DNA Structure Unit 1 Notes PDF
Document Details
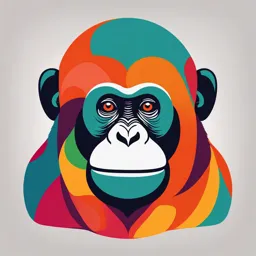
Uploaded by GlowingSwan
KIIT University
Tags
Summary
These notes cover the molecular structure of DNA. They discuss DNA as the genetic material, mechanisms of inheritance, and different structures like B-DNA, A-DNA, and Z-DNA. Information regarding the role of DNA in molecular biology is explored in terms of its specific structure.
Full Transcript
Unit 1 IMTH-IV semester Molecular Biology Gene expression at the molecular level DNA as the Genetic Material To fulfill its role, the genetic material must meet four criteria. 1. Information: The genetic material must contain the information necessary to construct an entire organism...
Unit 1 IMTH-IV semester Molecular Biology Gene expression at the molecular level DNA as the Genetic Material To fulfill its role, the genetic material must meet four criteria. 1. Information: The genetic material must contain the information necessary to construct an entire organism. (coding regions/genes) 2. Transmission: During reproduction, the genetic material must be passed from parents to offspring (vertical transmission). 3. Replication: Because the genetic material is passed from parents to offspring, and from mother cell to daughter cells during cell division, it must be copied(haploid to diploid). 4. Variation: The genetic material must vary in ways that can account for the known phenotypic differences within each species (recombination). Molecular basis of inheritance Hereditary material. Genetically stable due to less mutations because of Central Dogma Thymine (methylated at 5’) Genetic information carrier, also called genes, encodes for specific protein (phenotype), unstable as contains uracil, primitive hereditary material Phenotype or trait or the end product of RNA processing, each codon on RNA codes for specific amino acid that are present on tRNA (contains anticodon), and assembles with ribosomes to form polypeptides. First amino acid formed is Methionine (AUG) DNA as the Genetic Material Avery focused on three main candidates: DNA, RNA (the other Transformation nucleic acid in cells), and protein. Avery broke open the heat-killed pathogenic bacteria and extracted the cellular contents. He treated each of three samples with an agent that inactivated one type of molecule, then tested the sample for its ability to transform live nonpathogenic bacteria. Only when DNA was allowed to remain active did transformation occur. In 1944, Avery and his colleagues Maclyn McCarty and Colin MacLeod announced that the transforming agent was DNA. Building blocks of nucleic acids DNA Is a Double Helix Rosalind Franklin and Maurice Wilkins (in early 1950s) used the x-ray diffraction to analyze DNA fibers. From the x-ray diffraction pattern it was deduced that DNA molecules are helical with two periodicities along their long axis, a primary one of 3.4 Å and a secondary one of 34 Å. In 1953 Watson and Crick postulated a three dimensional model of DNA structure that accounted for all the available data: 1. Chargaff’s rules: At the time, the relationships A = T and G = C were quite obscure, because their significance was not apparent. 2. Correct tautomeric forms of the bases. X-ray, nuclear magnetic resonance (NMR), and spectroscopic investigations have firmly established that the nucleic acid bases are overwhelmingly in the keto tautomeric X-ray diffraction photograph of a vertically oriented Na+ DNA fiber forms. in the B conformation taken by 7 Rosalind Franklin. DNA structure Chargaff (late 1940s) – DNA molecules have distinctive base compositions Chargaff’s conclusions: 1. The base composition of DNA generally varies from one species to another. 2. DNA specimens isolated from different tissues of the same species have the same base composition. 3. The base composition of DNA in a given species does not change with an organism’s age, nutritional state, or changing environment. 4. In all cellular DNAs, regardless of the species, A = T, and G = C (Chargaff rule). From these relationships it follows that, 9 the sum of the purine residues = the sum of the pyrimidine Chargaff base pairing rule: A=T; G=C [Purines (A+G)=pyrimidines (C+T)] Applies to dsDNA, important for maintaining the stability of DNA structure. Ex: The Watson–Crick Structure: B-DNA – the native (biologically functional) form of DNA 1. It consists of two polynucleotide strands that wind about a common axis with a right- handed twist to form an 20-Å-diameter double helix. 2. The two strands are antiparallel (run in opposite directions) and wrap around each other. 3. The bases occupy the core of the helix and the sugar–phosphate chains are coiled about its periphery, thereby minimizing the repulsions between charged phosphate groups. 4. The planes of the bases are nearly perpendicular to the helix axis. 5. Each base is hydrogen bonded to a base on the opposite strand to form a planar base pair (specific complementary base pairing). 6. The aromatic bases have van der Waals thicknesses of 3.4 Å and are partially stacked 12 on each other. 8. DNA can accommodate only two types of base pairs (Watson– Crick base pairs): Each adenine residue must pair with a thymine residue (A- T) and vice versa, and each guanine residue must pair with Three hydrogen a cytosine residuebonds (G-C) can and vice versa. form between G and C, symbolized G≡C, but only two can form between A and T, symbolized A=T. Both of these base pairs are interchangeable in the double helix without altering the positions of the sugar–phosphate backbone’s C1’ atoms. Any other combination of bases (e.g., A-G or A-C) would significantly distort the double helix since they would require considerable reorientation of the sugar– 13 phosphate chain. 9. B-DNA has two deep exterior grooves that wind between its sugar– phosphate chains as a consequence of the helix axis passing through the approximate center of each base pair. However, the grooves are of unequal size: The minor groove exposes that edge of a base pair from which its C1’ atoms extend, whereas the major groove exposes the opposite edge of each base pair. 14 A-DNA A-DNA structure exists if less water is present. Like B-DNA, A-DNA is a right-handed helix, but it is shorter and wider than B-DNA and its bases are tilted away from the main axis of the molecule. There is little evidence that A-DNA exists under physiological conditions. Z-DNA Z-DNA is a left-handed helix. In this form, the sugar–phosphate backbone zigzags back and forth, giving rise to its name. A Z-DNA structure can result when DNA is placed in a high-salt solution. It can arise under physiological 15 conditions if the molecule contains Structural Features of Ideal A-, B-, and Z-DNA Symmetry elements in DNA Palindrome sequences: The regions of DNA with inverted repeats of base sequence having twofold symmetry over two strands of DNA. Mirror repeats: The inverted repeat occurs within each individual strand of the DNA. Direct repeats: In direct repeats, a particular sequence is repeated. The repeats need not be adjacent to one another. Hairpin or cruciform structures Palindrome sequences are self- complementary within each strand and therefore have the potential to form hairpin or cruciform structures. Hairpin: Only a single DNA (or RNA) strand is involved. Cruciform: Both strands of a duplex DNA are involved. Mirror repeats do not have complementary sequences within the same strand and cannot form hairpin or cruciform structures. 20 DNA Can Form a Triple Helix: Triplex DNA or H-DNA H-DNA is found in polypyrimidine or polypurine tracts that also incorporate a mirror repeat. The H-DNA structure features the triple-stranded form (due to Hoogsteen pairing). A Hoogsteen base pair applies the N7 position of the purine base (as a hydrogen bond acceptor) and C6 amino group (as a donor), which bind the Watson-Crick (N3–C4) face of the pyrimidine base. Some triplex DNAs contain two pyrimidine strands and one purine strand; others contain two purine strands and one pyrimidine strand. 21 on- Double-helical DNA and RNA can be denatured on Denaturation: When a solution of duplex DNA is heated above a characteristic temperature (or exposed to extremes of pH), its native structure collapses and its two complementary strands separate and assume a flexible and rapidly fluctuating conformational state known as a random coil. The denaturation accompanied by a change in the DNA’s physical properties: The characteristic high viscosity of native DNA solutions, drastically decreases when the DNA denatures. 22 The denaturation is mainly due When the temperature or pH is returns back, the DNA renatures to native state (Renaturation). For partially denatured DNA: Renaturation is a rapid one-step process, the denatured areas spontaneously rewind, or anneal, to yield the intact duplex. For completely denatured DNA: Step 1 (slow): the two strands “find” each other by random collisions and form a short segment of complementary double helix. Step 2 (fast): The remaining unpaired areas of the strands “zipper” themselves together to form the double helix. Melting point: The temperature at the midpoint of the 23 Hybridization Hybridization: The ability to form hybrids between two single-stranded nucleic acids is attributed to renaturation. The renaturation of DNA molecules permits artificial hybrid DNA molecules to be formed by slowly cooling mixtures of denatured DNA from two different sources. Hybrids can be formed between complementary strands of DNA and RNA. Hybridization, is the basis for several indispensable techniques, such as Southern blot hybridization and DNA microarray analysis Hyperchromicity The close interaction between stacked bases (base stacking interactions) and complementary base pairing in double strand DNA decreases the absorption of UV light relative to the single strand DNA. When DNA denatures, its UV absorbance increases by 40% at wavelengths mainly the 260 nm. This phenomenon is known as the hyperchromic effect or hyperchromicity. The hyperchromic effect results from the disruption of the electronic interactions among nearby bases. The transition from double-stranded DNA to the single-stranded, denatured 25 form can thus be detected by Effect of G-C on Tm The Tm increases linearly with the mole fraction of G- C base pairs: triply hydrogen bonded G-C base pairs are more stable than doubly hydrogen bonded A- T base pairs. Careful determination of the melting point of a DNA specimen, under fixed conditions of pH and ionic strength, can yield an estimate of its base composition.Tm is proportional to GC%, So, AT is easy to unwind faster than GC, This property of faster AT unwinding is a major 26 feature of origin of replication.Ori, rich Cot1/2 curve: Graphical representation of renaturation kinetics of ssDNA, depends on DNA 1. DNA is isolated from an organism. complexity and size (cocn X time) 2. The DNA is cut into pieces (of about 450 bp; for example) by sonication or high-speed blending. 3. The DNA concentration is measured 4. The tube is placed in boiling water to denature DNA duplexes. 5. The tube then placed in a water bath to allow denaturation. 6. At specific intervals (specific cot values), the renaturation (% of dsDNA and ssDNA) is measured and plotted in a graph. I, renatures first, Cot1/2 less, has repeats Cot (palindrome, inverted repeats, microstaellites, VNTRS, etc), More GC faster analysis renaturation owing II, renatures to stability second, Cot1/2 of moderate, has moderate repeats eukaryo (transposons, LINES, SINES) tic III, renatures last, Cot1/2 genome maximum, has unique seqs 27 Cot analysis The rate of renaturation of DNA depends on: 1. Temperature, 2. Ionic composition, and 3. Concentration of DNA. When the temperature and ionic strength are fixed, the rate of renaturation is inversely proportional to the concentration. Cot value = Conc. of DNA (M) x renaturation time (sec). Cot1/2 = Conc. of DNA (M) x time to reach 50% renaturation (sec). 29 The shape of a "Cot" curve for a given species is a function of two factors: -the size or complexity of the genome; -the amount of repetitive DNA within the genome The size or complexity of the genome The "Cot" curves of the genome of bacteriophage lambda, E. coli and yeast have the same shape, but Cot½ of yeast is largest, E. coli next and lambda smallest. The larger the genome size the longer it will take for any one sequence to encounter its complementary sequence in the solution, and greater is its complexity. 1 C value paradox: why eukaryotic genome sizes vary The range in C values does not correlate well with the complexity of the organism. This phenomenon is called the C value paradox. Why? C-Value Paradox nisms like Amoeba which is a unicellular, can have 100 times more DNA than hum cellular organisms. Ex. Amoeba proteus, dubia hibians, even though belong to same class or family have different geno. C-value - The amount of DNA per haploid cell (usually expressed as picograms) or the number of kilobases per haploid cell Expectation: 1. The more complex the organism, the more DNA is needed. 2. A linear relationship between genome size and organism complexity. At the lower range of complexity, this holds: Bacteria have smaller genomes than eukaryotes, and viruses have smaller genomes than bacteria. In larger organisms, relationship breaks down: Organisms have DNA apparently in excess of what is needed; repetitive sequences, junk DNA. C-value Paradox: In the most complex organisms Chromosome organization and molecular structure Genome organization: How the genome of an organism is arranged in the cell? Genome- Viral genomes A viral genome or chromosome can be DNA or RNA, but not both is singlestranded or double- stranded can be linear (Phages) or circular (pathogenic) Mostly contains genes without introns Considerably smaller in comparison to multicellular ℷ (Lambda) phage Genome (Ly and Lyso) Cos sites Prokaryote genomes Example: E. coli 89% coding 4,285 genes 122 structural RNA genes Prophage remains Insertion sequence (IS) elements Horizontal transfers Prokaryotic genome organization: Haploid circular genomes (0.5-10 Mbp, 500- 10000 genes) Operons: polycistronic transcription units Environment-specific genes on plasmids and other types of mobile genetic elements Usually asexual reproduction, great variety of recombination mechanisms Transcription and translation take place in the same compartment Bacterial chromosomes Key features: Most, but not all, bacterial species contain circular chromosomal DNA. A typical chromosome is a few million base pairs in length. Most bacterial species contain a single type of chromosome, but it may be present in multiple copies. Several thousand different genes are interspersed throughout the chromosome. Histone like proteins (HU, SMC, IFS etc) present- DNA accessory proteins One origin of replication is required to initiate DNA replication (bidirectional). Repetitive sequences may be interspersed throughout the chromosome. They may play a role in a variety of genetic processes, including DNA folding, replication, Condensed DNA Microbes/ Small molecules : Basic in nature: Polyamines Bacteria examples: Spermine, Spermidine SMC-proteins ( Muk B EF, helps during cell divison ) HU-proteins Compaction or DNA packaging IHF DNA bending or flexibility FIS DNA bending or flexibility All these proteins are generally basic in Nucleoid: entangled or irregular structure comprisisng the genetic material or DN s distributed horughout the cytoplasm of prokaryotes Histone HU is like proteins a small, andand basic, SMC (Structural mainetance of chromosomes- cohesins thermostable dimeric DNA-binding protein initially isolated as a factor stimulating the expression of phage lambda genes. It is a major structural component of the nucleoid, and it is conserved among the majority of eubacteria. HU is also present in archaea, in plant chloroplasts, and in a eukaryotic virus. HU of E. coli was shown to be a “histone-like Stereodiagram of HU protein: 90 protein” which can introduce negative supercoiling into a closed residues-Bacillus circular DNA in presence of stereothermophilis. Highly topoisomerase I conserved protein. Abundant protein , ~ 60,000 copies per cell The formation of chromosomal loops and DNA supercoiling helps make the bacterial chromosome more compact: DNA topology DNA packaging/coiling Positive supercoiling: Negative supercoiling: DNA unpack and replication transcription The chromosomal DNA in bacteria is negatively supercoiled. In E. coli, about one negative supercoil per 40 turns of the double helix. Supercoiling makes chromosomal DNA much more compact. Negatively supercoiling makes the separation of the two strands of DNA easier during replication and transcription (due to under rotation). Eukaryotic genome organization 1. Multiple genomes: nuclear, plastid: mitochondria, chloroplasts 2. Plastid genomes resemble prokaryotic genomes 3. Multiple linear chromosomes, total size 5- 10,000 MB, 5000 to 50000 genes 4. Monocistronic transcription units 5. Discontinuous coding regions (introns and exons) Eukaryotic genome organization (contd.) 6. Large amounts of non-coding DNA 7. Transcription and translation take place in different compartments 8. Variety of RNAs: Coding (mRNA, rRNA, tRNA), Non-coding (snRNA, snoRNA, microRNAs, etc). 9. Often diploid genomes and obligatory sexual reproduction 10.Standard mechanism of recombination: meiosis Eukaryotic chromosomes Packaging 30 nm Core histone proteins Solenoid model The nucleosome is the fundamental unit (building blocks) of chromosome packaging Each nucleosome is composed of a core of eight histone proteins and the DNA wrapped around them. Linker DNA: The DNA between each nucleosome. Length is variable – each eukaryote has a characteristic average linker DNA length. Core DNA: Typical length is 20–60 bp. The DNA most tightly associated with the nucleosome. wound about 1.65 times around the outside of the histone octamer like thread around a spool. Length is invariant in all eukaryotic cells – 146 or 147-bp length. By assembling into nucleosomes, the DNA is compacted approximately sixfold. Histones are small, positively charged proteins Nucleosomes showing core histone proteins Molecular model for nucleosome structure Nucleosomes showing linker histones and nonhistone This drawing shows two views of a nucleosome that are at right angles to each proteins other. The horizontal bar passes through the center of the nucleosome in each view. Core histones: Two copies each of H2A, H2B, H3, and H4 Each of the core histones has a flexible “tail,” containing from 11 to 37 amino acids, that extends out from the nucleosome. Positively charged amino acids (arginines, in particular) in the tails of the histones interact with the negative charges of the phosphates on the DNA, and the tails of one nucleosome may interact with neighboring nucleosomes. Chemical modifications of these histone tails (phosphorylation, acetylation, and methylation on serine, lysine, and arginine residues) bring about changes in chromatin structure that are necessary for gene expression. Linker histone: H1 H1 binds to 20 to 22 bp of DNA where the DNA joins and leaves the octamer and helps to lock the DNA into place, acting as a clamp around the nucleosome octamer. Chromatosome: Together, the nucleosomes and its associated H1 histone. In addition, nonhistone proteins bound to the linker region The repeating nucleosome structure is revealed by digestion of the linker region The data of the experiment At high DNase I concentrations, the entire sample of chromosomal DNA was digested into fragments of approximately 200 bp in length, which is predicted by the beads-on-a-string model. At lower DNase I concentrations, longer pieces were observed, and these were in multiples of 200 bp (400, 600, etc.). How do we explain these longer pieces? They occurred because occasional linker regions remained uncut at lower DNase I concentrations. 1. What is the basic unit of DNA packaging? Nucleosome. 2. Nucleosome components: Histone proteins octamer. Core histones + Linker H1 3. What is the charge of native nucleosome and why: positive, lysine, arginine so that it can bind to negatively charged DNA due to phosphate and hydroxyl. 4. What is the first order of DNA packaging: formation of 11 nm DNA structure (Beads on a string): Nucleosome model. 5. What is the second order of DNA packaging: Chromatosome: Solenoid model: 30 nm fibril. 6 nucleosomes/turn. 6. What is the third order of DNA packaging: Chromomere formed due to coiling of 30 nm fibril. Chromomere is a bead like structure or loop like strusture arranged in a linear order of 300 nm diameter This is the final order of DNA packaging finally forming chromonemata: coiled filament through the entire lenghth of chromosomes. Wrapping of the DNA around the histone protein core stores negative superhelicity Each nucleosome changes the linking number of the associated DNA by approximately –1.2. The DNA that is packaged into nucleosomes would become negatively supercoiled if nucleosomes were removed from the DNA. Why would the cell want to maintain a stockpile of negative superhelicity? There are many instances when it is useful to drive unwinding of DNA in the cell, including initiation of DNA replication, transcription, and recombination. Importantly, negatively supercoiled DNA favors DNA unwinding. Thus, removal of a nucleosome not only allows increased access to the DNA, but also facilitates DNA unwinding of If nucleosomes nearby store negative DNA sequences. superhelicity in eukaryotic cells, what serves the equivalent function in prokaryotic cells? Topisomerase enzyme (DNA gyrase) The compaction level of interphase chromosomes Euchromatin may vary Heterochromatin Having a relatively Tightly compacted regions of open structure chromatin The 30-nm fiber Radial loop domains become forms radial loop compacted even further domains Transcriptionally Transcriptionally inactive - active – high gene very limited gene expression expression Staining poorly with Dense staining with a dyes variety of dyes Associated with particular Electron micrograph of a nucleus chromosomal regions, including the telomere and the centromere, and is important for their functions. Nucleosome s Chromatin structure during interphase DNA topology DNA is a flexible structure – its exact molecular parameters are a function of both the surrounding ionic environment and the nature of the DNA-binding proteins. Linear DNA molecules: Because their ends are free, linear DNA molecules can freely rotate to accommodate changes in the number of times the two chains of the double helix twist about each other. Covalently closed, circular DNA (cccDNA): topologically constrained - the absolute number of times the chains can twist about each other cannot change since the two ends are covalently linked. Linear DNA molecules of eukaryotic chromosomes: topologically constrained because of their extreme length, entrainment in chromatin, and interaction with other cellular components. Then, how the cell both accommodates and exploits topological constraints during DNA replication, transcription, and other chromosomal transactions? DNA topology features DNA exists in different topological forms in vivo and in vitro DNA topoisomerases catalyze the interconversion of DNA forms Negative superhelicity (underwinding) helps proteins ( of replication and transcription machinery )bind DNA by favoring unwinding of the helix. DNA compaction during chromatin organization and condensation during mitosis involves topological transformations. Twist-no. of helical turns Writhe: knots, no. of times one strand passes through the other. Linking number: parameter for measuring DNA topology, includes twist and writhe depending on the state of DNA (constrained or relaxed) Primary structure of DNA: linear arrangement of nucleotides (ATGC)(sequential) Secondary structure of DNA: Double helical strutrure (Watson-Crick model) Tertiery structure: Supercoiled structure: H DNA (Triple helical DNA), Quadruplets DNA (G DNA) etc. Take home messages: DNA topology DNA topology is arrangement of 1. different forms of DNA. In solution form, B DNA is the most prevalent form-10.5bps/turn. (Twist/Unit twist). Topologically stable form: B DNA because thermodynamically it is the most stable form: stored free energy is minimum. Supercoiling: it can relax strain (underwound) as well as add strain (during packaging, overwound). Postive supercoil (overwound): Right handed turn or twist, clockwise Negative supercoil: (underwound) Left 2. Lkw= Tw + Wr, Relaxed DNAnhanded withouttwist supercoiling, wr=0, os or turn, counterclockwise Lk0=Tw= no. of bps/10.5 3. Change in linking number, delta LK (linking difference, also called writhe)= Lk- Lko Linking number The linking number L of DNA is a topological property. L: can be used to denote the degree of supercoiling. L: defines the number of times a strand of DNA winds in the around the other strand along the helix axis; Twist If both strands are covalently closed, the linking number in a relaxed state cannot change without breaking the bond in one of the strands; For instance, in a circular DNA of 5200 basepairs, the linking number is 5250/10.5=500, where 10.5 is the base-pair per turn for type B DNA. Practice Questions Q1. A circular relaxed DNA molecule ( a plasmid ) consists of 1300 bp and has 10 bp/turn (NOTE: B-DNA has 10.5 bp/turn, but this makes the math easier!). The double-stranded helix is right-handed. a) What is the Lk of the relaxed form of this DNA molecule? What is the Tw and Wr of the relaxed form of this molecule? Lk= 1300/ 10 = 130 , Tw= 130 , Wr=0 ( molecule is flat, no writhes when DNA is relaxed) b) An enzyme (topoisomerase II) introduces left-handed twists into the double- stranded DNA and changes the number of twists/turns by -6. Is the DNA being underwound or overwound by topoisomerase II? Underwound (Negative coiling), b/c introducing opposite direction twists into a right-handed DNA structure c) Assume that no writhes are introduced yet after step (circular state) b). Is the plasmid DNA under torsional strain at this point? What will be the new Lk, Tw and Wr? Yes! Writhes will want to be formed to compensate for the torsinal strain. Previous Tw=Lk0= 130, New Tw=130-6= 124=Lk, so, Tw= 124, Lk=124, Wr= 0 d) The plasmid DNA releases its torsional strain by forming writhes. Given the information in this question are the writhes left (-) or right-handed (+)? NA in Cells Is negatively supercoiled (Writhe is negativ Both bacterial and eukaryotic DNA usually folds into loops stabilized by proteins, and supercoiling takes place within the loops. Most DNA found in cells is negatively supercoiled. Advantages of negative supercoiling: Negatively supercoiling makes the separation of the two strands of DNA easier during replication and transcription (due to under rotation) Supercoiled DNA can be packed into a smaller space than can relaxed DNA. Exceptions: certain thermophiles (Thermus aquaticus : Taq polymerase bacteria) have positively supercoiled DNA. Sulfolobus acidocaldarius is an aerobic thermoacidophilic Archea. It grows optimally at 75 to 80°C and pH 2 to 3 Extrememe Temps, denaturation. Starn sperate. DNA loose structure. DNA will protected Nucleosomes introduce negative supercoiling in eukaryotes Writhe in nucleosomes: left-handed toroid or Spiral writhe; negative supercoiling. Topoisomerases can relax supercoiled DNA In prokaryotes and eukaryotes, topoisomerases remove the topological constraints by introducing transient single-strand or double-strand breaks into the DNA. Catalyse the formation of topoisomers:- Cut+pass one strand through another+paste_ Topoisomerases I cuts a single strand of Topoisomerases II binds to DNA, the DNA duplex, passes the uncut strand creates a double-strand break, through the break, and then reseals the passes uncut DNA through the gap, break. The process increases the linking and then reseals the break. These number by +1. These enzymes do not enzymes hydrolyse ATP for energy. require ATP. Change Lk by multiples of 2 Both prokaryotes and eukaryotes have type I and type II topoisomerases that are capable of removing supercoils from DNA. Prokaryotes have a special topoisomerase that introduces supercoils into DNA (Gyrase) DNA gyrase (acts during prokaryotic replication contrast to nucleosomes In eukaryotes) (negative supercoil) A special type II topoisomerase present in prokaryotes that introduces, rather than removes, negative supercoils. This enzyme is responsible for the negative supercoiling of chromosomes in prokaryotes. Reduces linking number. Dlk0. By keeping the genome positively supercoiled, reverse gyrase counteracts the effect of thermal denaturation that would ordinarily result in many regions of the genome being Topoisomerases also unknot and disentangle (seperate) DNA moleculesafter replication Catenated DNA molecules are commonly produced in prokaryotes as a round of DNA replication is finished. During DNA replication in eukaryotes, the two double- stranded daughter DNA molecules will often become entangled. Some site-specific recombination reactions give rise to knotted DNA products Topoisomerases cleave DNA using a covalent tyrosine–DNA intermediate Catalytic site- tyrosine For simplicity, only a single strand of DNA is shown. Type II topoisomerases also follow the same mechanism is used by, although two enzyme subunits are required, one to cleave each of the two DNA Topoisomers can be separated by electrophoresis Lane A: Relaxed or nicked Separation of relaxed and supercoiled circular DNA DNA by gel electrophoresis. The speed Lane B: Linear DNA with which the DNA molecules migrate Lane C: Highly supercoiled increases as the number of superhelical cccDNA turns increases. Lane DNAD:topoisomers a ladder of-topoisomers cccDNA of the same length but of different linking numbers. 13.3 – Topoisomerases Visualization of Supercoiling on an Electrophoretic gel Cathode (-) Review: On an agarose electrophoretic gel, small DNA fragments migrate towards the bottom of the gel (anode). Why? Smaller fragments can move through the agarose more quickly. Larger DNA fragments get trapped and stay higher up on the gel (closer to the cathode). In Lane #1: Supercoiled DNA molecules are found lower on the gel (because they are smaller in overall size). Anode (+) Relaxed DNA molecules are found higher on the gel (because they are larger in overall size). If smaller, pass faster and goes lower quicker! 13.3 – Topoisomerases Visualization of Supercoiling on an Electrophoretic gel Cathode (-) In Lane #2: The supercoiled DNA becomes more relaxed upon being treated with DNA Topoisomerase Type I. Different topoisomers can be seen of the DNA. In Lane #3: Same treatment with DNA Topoisomerase Type I. Different topoisomers can be seen of the DNA. Q12. Which Lane #2 or #3 represents DNA that has been treated with Anode (+) Topoisomerase Type I for a longer period of time? Lane #3, because increase [topoisomerase] that are closer to relaxed Lk. Remember topo type I removes supercoils