Mader 17e PPT Ch25 DNA Structure and Gene Expression PDF
Document Details
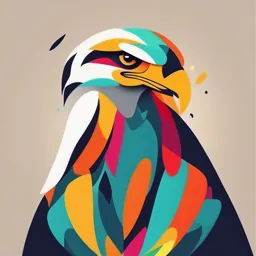
Uploaded by AffluentJungle2903
Alabama State University
Sylvia S. Mader, Michael Windelspecht
Tags
Summary
This PowerPoint presentation details the structure of DNA, including its components, base pairing, and overall double helix structure. It also covers the process of DNA replication, emphasizing the semiconservative nature of the process, and the role of DNA as genetic material.
Full Transcript
Because learning changes everything. ® INQUIRY INTO LIFE...
Because learning changes everything. ® INQUIRY INTO LIFE Seventeenth Edition Sylvia S. Mader Michael Windelspecht Chapter 25 DNA Structure and Gene Expression © McGraw Hill LLC. All rights reserved. No reproduction or distribution without the prior written consent of McGraw Hill LLC. 25.1 DNA Structure 1 In the mid-twentieth century, geneticists were determining that DNA (deoxyribonucleic acid) is the genetic material. Biochemists were determining the structure of DNA. These research efforts led to our knowledge of modern molecular biology. © McGraw Hill LLC 2 25.1 DNA Structure 2 Prior to these efforts, it was known that genetic material had certain qualities. It was able to store information for development, structure, and metabolism of a cell or organism. It was stable, so it could be replicated with high accuracy and transmitted from generation to generation. © McGraw Hill LLC 3 The Nature of the Genetic Material 1 In 1931, discovery of the genetic role of DNA began with research by Frederick Griffith. He worked with Streptococcus pneumoniae, bacteria that cause pneumonia in animals. He noticed that one strain appeared smooth because it had a capsule (S strain). Another strain appeared rough and lacked the capsule (R strain). © McGraw Hill LLC 4 The Nature of the Genetic Material 2 When mice were injected with S strain bacteria, the mice died. When mice were injected with R strain bacteria, the mice did not die. To determine if the capsule alone caused virulence of the S strain, he injected mice with heat-killed S strain bacteria. The mice did not die. © McGraw Hill LLC 5 Griffith’s Experiment Figure 25.1 Access the text alternative for slide images. © McGraw Hill LLC 6 The Nature of the Genetic Material 3 In a final experiment, Griffith injected mice with a mixture of heat-killed S strain and live R strain bacteria. The mice died. Living S strain bacteria were recovered from the bodies. Griffith concluded the following: Some substance must have passed from the dead S strain to the live R strain, transforming the R strain. That substance was necessary for bacteria to produce the capsule and be virulent. © McGraw Hill LLC 7 The Nature of the Genetic Material 4 The change in the phenotype of the R strain must be due to a change in their genotype. The transforming substance was potentially the genetic material. Scientists began looking for the transforming principle to determine the chemical nature of the genetic material. © McGraw Hill LLC 8 The Nature of the Genetic Material 5 By the 1940s, scientists recognized that genes are located on chromosomes, and that chromosomes contain both proteins and nucleic acids. A debate arose about whether DNA or protein was the genetic material. In 1944, Oswald Avery and his coinvestigators reported that DNA was the transforming substance. Thus, DNA is the genetic material. It allowed S. pneumoniae to produce a capsule and be virulent. © McGraw Hill LLC 9 The Nature of the Genetic Material 6 Results of Avery and his coinvestigators: DNA from S strain bacteria causes R strain bacteria to be transformed to produce a capsule and be virulent. The addition of DNase (an enzyme that digests DNA) prevents transformation from occurring. This supports the hypothesis that DNA is the genetic material. © McGraw Hill LLC 10 The Nature of the Genetic Material 7 Results of Avery and his coinvestigators: The molecular weight of the transforming substance is large, suggesting genetic variability. Use of protein-digesting enzymes has no effect on the transforming substance nor does RNase. This suggests that neither protein nor RNA is the genetic material. © McGraw Hill LLC 11 The Nature of the Genetic Material 8 Alfred Hershey and Martha Chase (early 1950s). Demonstrated that DNA is the genetic material, not proteins. Two experiments. Viral DNA labeled with 32P was found in the bacteria and not the medium—it had entered the bacteria. Viral protein in capsids labeled with 35S was found in medium and not in the bacterium—it never entered the bacteria. Only DNA entered the bacteria. © McGraw Hill LLC 12 Hershey–Chase Experiments Figure 25.2 Access the text alternative for slide images. © McGraw Hill LLC 13 Structure of DNA 1 Structure of DNA. James Watson and Francis H. C. Crick determined the structure of DNA in 1953. DNA is a chain of nucleotides. Each nucleotide is a complex of three subunits. Phosphoric acid (phosphate). A pentose sugar (deoxyribose). A nitrogen-containing base. © McGraw Hill LLC 14 Structure of DNA 2 Four possible bases in DNA. Two purines with a double ring. Adenine (A). Guanine (G). Two pyrimidines with a single ring. Thymine (T). Cytosine (C). DNA is a polynucleotide strand with a backbone of alternating phosphate and sugar groups. The bases are attached to the sugar and project to the side. © McGraw Hill LLC 15 Structure of DNA 3 Two polynucleotide strands make up a DNA double helix. The strands are held together by hydrogen bonding between the bases. Complementary Base Pairing. Adenine (A) always pairs with Thymine (T). Connected by two hydrogen bonds. Guanine (G) always pairs with Cytosine (C). Connected by three hydrogen bonds. A purine is always bonded to a pyrimidine. © McGraw Hill LLC 16 Structure of DNA 4 Unwound DNA helix resembles a ladder. Sides of the ladder are sugar-phosphate backbones. Rungs of the ladder are complementary base pairs. The two DNA strands are antiparallel-oriented in opposite directions. The sugars are oriented differently. The 5′ carbon atom is the uppermost on one strand, and the 3′ carbon atom is the uppermost in the other strand. © McGraw Hill LLC 17 Overview of DNA Structure 1 Figure 25.3 Access the text alternative for slide images. © McGraw Hill LLC 18 Overview of DNA Structure 2 Figure 25.3a, b Access the text alternative for slide images. © McGraw Hill LLC 19 Overview of DNA Structure 3 Figure 25.3c Access the text alternative for slide images. © McGraw Hill LLC 20 25.2 DNA Replication 1 DNA replication is the process of copying one DNA double helix into two identical double helices. Double-stranded structure of DNA allows each original strand to serve as a template for a complementary strand. © McGraw Hill LLC 21 25.2 DNA Replication 2 DNA replication is semiconservative. Each daughter DNA double helix consists of one new strand of nucleotides and one old strand conserved from the parent DNA molecule. The two daughter DNA molecules will be identical to the parent molecule. © McGraw Hill LLC 22 Overview of DNA Replication 1 Figure 25.4 Access the text alternative for slide images. © McGraw Hill LLC 23 Overview of DNA Replication 2 Figure 25.4a Access the text alternative for slide images. © McGraw Hill LLC 24 Overview of DNA Replication 3 Figure 25.4b Access the text alternative for slide images. © McGraw Hill LLC 25 25.2 DNA Replication 3 Several enzymes and proteins participate in the replication of DNA. Steps of replication: The enzyme DNA helicase unwinds and “unzips” the double-stranded DNA by breaking the hydrogen bonds between paired bases. New complementary DNA nucleotides fit into place along separated strands by complementary base pairing. These are positioned and joined by DNA polymerase. DNA polymerase uses each original strand as a template. © McGraw Hill LLC 26 25.2 DNA Replication 4 Steps of DNA Replication. Because strands are antiparallel and DNA polymerase can only add new nucleotides to one chain, DNA synthesis occurs in opposite directions. Leading strand follows DNA helicase. Lagging strand synthesized in Okazaki fragments. DNA ligase connects Okazaki fragment and seals breaks in the sugar-phosphate backbone. The two double helix molecules are identical to each other and to the original DNA molecule. © McGraw Hill LLC 27 Molecular Mechanism of DNA Replication Figure 25.5 Access the text alternative for slide images. © McGraw Hill LLC 28 25.2 DNA Replication 5 Several chemotherapeutic drugs for cancer treatment stop replication and, therefore, cell division. Some are analogs that have a similar structure to one of the four nucleotides in DNA. When these analogs are mistakenly used by the cancer cells to synthesize DNA, replication stops, and the cancer cells die off. © McGraw Hill LLC 29 25.3 Gene Expression 1 Gene expression. The process of using a gene sequence to synthesize a protein. Relies on different types of RNA (ribonucleic acid). Messenger RNA (mRNA). Transfer RNA (tRNA). Ribosomal RNA (rRNA). © McGraw Hill LLC 30 25.3 Gene Expression 2 Gene expression includes two processes. Transcription. Takes place in the nucleus. A portion of DNA serves as a template for mRNA formation. Translation. Takes place in the cytoplasm. Sequence of mRNA bases (complementary to those in the template DNA) determines the sequence of amino acids in a polypeptide. tRNA assists by bringing amino acids to the ribosome. © McGraw Hill LLC 31 Overview of Gene Expression Figure 25.6 Access the text alternative for slide images. © McGraw Hill LLC 32 Transcription During transcription, a gene (segment of DNA) serves as a template to produce an RNA molecule. Historical definition. A gene was a nucleic acid sequence that coded for sequences of amino acids in a protein. Proposed new definition. A gene represents a segment of genetic material that codes for functional products, which may be either DNA or polypeptides (proteins). © McGraw Hill LLC 33 Messenger RNA 1 Messenger RNA (mRNA) serves to carry genetic information from DNA to the ribosomes for protein synthesis. mRNA is formed by the process of transcription. Transcription begins when RNA polymerase binds to a promoter (in DNA). The DNA helix is opened so complementary base pairing can occur. RNA polymerase joins new RNA nucleotides in a sequence complementary to that on the DNA. © McGraw Hill LLC 34 Messenger RNA 2 When mRNA forms, it has a sequence of bases complementary to the DNA. Wherever A, T, G, or C is present in the DNA template, U, A, C, or G, respectively, is incorporated into the mRNA molecule. The mRNA is a faithful copy of the sequence of bases in DNA. © McGraw Hill LLC 35 Transcription of DNA to Form mRNA Figure 25.7 Access the text alternative for slide images. © McGraw Hill LLC 36 Processing of mRNA Processing of mRNA occurs in eukaryotic cells. Pre-mRNA contains bases complementary to both intron and exon segments of DNA. Introns are noncoding, intergenic, and often regulatory segments that get removed. Exons are portions of a gene that are needed to produce a protein and are joined to form a mature mRNA molecule. A guanine cap is added to the 5′ end. A poly-A tail is added to the 3′ end. The mature mRNA molecule is ready. © McGraw Hill LLC 37 mRNA Processing Figure 25.8 Access the text alternative for slide images. © McGraw Hill LLC 38 Translation Translation is the second process by which gene expression leads to protein synthesis. Translation occurs at the ribosome and requires several enzymes and different types of RNA molecules. mRNA. tRNA. rRNA. © McGraw Hill LLC 39 The Genetic Code 1 Triplet code: each three-nucleotide unit of an mRNA molecule is called a codon. There are 64 different mRNA codons. 61 code for particular amino acids. Degenerate code—most amino acids are coded for by more than one codon. Provides some protection against mutations. Three are stop codons (UAA, UGA, UAG) that signal termination. The genetic code is almost universal in all living organisms. © McGraw Hill LLC 40 The Genetic Code 2 Figure 25.9 top Access the text alternative for slide images. © McGraw Hill LLC 41 The Genetic Code 3 Figure 25.9 bottom Access the text alternative for slide images. © McGraw Hill LLC 42 Transfer RNA Transfer RNA (tRNA) transports amino acids to ribosomes. Boot-like shape due to base pairing within the one strand. Amino acid binds to one end, and the opposite end has an anticodon: Triplet of three bases complementary to a specific codon of mRNA. Order of mRNA codons determines the order in which tRNA brings in amino acids. Codon (mRNA) Anticodon (tRNA) Amino Acid (protein) CGG GCC Arginine © McGraw Hill LLC 43 Transfer RNA: Amino Acid Carrier Figure 25.10 Access the text alternative for slide images. © McGraw Hill LLC 44 Ribosomes and Ribosomal RNA 1 Ribosomes. Exist as free or attached to endoplasmic reticulum. Composed of many proteins and several ribosomal RNAs (rRNAs). Two ribosomal subunits (small and large). Subunits join in cytoplasm just before translation is to occur. Has binding site for mRNA and three tRNAs. Binding sites facilitate complementary base pairing between tRNA anticodons and mRNA codons. Polypeptide forms as new tRNA molecules arrive. Translation terminates at mRNA stop codon. © McGraw Hill LLC 45 Ribosomes and Ribosomal RNA 2 After the initial portion of mRNA is translated by one ribosome, the ribosome begins to move down the mRNA. Then other ribosomes may attach to the mRNA. Several ribosomes may move along the same mRNA. Multiple copies of a polypeptide may be made. The entire complex is called a polyribosome. © McGraw Hill LLC 46 Polyribosome Structure and Function Figure 25.11 Access the text alternative for slide images. © McGraw Hill LLC (d): Omikron/Science Source 47 Translation Requires Three Steps During translation, codons on mRNA base pair with anticodons on tRNA molecules carrying specific amino acids. The order of codons determines the order of tRNA entering the ribosome and the sequence of amino acids in a polypeptide. The process must be orderly. Translation involves three steps. Initiation (requires energy). Elongation (requires energy). Termination. © McGraw Hill LLC 48 Initiation 1 Initiation brings all the translation components together. Initiation factors are required to assemble: Small ribosomal subunit, mRNA, initiator tRNA, and the large ribosomal subunit. Small ribosomal subunit attaches to mRNA. The initiator tRNA (UAC) attaches to the start codon (AUG) on mRNA. Large ribosomal subunit joins to small subunit. The ribosome has three binding sites for tRNA. A (amino acid) site, P (peptide) site, and E (exit) site. © McGraw Hill LLC 49 Initiation 2 Figure 25.12 Access the text alternative for slide images. © McGraw Hill LLC 50 Elongation 1 Elongation is the process by which the polypeptide increases in length. This occurs one amino acid at a time. In addition to tRNA, proteins called elongation factors are also required. Facilitate the binding of tRNA anticodons to mRNA codons at the ribosome. © McGraw Hill LLC 51 Elongation 2 Elongation consists of a series of four steps. A tRNA with attached peptide is at the P site, and a tRNA carrying the next amino acid is arriving at the A site. After the next tRNA is in place at the A site, the peptide chain will be transferred to this tRNA. Two tRNAs can be at a ribosome at one time. The tRNA anticodons are paired to the mRNA codons. © McGraw Hill LLC 52 Elongation 3 Energy and part of the ribosomal subunit are needed to bring about this transfer. The energy contributes to peptide bond formation, which attaches the peptide chain to the new amino acid at the A site. Next, translocation occurs: the mRNA moves forward one codon length, and the peptide- bearing tRNA is now at the ribosome P site. The “spent” tRNA exits from the E site. The new codon is at the A site and ready to receive the next complementary tRNA. © McGraw Hill LLC 53 Elongation 4 Figure 25.13 Access the text alternative for slide images. © McGraw Hill LLC 54 Termination 1 Termination is the final step in protein synthesis. Termination of polypeptide synthesis occurs when the ribosome comes to a stop codon. Termination requires a release factor protein, which binds to the A site where the stop codon is exposed and hydrolyzes the bond between the last tRNA at the P site and the polypeptide. The polypeptide is free to take on its final three- dimensional shape. The ribosome dissociates into two subunits. © McGraw Hill LLC 55 Termination 2 Figure 25.14 Access the text alternative for slide images. © McGraw Hill LLC 56 Termination 3 Properly functioning proteins are of paramount importance. If an organism inherits a faulty gene, a genetic disorder like Huntington’s disease can result or cancer. Proteins are the link between genotype and phenotype. The DNA sequence underlying these proteins distinguishes different types of organisms. Proteins account for the differences between organisms in addition to difference between cell types. © McGraw Hill LLC 57 Review of Gene Expression 1 A gene is expressed when a protein has been synthesized; requires transcription and translation. Transcription A segment of a DNA strand serves as a template for the formation of messenger RNA (mRNA). The bases in mRNA are complementary to those in DNA. Every three mRNA bases is a codon (a triplet code) for a certain amino acid. Messenger RNA is processed before it leaves the nucleus, during which time the introns are removed and the ends are modified. Messenger RNA carries a sequence of codons to the ribosomes. © McGraw Hill LLC 58 Review of Gene Expression 3 Translation tRNAs bring attached amino acids to the ribosomes. Because tRNA anticodons pair with codons, the amino acids become sequenced in the order originally specified by DNA. The genes we receive from our parents determine the proteins in our cells and these proteins are responsible for our inherited traits. © McGraw Hill LLC 59 Review of Gene Expression 2 Figure 25.15 Access the text alternative for slide images. © McGraw Hill LLC 60 25.4 Control of Gene Expression 1 The human body contains many cell types that differ in structure and function. Each cell type contains its own specific protein combination to distinguish it from other cells. Therefore, only certain genes are active in cells that perform specialized functions. Such as nerve, muscle, gland, and blood cells. Housekeeping genes govern functions that are common to many types of cells. Active in many cell types for routine functions. © McGraw Hill LLC 61 25.4 Control of Gene Expression 2 The activity of selected genes accounts for the specialization of cells. Gene expression is controlled in a cell, and this control accounts for its specialization. Access the text alternative for slide images. © McGraw Hill LLC 62 Control of Gene Expression in Prokaryotes 1 Escherichia coli can use various sugars as a source of energy and carbon. It can quickly adjust its gene expression based on which sugar is available and will metabolize. The enzymes needed to digest lactose (milk sugar) are encoded together in an operon of the E. coli DNA. © McGraw Hill LLC 63 Control of Gene Expression in Prokaryotes 2 An operon is a cluster of genes usually coding for proteins related to a certain metabolic pathway, along with the short DNA sequences that coordinately control their transcription. Control sequences. Promoter: a sequence of DNA where RNA polymerase attaches and transcription begins. Operator: a sequence of DNA in the lac operon where a repressor protein binds. © McGraw Hill LLC 64 The lac Operon Figure 25.16 Access the text alternative for slide images. © McGraw Hill LLC 65 Control of Gene Expression in Prokaryotes 3 The lac operon in E. coli includes three genes that code for enzymes needed for lactose metabolism and are under the control of one promoter and one operator. When lactose is absent: A repressor protein binds to the operator. RNA polymerase cannot transcribe the three genes of the operon (genes are not expressed). The lac repressor is encoded by a regulatory gene located outside the operon. © McGraw Hill LLC 66 Control of Gene Expression in Prokaryotes 4 When lactose is present: Lactose binds with lac repressor. The repressor is unable to bind to the operator. RNA polymerase is able to transcribe the genes. Lactose-digesting enzymes are produced. The lac operon is considered an inducible operon. Only activated when lactose induces its expression. Repressible operons are usually active until a repressor turns them off. © McGraw Hill LLC 67 Control of Gene Expression in Eukaryotes In prokaryotes, a single promoter serves several genes that make up a transcription unit or operon. In eukaryotes, each gene has its own promoter. Eukaryotes employ a variety of mechanisms to regulate gene expression. Affect whether the gene is expressed, the speed with which it is expressed, and how long it is expressed. © McGraw Hill LLC 68 Levels of Gene Control 1 Eukaryotic gene expression is controlled at different levels: Pretranscriptional control. Transcriptional control. Posttranscriptional control. Translational control. Posttranslational control. © McGraw Hill LLC 69 Levels of Gene Control 2 Pretranscriptional Control. DNA methylation and chromatin packing are used to keep genes turned off. Genes located within heterochromatin (darkly staining portions of chromatin) are inactive. Barr body in mammalian females to inactivate one X chromosome. One or other X chromosome in a cell is randomly inactivated during early prenatal development by chromosome condensation into heterochromatin. Cells that form from each cell will have same X chromosome inactivated. Heterozygous females are mosaics (example: calico cats). © McGraw Hill LLC 70 X-Inactivation Figure 25.17 Access the text alternative for slide images. © McGraw Hill LLC (photo): Linn Currie/Shutterstock 71 Levels of Gene Control 3 Pretranscriptional Control. Active genes are found in euchromatin (loosely packed areas of chromatin). Still needs to be “unpacked” before it can be transcribed. Chromatin remodeling complex pushes aside nucleosomes to open up DNA for expression. Access the text alternative for slide images. © McGraw Hill LLC 72 Levels of Gene Control 4 Epigenetic Inheritance. Variations in the pattern of inheritance that are not due to changes in the sequence of the DNA nucleotides, but rather to changes to the DNA that alters gene expression. Also, inheritance patterns that do not depend on the genes themselves. Explains unusual inheritance patterns; may play an important role in growth, aging, and cancer. © McGraw Hill LLC 73 Levels of Gene Control 5 Genomic imprinting. A form of epigenetic inheritance that involves the methylation of the DNA molecule. Either the mother’s or the father’s gene (but not both) is methylated during gamete formation. If an inherited allele is highly methylated, the gene is not expressed, even if it is a normal gene in every other respect. For traits that exhibit genomic imprinting, the expression of the gene depends on whether the unmethylated allele was inherited from the mother or the father. © McGraw Hill LLC 74 Levels of Gene Control 6 Transcriptional Control. This control depends on interactions between certain proteins and particular DNA sequences. The proteins are called transcription factors and activators. The DNA sequences are called promoters or enhancers. Transcription factors help RNA polymerase bind to a promoter. © McGraw Hill LLC 75 Levels of Gene Control 7 The transcription initiation complex is the joining of several transcription factors at a promoter and helps pull double-stranded DNA apart for transcription to begin. The same transcription factors can be used at other promoters. Transcription activators are proteins that speed transcription dramatically. They bind to enhancer regions to further stimulate transcription. © McGraw Hill LLC 76 Levels of Gene Control 8 Posttranscriptional Control. After transcription, mRNA is processed before leaving the nucleus. Primary mRNA is converted to a mature mRNA. Addition of poly-A tail and a guanine cap. Removal of introns and splicing of exons. The same mRNA can be spliced in different patterns to generate slightly different proteins upon translation. The speed of transport of mRNA out of the nucleus can ultimately affect the amount of gene product following transcription. © McGraw Hill LLC 77 Levels of Gene Control 9 Translational Control. The longer the mRNA is available in the cytoplasm, the more gene product can be translated. Differences in the poly-A tails and/or guanine caps may determine how long an mRNA is available for translation. Specific hormones may also affect the longevity of mRNA. © McGraw Hill LLC 78 Levels of Gene Control 10 Posttranslational Control. Some proteins must be activated after synthesis. For example, insulin is activated by the enzymatic removal of a sequence of 30 amino acids. Chemical modifications such as phosphorylation may also affect the activity of a protein. Many proteins function only for a short time before they are degraded or destroyed by the cell. © McGraw Hill LLC 79 Levels at Which Control of Gene Expression Occurs in Eukaryotic Cells Figure 25.18 Access the text alternative for slide images. © McGraw Hill LLC 80 25.5 Gene Mutations and Cancer A gene mutation is a permanent change in the sequence of bases in DNA. Effects can range from changing expression of a gene to complete inactivity of a protein. Germ-line mutations occur in sex cells and can be passed to subsequent generations. Some germ-line mutations can be responsible for susceptibility to cancer in individuals. Somatic mutations, which are not passed on to the next generation, can also lead to cancer development. © McGraw Hill LLC 81 Causes of Mutations 1 Spontaneous mutations arise due to abnormalities in normal biological processes. Induced mutations result from environmental influences. Errors in DNA Replication. DNA replication errors are a rare mutation source. Proofreading by DNA polymerase usually minimizes errors in the new strands. Mismatched pairs are usually replaced. Typically, there is one error per one billion nucleotide pairs replicated. © McGraw Hill LLC 82 Causes of Mutations 2 Mutagens. Mutagens are environmental influences that can cause mutations. Radiation, X-rays, ultraviolet (UV) radiation, certain organic chemicals such as cigarette smoke, and certain pesticides are mutagens. DNA repair enzymes constantly monitor and repair any irregularities, generally keeping the mutation rate due to mutagens low. © McGraw Hill LLC 83 Causes of Mutations 3 Transposons—jumping genes. Specific DNA sequences that move within and between chromosomes. Sometimes alters neighboring gene expression in new location. Usually by increasing or decreasing the gene’s expression. Likely all organisms (including humans) have them. © McGraw Hill LLC 84 Causes of Mutations 4 Transposons. The presence of white kernels in corn is due to a transposon within a gene for a pigment-producing enzyme. “Indian corn” displays a variety of colors and patterns. Charcot–Marie–Tooth disease is a rare neurological disease in humans and is caused by a transposon (Mariner). Causes muscles and nerves of the legs and feet to gradually wither away. © McGraw Hill LLC 85 Transposon Figure 25.19 Access the text alternative for slide images. © McGraw Hill LLC (c): Mondae Leigh Baker 86 Effect of Mutations on Protein Activity 1 Point mutations involve a change in a single DNA nucleotide. One type is a base substitution: one DNA nucleotide is replaced with another incorrect nucleotide. Possible outcomes: May have no effect at all. May produce an incomplete protein (STOP codon). May cause change in a specific amino acid. May produce an abnormal protein. Sickle-cell disease is due to a base substitution. © McGraw Hill LLC 87 Point Mutations Figure 25.20 Access the text alternative for slide images. © McGraw Hill LLC 88 Mutation and Sickle-Cell Disease Figure 25.21 Access the text alternative for slide images. © McGraw Hill LLC (photos): Eye of Science/Science Source 89 Effect of Mutations on Protein Activity 2 Frameshift mutations. One or more nucleotides are either inserted or deleted from DNA. The result can be a completely new sequence of codons and a nonfunctional protein. Example: THE CAT ATE THE RAT. If “C” is deleted, the sequence becomes: THE ATA TET HER AT. © McGraw Hill LLC 90 Nonfunctional Proteins 1 A single nonfunctioning protein can have a dramatic effect on the phenotype, because enzymes are often a part of metabolic pathways. PKU (phenylketonuria) and albinism. If a person inherits a faulty code for enzyme EA, then phenylalanine cannot be broken down and builds up, causing mental impairment. If a person inherits a faulty code for enzyme EB, then tyrosine cannot be converted to melanin, causing albinism. © McGraw Hill LLC 91 Nonfunctional Proteins 2 Androgen insensitivity. Faulty receptor makes cells insensitive to androgens, which are male sex hormones such as testosterone. Even though testosterone is present in the blood, cells are unresponsive to it. As a consequence, female external genitals form instead of male genitals. Female secondary characteristics also develop. A genetic male looks like a normal female. © McGraw Hill LLC 92 Mutations Can Cause Cancer 1 In the U.S., the three deadliest forms of cancer are lung, colorectal, and breast cancer. Development of cancer involves a series of accumulating mutations that can be different for each type of cancer. Carcinogenesis begins with the loss of tumor suppressor gene activity and/or gain of oncogene activity. As a result, cell division occurs uncontrollably. © McGraw Hill LLC 93 Mutations Can Cause Cancer 2 Tumor suppressor genes and proto-oncogenes code for transcription factors or proteins that control transcription factors. These are of fundamental importance to DNA replication and repair, cell growth and division, control of apoptosis, and cell differentiation. Therefore, it should not be surprising that inherited or acquired defects in transcription factor function/structure contribute to cancer development. © McGraw Hill LLC 94 Mutations Can Cause Cancer 3 p53 is a major tumor suppressor gene that is more frequently mutated in human cancers than any other known gene. It acts as a transcription factor that controls genes whose products are cell cycle inhibitors. It also promotes apoptosis when needed. © McGraw Hill LLC 95 Mutations Can Cause Cancer 4 The retinoblastoma (RB) protein controls the activity of a transcription factor for cyclin D and other genes whose products promote entry into the S stage of the cell cycle. When the tumor suppressor gene p16 mutates, it is unable to control the cell cycle, the RB protein is continuously expressed, and that results in too much cyclin D in the cell. © McGraw Hill LLC 96 Mutations Can Cause Cancer 5 Although cancers vary greatly, they usually follow a common multistep progression. Most cancers begin as an abnormal cell growth that is benign, or not cancerous, and usually does not grow larger. Additional mutations may occur and the growth may become malignant, meaning that it is cancerous and possesses the ability to spread. © McGraw Hill LLC 97 Progression of Cancer Figure 25.22 Access the text alternative for slide images. © McGraw Hill LLC 98 Characteristics of Cancer Cells 1 Cancer cells are genetically unstable. Cancer generation appears to be linked to mutagenesis. A cell acquires a mutation that allows it to continue to divide. Eventually one of the progeny cells acquires another mutation and gains the ability to form a tumor. Tumor cells undergo multiple mutations and also tend to have chromosomal aberrations and rearrangements. © McGraw Hill LLC 99 Characteristics of Cancer Cells 2 Cancer cells do not correctly regulate the cell cycle. The normal controls of the cell cycle do not function to stop the cycle and allow the cells to differentiate. Cancer cells tend to be unspecialized due to lack of differentiation. Both the rate of cell division and the number of cells increase. © McGraw Hill LLC 100 Characteristics of Cancer Cells 3 Cancer cells can escape the signals for cell death. A cell with genetic damage or problems to the cell cycle will initiate apoptosis. Cancer cells do not respond to internal signals to die. They continue to divide even with genetic damage. Cancer cells also ignore signals from the immune system that induce apoptosis. Cancer cells also avoid the role of telomeres, which limit the number of cell divisions before they die. © McGraw Hill LLC 101 Characteristics of Cancer Cells 4 Cancer cells can survive and proliferate elsewhere in the body. Cancer cells seem to disrupt the normal adhesive mechanism and move to another place within the body. They travel through the blood or lymphatic vessels and invade new tissues to form new tumors, a process known as metastasis. Angiogenesis provides blood vessels that bring blood, nutrients, and oxygen to the tumor, depriving the surrounding normal tissues. © McGraw Hill LLC 102 End of Main Content Because learning changes everything. ® www.mheducation.com © McGraw Hill LLC. All rights reserved. No reproduction or distribution without the prior written consent of McGraw Hill LLC.