additive systems? Which parts are technically feasible? Above: ALM Capability established by surveying academic papers, case studies and trade publications. Feasibility case study 1: gate arm Feasibility case study 1: gate arm Feature Material: A240 Chromium Nickel SS Build envelope: 8’’x5.88’’x2.562’’ (203.2x149.352x 65.0748mm) Surface roughness 125 Ra Yes No Maybe Reasons X Mostly all machines X Most machines: 250 x 250 x 250 mm X Most of the reported results are less than 20 Ra Feasibility case study 1: gate arm Feature Hole diameter: 9,25.4,69.85mm Hole accuracy: Ø ±.001’’, +.003’’ (.0254,.0762mm) Yes No Maybe Reasons Should be comfortable with more than 5mm X X Most of the hole error range between -0.2 to -0.4mm Fastener: 9.525mm X But hole of 9mm could be created Positional accuracy: Ø.001’’ X No reported data X X No reported data Parallelism:.002’’ Perpendicularity: Ø.001’’ Curvature radius: 1.12’’,2.94’’, 3.31’’, 4.56’’ Hole depth: 0.31’’,0.62’’ No reported data ? ? No reported data No reported data Technical feasibility: Conclusions ALM unable to deliver engineering levels of precision Consequently coverage almost always less than 100% However consider Hybrid approach ALM followed by CNC Comparison of ALM capability should be made against Near-Net-Shape (NNS) technology forgings, casting or rough machining. This approach makes sense of many of the observed trends in metal ALM (e.g. speed rather than precision) What about economic feasibility? (very few significant academic papers in addressing this) What about economic feasibility? (very few significant academic papers in addressing this) Plastic injection moulding vs SLA HPDC= High Pressure Die Casting SLS = Selective Laser Sintering SLM = Selective Laser Melting What about economic feasibility? The cost of high quality powered metal dominates SLS/SLM costings. What about economic feasibility? Occasionally there are other references in the literature which suggest existence of unpublished comparative studies If the "buy to fly ratio" of a structure is below about 30%, meaning that more than 70% is machined away, additive manufacturing technologies become an alternative to conventional machining for complex metallic parts. This is said in the context of aerospace parts but no source is cited How do you compare different systems? How do you assess or evaluate them? Which one is best? You can compare: Energy Materials Accuracy and speed Environmental feasibility How much energy is required for FDM? The energy need to melt the filament can be estimated by the formula where Q is the amount of energy in Joules, c is the specific heat of the extruded material, m is the mass, and ΔT is the change in temperature. Typical Values ABS filament has a specific heat of 1300J/kg-K (1.3 J/g°K), ABS filament has a density of 1.38 g/cm 3 (0.00138 g/mm3) Assume Filament diameter of 1.75mm Extrusion temperature of 110 oC Therefore minimum theoretical (I.e. baseline) power required for an extrusion rate of 2.2mm/sec at an ambient temperature of 20oC (ignoring friction effects, motor power, bed heating and other losses) can be estimated: Volume of material per second = 3.14x (1.75^2/4)x2.2 = 5.3mm3 M (mass of material per second) = 5.3x 0.00138 = 0.007314g ΔT = 110 – 20 = 90oC c = 1.3J/gK Q = 1.3 x 0.007314 x 90 = 0.86J/s = 0.86W Build takes 1.5hours = 5400 seconds 0.86 x 5400 = 4.6kW ! Energy consumption vs. quality The graphical relationship between energy consumption and print quality/layer resolution/layer height within small scale additive manufacture. 1. makerbot.com 2. efergy.com 3. http://www.strath.ac.uk/dmem/businessandindustry/rapidprototypingandmanufacturingfacilities/ 4. http://wwwusers.york.ac.uk/~mb55/msc/maths/graphstr.htm Energy consumption vs. quality The graphical relationship between energy consumption and print quality/layer resolution/layer height within small scale additive manufacture. Energy Use 0 500 1000 High Quality 1500 Medium Quality 2000 Low Quality 2500 3000 Watts consumed 1. makerbot.com 2. efergy.com 3. http://www.strath.ac.uk/dmem/businessandindustry/rapidprototypingandmanufacturingfacilities/ 4. http://wwwusers.york.ac.uk/~mb55/msc/maths/graphstr.htm How much energy is required for SLM? Volumetric energy density (VED) Where (typical values): Es = Area energy density P = Laser Power (150-2000W) V = Scan speed (5 – 20mm/sec) d = diameter of beam (0.2mm) Es= 150/(5x0.2) = 150J/mm2 How much can be melted (ie layer thickness)? Consider one second at 20oC ambient Volumetric Energy Density(VED) is an important parameter for comparing systems. Typical values c = Specific heat of steel (solid)= 0.49kJ/kg K Steel melts at around 1370oC so ΔT = 1370 – 20 = 1350oC Q = 150J/mm2 So m = 150/(490x1350) = 2.3x10-4kg = 0.226grams Density of steel 7850kg/m3 (0.00785g/mm3) How deep a 1mm3 volume of metal m = ρV => V = 0.226/0.00785 = 0.28mm This is the Layer thickness (t) implies …2.8mm3/sec …compared to plastic 5.3mm3/sec Comparison of ALM material properties Randal Schubert, HRL Laboratories @ 2013 Metallic materials available for ALM Melting vs. sintering (1) In sintering particle bonding is initiated at contact points. (2) Contact points grow into "necks". (3) pores between particles are reduced in size. (4) Grain boundaries develop between particles in place of necked regions. Thus, powder particles are consolidated in the solid state rather than coalescing into a liquid melt pool. Properties depend on microstructure In ALM Microstructure varies with…. So AML parts will be stronger in one direction than another, but this can also be true for other types of metal production methods, such as rolling. Laser paths control microstructure because of the difference in cooling rates This allows the material properties to be controlled by adjusting/tuning the laser paths during SLS/SLM. Metal powder characteristics Engineering powders A powder can be defined as a finely divided particulate solid Geometric features of engineering powders: – Particle size and distribution – Particle shape and internal structure – Surface area ALM Benchmarking Further comparison of ALM methods An Msc student figured it out in 1996: Rapid Prototyping of Solid Three-Dimensional Parts By Sara Anne McMains EECS Department University of California, Berkeley Technical Report No. UCB/CSD-96-892 1996 http://www.eecs.berkeley.edu/Pubs/TechRpts/1996/CS D-96-892.pdf This is an old document but worth reading for background Cost comparison The “Chrysler Part” used as a Standard test component Speed comparison Volume of material added to model per second (independent of geometry). Accuracy comparison Accuracy of machine when it moves to given x-y-z coordinate. z x-y Geometry comparison The smallest hole that can be made. Shape capability comparison Dependent on support materials and how they are removed. Benchmarking ALM technologies Sara’s idea of a standard test components lead to the idea of a standard benchmark part. We’ll focus on the SLS benchmark part Same part is built on a number of different SLS machines to assess their performance. What the SLS benchmark assesses SLS Benchmark Assesses the following… 1. Sloping plane: Surface finish; stair case effect 2. Cylinder 1-4: resolution (smallest cylinder) and accuracy (relative alignment of the stepped cylinders) 3. Thin plane: Warping, strength 4. Rounded Corner: Surface finish on overhanging sections (in the face of support removal) 5. Overhangs: Ease of support, powder or liquid removal. 6. Holes 1-4: Resolution (at what point does the holes become un-manufacturable) 7. Thin Wall 1-3: Strength, and warping 8. Sharp Corners: Resolution, Accuracy 9. Overall Shape: warping 10. Overall Shape & Relative location of features: Dimensional accuracy. Benchmark shape dimensions 0.25L L=? L=? Benchmark dimension should reflect typical build volumes of the systems being compared and the nominal range of accuracies and resolutions. As a general rule the smallest features are about 1% of L and L must be less than max build volume. So if max volume is 250x250x250 say L for benchmark would be 100mm would small features of around 1mm (sufficient to challenge most systems). Results Results 2 Benchmark paper on MyPlace What is additive layer manufacturing? Benchmarks should be built in a number of orientations because... Part Orientation Roughness also effected by process The CAD interface The CAD interface STL Format = The universal language of Manufacturing and Design CAD models translated into STL before building on ALM systems STL file STL was invented by the Albert Consulting Group for 3D Systems (Chuck Hull) in 1987. The format was developed for 3D Systems' first commercial 3D printers. Since its initial release, the format remained relatively unchanged for 22 years. Example.stl file format for a tetrahedron solid tetrahedron facet normal 0 0 0 outer loop vertex 1 1 1 vertex 1 -1 -1 vertex -1 1 -1 endloop endfacet facet normal 0 0 0 outer loop vertex 1 1 1 vertex -1 1 -1 vertex -1 -1 1 endloop endfacet facet normal 0 0 0 outer loop vertex 1 1 1 vertex -1 -1 1 vertex 1 -1 -1 endloop endfacet facet normal 0 0 0 outer loop vertex -1 1 -1 vertex 1 -1 -1 vertex -1 -1 1 endloop endfacet endsolid tetrahedron Mesh density The mesh density determines the quality of the parts. Shape only format: STL STL is a verbose, but precise mesh format. (I.e. it has no gaps between triangles.) For a long time ONLY ONE VERSION. Lowest common denominator …it became used as general CAD exchange format and is used in many applications that have nothing to do with 3D printing. *.STL => *.3MF or *.AMF or ? STL file format is becoming a major barrier to production of objects with high volumetric complexity, such as internal lattices and graded materials – essentially reaching a point where STL files make certain advanced ALM applications difficult or impossible. 3D Manufacturing Format or 3MF is a file format developed and published by the 3MF Consortium. 3MF is an XML-based data format designed for using additive manufacturing, including information about materials, colours, and other information that cannot be represented in the STL format. AMF (Additive Manufacturing File Format) or AMF is an open standard for describing objects for additive manufacturing processes such as 3D printing. The official ISO/ASTM 52915:2013 standard is an XML-based format designed to allow any computer-aided design software to describe the shape and composition of any 3D object to be fabricated on any 3D printer. Unlike its predecessor STL format, AMF has native support for colour, materials, lattices, and constellations. Herringbone diagrams for ALM Factors affecting ALM Q: There are so many potential factors affecting ALM quality how can we record them all? A: The Cause and Effect (CE) Diagram a.k.a. a fishbone diagram a.k.a. “An Ishikawa Diagram” : Created in 1943 by Professor Kaoru Ishikawa of Tokyo University Six categories affecting quality A mind map for process engineers Watch video on fishbone diagrams https://youtu.be/BW4qvULMJjs Ishikawa diagram for SLM Poor Quality Part 8 Major Factor: (1) User , (2) Process preparation, (3) Part geometry, (4) CAD data, (5) Material, (6) LFF System (i.e. the SLM machine), (7) Process, (8) Post-processing You should be able to list the eight main classes of process factors that can lead to poor quality ALM results. SLM = Selective Laser Melting = Laser Freeform Fabrication = LFF Next week Perhaps today’s ALM systems are like the Internet before web browsers were invented (a technology looking for the compelling application). So in the final week we look at trends: Topological and material optimisation Emerging Distributed Manufacturing Paradigms Remanufacturing applications