History of Data Communications PDF
Document Details
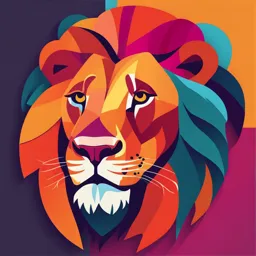
Uploaded by SuppleIndianapolis6251
Aldrin Cedre
Tags
Summary
This presentation provides a detailed overview of the history of data communications, tracing its evolution from early methods like smoke signals and telegraphs to modern digital systems. It examines key advancements like the telegraph, telephone, and the development of computers, highlighting the increasing complexity and speed of communication across time.
Full Transcript
Presentation 04 01 02 03 Optical Telegraphs Data communications began long before recorded time in the form of smoke signals or tom-tom drums. Soon these methods were replaced by flags and later, optical telegraphs. The Semaphore Telegraph System was eventually adopted and widely implemented...
Presentation 04 01 02 03 Optical Telegraphs Data communications began long before recorded time in the form of smoke signals or tom-tom drums. Soon these methods were replaced by flags and later, optical telegraphs. The Semaphore Telegraph System was eventually adopted and widely implemented all throughout France. At the time of French revolution and Napolean prominence, the initial motivation to implement Semaphore Telegraph System was military usage. Stations were built 10 to 15 km apart, operators did not know the exact message, but only relaying the symbols which will be decoded at the final receiving station. Optical Telegraphs One of the earliest means of communicating electrically coded information occurred in 1753, when a proposal submitted to a Scottish magazine suggested running a communications line between villages comprised of 26 parallel wires, each wire for one letter of the alphabet. A Swiss inventor constructed a prototype of the 26-wire system, but current wire-making technology proved the idea impractical. Optical Telegraphs In 1833, Carl Friedrich Gauss developed an unusual system based on a five-by-five matrix representing 25 letters (I and J were combined). The idea was to send messages over a single wire by deflecting a needle to the right or left between one and five times. The initial set of deflections indicated a row, and the second set indicated a column. Consequently, it could take as many as 10 deflections to convey a single character through the system. Optical Telegraphs Sir Charles Wheatstone and Sir William Cooke allegedly invented the first telegraph in England, but their contraption required six different wires for a single telegraph line. Morse Telegraphs The first successful data communications system that used electrical signals to transmit information was invented by Samuel F. B. Morse in 1832 and called the telegraph. Morse also developed the first practical data communications code, which he called the Morse code. With telegraph, dots and dashes (analogous to logic 1s and 0s) are transmitted across a wire using electromechanical induction. Most common letters could be sent with a single symbol (dot for E, dash for T), the other letters were arranged by frequency and assigned increasingly complex code. Morse Telegraphs In 1840, Morse secured an American patent for the telegraph, and in 1844 the first telegraph line was established between Baltimore and Washington, D.C., with the first message conveyed over this system being "What hath God wrought!" In 1849, the first slow-speed telegraph printer was invented, but it was not until 1860 that high-speed (15-bps) printers were available. In 1850, Western Union Telegraph Company was formed in Rochester, New York, for the purpose of carrying coded messages from one person to another. Advancements in Telegraph In 1874, Emile Baudot invented a telegraph multiplexer, which allowed signals from up to six different telegraph machines to be transmitted simultaneously over a single wire. The telephone was invented in 1875 by Alexander Graham Bell, and, which heavily contributed to the slow evolution in telegraph until 1899, when Guglielmo Marconi succeeded in sending radio (wireless) telegraph messages. Telegraph was the only means of sending information across large spans of water until 1920, when the first commercial radio stations carrying voice information were installed. Origin of Computers It is unclear exactly when the first electrical computer was developed. Konrad Zuis, a German engineer, demonstrated a computing machine sometime in the late 1930s: however, at the time, Hitler was preoccupied trying to conquer the rest of the world, so the project fizzled out. Origin of Computers Bell Telephone Laboratories is given credit for developing the first special- purpose computer in 1940 using electromechanical relays for performing logical operations. However, J. Presper Eckert and John Mauchley at the University of Pennsylvania are given credit by some for beginning modern-day computing when they developed the ENIAC computer on February 14, 1946. Origin of Computers In 1949, the U.S. National Bureau of Standards developed the first all- electronic diode-based computer capable of executing stored programs. The U.S. Census Bureau in- stalled the machine, which is considered the first commercially produced American computer. In the 1950s, computers used punch cards for inputting information, printers for outputting information, and magnetic tape reels for permanently storing information. These early computers could process only one job at a time using a technique called batch processing. The first general-purpose computer was an automatic sequence- controlled calculator developed jointly by Harvard University and International Business Machines (IBM) Corporation. The UNIVAC computer, built in 1951 by Remington Rand Corporation, was the first mass-produced electronic computer. In the 1960s, batch-processing systems were replaced by on-line processing systems with terminals connected directly to the computer through serial or parallel communications lines. The 1970s introduced microprocessor-controlled microcomputers, and by the 1980s personal computers became an essential item in the home and workplace. Since then, the number of mainframe computers, small business computers, personal computers, and computer terminals has increased exponentially, creating a situation where more and more people have the need (or at least think they have the need) to exchange digital information with each other. Consequently, the need for data communications circuits, networks, and systems has also increased exponentially. Soon after the invention of the telephone, the American Telephone and Telegraph Company (AT&T) emerged, providing both long-distance and local telephone service and data communications service throughout the United States. Until 1968, the AT&T operating tariff allowed only equipment furnished by AT&T to be connected to AT&T lines, but, a landmark Supreme Court decision, the Carterfone decision, allowed non-Bell companies to interconnect to the vast AT&T communications network. This decision started the interconnect industry, which has led to competitive data communications offerings by a large number of independent companies. In 1983, as a direct result of an antitrust suit filed by the federal government, AT&T agreed in a court settlement to divest itself of operating companies that provide basic local telephone service to the various geographic regions of the United States. Since the divestiture, the com- plexity of the public telephone system in the United States has grown even more in- volved and complicated. In the 1960s, batch-processing systems were replaced by on-line processing systems with terminals connected directly to the computer through serial or parallel communications lines. The 1970s introduced microprocessor-controlled microcomputers, and by the 1980s personal computers became an essential item in the home and workplace. Since then, the number of mainframe computers, small business computers, personal computers, and computer terminals has increased exponentially, creating a situation where more and more people have the need (or at least think they have the need) to exchange digital information with each other. Consequently, the need for data communications circuits, networks, and systems has also increased exponentially. Presentation 05 01 02 03 04 What is Transmission Media? A transmission medium is a physical path between the transmitter and the receiver i.e. it is the channel through which data is sent from one place to another. Transmission Media is broadly classified into the following types: Metallic Transmission Media Metallic Transmission Media refers to the use of conductive materials, typically copper, to transmit data in the form of electrical signals. It has been a traditional choice for various communication systems, including telephony, networking, and broadcasting. In metallic cables, data is transmitted in the form of transverse electromagnetic (TEM) waves. These waves consist of oscillating electric (E) and magnetic (H) fields, which propagate through the non-conductive insulating material (dielectric) between the conductors perpendicular to each other and the direction of wave propagation. Balanced vs. Unbalanced Lines In a balanced transmission line (e.g., twisted pair), both wires carry current, but in opposite directions. The voltage difference between the two wires is the signal. The symmetrical nature of balanced lines helps cancel out external noise, which affects both wires equally. This makes balanced lines more resistant to interference from external sources such as power lines or radio signals. Twisted- pair cables used in Ethernet (UTP or STP) are common examples of balanced lines. In an unbalanced transmission line (e.g., coaxial cable), one conductor carries the signal, while the other conductor (typically the shield) is grounded. This type of line is more prone to noise, especially over long distances, since external interference can affect the single conductor carrying the signal. However, the shielding in coaxial cables helps mitigate this issue by protecting the inner conductor from external electromagnetic fields. Transmission Line Losses As electrical signals travel through metallic cables, they lose strength due to the resistance of the conductor and the dielectric. This loss of signal strength is called attenuation, and it increases with the length of the cable and the frequency of the signal. Attenuation is more pronounced in higher-frequency signals, making it a limiting factor for long-distance and high-speed communication over metallic cables. When the signal from one cable interferes with the signal in another cable, especially in cases where multiple cables are run closely together, another type of loss known as crosstalk occurs. In twisted-pair cables, the twisting of the wires helps reduce crosstalk, as it minimizes the exposure of the wires to external interference. Shielded cables (STP and coaxial) offer additional protection against crosstalk by using shielding to block external electromagnetic fields. Electromagnetic Interference (EMI) occurs when external sources, such as electrical motors, transformers, or even fluorescent lights, create electromagnetic fields that interfere with the signal in a cable. Shielded cables (STP and coaxial) are designed to prevent this type of interference by enclosing the signal-carrying conductor in a grounded shield. Unshielded cables (e.g., UTP) are more susceptible to EMI, although proper cable installation techniques, such as avoiding power lines, can minimize its effects. Types of Metallic Cables Open-wire transmission lines consist of two parallel copper wires separated by air or another insulator. Although simple in construction, open-wire systems are vulnerable to environmental factors like weather and physical interference. The lack of shielding makes them susceptible to electromagnetic interference (EMI) and crosstalk, where signals in one wire can interfere with those in adjacent wires. Types of Metallic Cables Twin-lead cables are similar to open-wire, but with a solid dielectric insulator keeping the two conductors at a fixed distance. This type of cable is commonly used in older TV antenna setups. The continuous dielectric provides better consistency in transmission, reducing interference, but it still lacks the shielding found in more advanced cables. Types of Metallic Cables Unshielded Twisted Pair cables consist of two copper wires twisted around each other. The twisting helps reduce EMI by ensuring that external interference affects both wires equally, allowing the interference to cancel out. UTP is widely used in telephone networks and Ethernet LANs. Categories of UTP are defined by the number of twists per meter, which impacts bandwidth and data transmission rates. Shielded Twisted Pair cables, on the other hand, consists of twisted pairs that are enclosed in a foil or braided shield that helps further reduce EMI and crosstalk. This makes STP more resistant to interference than UTP, but it is bulkier, more expensive, and harder to install. STP is often used in environments with high interference, such as industrial or data center applications. Types of Metallic Cables CAT-3 or Category 3 is a UTP cable used for basic telephone lines and older data networks, supporting speeds up to 10 Mbps. CAT-5 is a UTP cable widely used in modern Ethernet networks, capable of supporting speeds up to 100 Mbps (Gigabit Ethernet). CAT-5e is the STP counterpart of CAT-5 which further reduces EMI by wrapping each twisted pair with foil or mesh. CAT-6 is an improvement over CAT-5, with higher twists per foot, providing better resistance to interference and supporting speeds up to 10 Gbps. CAT-6e is the STP counterpart of CAT-6 Types of Metallic Cables Coaxial cables consist of a central conductor surrounded by an insulating layer, a metallic shield (typically braided copper or aluminum), and an outer protective jacket. The shielding prevents interference from external sources and helps contain the signal within the cable, making coaxial cables highly efficient for high-frequency applications. Coaxial cables were historically used for Ethernet (10BASE2 and 10BASE5) networks and Cable tv. Limitations & Emerging Trends While metallic cables are still widely used, their role is gradually diminishing in favor of optical fiber due to fiber’s ability to transmit data at much higher speeds over longer distances without significant signal loss. Power over Ethernet (PoE) is one area where twisted-pair cables (like CAT-5e and CAT-6) continue to evolve, as they now support both data and electrical power transmission over a single connection to devices such as cameras, Wi-Fi access points, and IP phones. Metallic Transmission Media Optical Transmission Media relies on fiber optic cables to transmit data as light signals rather than electrical signals, making it highly advantageous for high-speed, long-distance communications. Optical fibers, typically made from glass or plastic, have transformed telecommunications by enabling enormous data-carrying capacity with minimal signal degradation. Parts of Optical Fiber Systems A transmitter converts electrical data signals into light signals, using either an LED (for MMF) or laser diode (for SMF). It includes components such as a voltage-to-current converter and a light source (laser or LED) that encodes data onto the light wave. A receiver converts the light signal back into an electrical signal. The receiver typically uses a photodetector (like a PIN diode or an avalanche photodiode) to sense incoming light pulses and convert them to electrical pulses. Parts of Optical Fiber Systems Optical Fiber Cables, typically made from ultra-pure glass or plastic, connect the transmitter and receiver. In cases of longer distance transmission, repeaters might be used as buffer to ensure signal strength. Each cable typically includes: Core: The central part where light travels, made from high-quality glass or plastic. Cladding: Surrounds the core with a lower refractive index material, which helps contain light within the core by total internal reflection. Outer Protective Jacket: Provides physical protection and strength for handling, often made of tough polymers or materials like PVC. Light Propagation in Fiber Optics The idea of sending data over fiber optics is only possible through the concept of total internal reflection wherein light signals are confined within the core of the fiber. When light strikes the core-cladding boundary at an angle greater than the critical angle, it reflects back into the core, allowing the light signal to travel long distances without escaping. Types of Optical Fiber Single-Mode Fiber has a small core (about 8-10 micrometers in diameter) that allows only a single light path or mode, usually using a laser light source. This is ideal for long-distance communications, such as intercity or transcontinental links, due to minimal signal dispersion (spreading of light pulses). SMF supports very high data rates and is widely used for backbone infrastructure in telecommunications and internet data centers. Types of Optical Fiber Multimode Fiber has a larger core (typically 50-62.5 micrometers), allowing multiple light paths (modes) to travel through the core. Its commonly used with LED light sources and suitable for shorter distances, such as within buildings, data centers, and campus networks, where cost and ease of installation are prioritized over long-distance performance. Types of Optical Fiber Types of multimode fibers: Graded-Index Fiber: The refractive index gradually decreases from the core center toward the cladding, reducing modal dispersion and enhancing performance for shorter distances. Step-Index Fiber: The refractive index has a sharp boundary between core and cladding, causing more dispersion, which makes it less efficient for high-speed, long-distance data transmission Limitations & Emerging Trends Optical transmission media, while powerful, face limitations such as high initial costs, fragility, and the need for specialized maintenance due to the delicate nature of fiber connections. However, emerging trends are expanding fiber’s capabilities and accessibility. Dense Wavelength Division Multiplexing (DWDM) significantly increases data capacity by sending multiple light wavelengths through a single fiber, achieving terabit-level speeds. Fiber-to-the-Home (FTTH) now enables high-speed internet and HD streaming directly to residences, becoming a crucial part of broadband infrastructure. Additionally, Plastic Optical Fiber (POF), which is more flexible than glass fiber, is gaining popularity for short-range applications in home networking, electronics, and automotive systems, marking a continued evolution in optical technology. Wireless Transmission Media Wireless transmission media enable the transmission of electromagnetic waves wirelessly, without the use of a physical medium. While they facilitate the transfer of these waves, they do not control their direction, hence the name unguided transmission media. Radio Waves Radio waves have a frequency range of 3 KHz to 1 GHz and are easy to produce. They can travel long distances and are omnidirectional, meaning they move in all directions. Their ability to penetrate walls makes them ideal for both indoor and outdoor communication. Common applications include AM and FM radio, television, cellular phones, and wireless LAN. Microwaves Microwaves are electromagnetic waves with a frequency range of 1 GHz to 300 GHz and can cover long distances. They are unidirectional, meaning they travel in a straight line. At higher frequencies, they cannot pass through walls. Microwaves are commonly used for one-to-one communication, such as in cellular phones, satellite networks, and wireless LAN. Infrared Waves Infrared waves are electromagnetic waves with a frequency range of 300 GHz to 400 THz and are limited to short- distance communication. They rely on line-of-sight propagation and cannot pass through solid objects like walls. A common application of infrared waves is in remote controls for devices such as TVs, DVD players, and stereo systems. Limitations & Emerging Trends Wireless transmission media face limitations such as restricted bandwidth, increasing congestion as user demands grow, and susceptibility to interference from obstacles, weather, and electronic devices, which can affect signal quality. Security is also a challenge, as wireless networks are more vulnerable to interception and require strong encryption protocols. Additionally, wireless coverage weakens over distance, necessitating boosters or repeaters, particularly in remote areas. Emerging trends aim to address these challenges, with 5G networks offering faster speeds, lower latency, and high capacity for applications like IoT and smart cities. Wi-Fi 6 and Wi-Fi 7 enhance performance in crowded areas by increasing speed and reducing congestion, while millimeter-wave (mmWave) technology leverages high-frequency bands to achieve very high data rates, supporting next-generation mobile and broadband applications. Presentation 06 01 02 03 04 05 06 Data Transmission Data transmission refers to the process of sending and receiving digital or analog data between two or more devices over a communication medium. It encompasses various methods and technologies that enable devices to exchange information effectively. Data Transmission A data transmission system typically consists of five essential components: 1. Message: This is the actual data or information that needs to be communicated. Messages can take various forms, including text, images, audio, or video files. The content of the message is critical as it determines the nature of the communication. 2. Sender: The sender is the device or entity that initiates the transmission of the message. This could be any device capable of sending data, such as a computer, smartphone, or sensor. The sender encodes the message into a format suitable for transmission. 3. Receiver: The receiver is the device that receives the transmitted message from the sender. It decodes and interprets the incoming data to make it usable. Like the sender, receivers can also be computers, mobile devices, or other types of electronic equipment. Data Transmission 4. Transmission Medium: This refers to the physical pathway through which data travels from sender to receiver. Transmission mediums can be classified into two categories: a. Guided Media: Includes physical cables such as twisted pair cables, coaxial cables, and fiber optic cables. b. Unguided Media: Involves wireless transmission methods such as radio waves, microwaves, and infrared signals. 5. Protocol: Protocols are sets of rules and conventions that govern how data is transmitted over a network. They define aspects such as data format, timing of transmissions, error detection and correction methods, and how devices establish connections with one another. Without protocols, communication between devices would not be possible. Data Transmission Modes Data Transmission Modes Direction of Information Bit Transmission Type Flow Synchronization Level The first classification of data transmission modes is based on the direction of information flow, which plays a crucial role in how data is exchanged between devices. There are three primary modes: simplex, half-duplex, and full-duplex. Simplex Mode In simplex mode, communication is unidirectional, meaning data flows in only one direction. This mode is akin to a one-way street where the sender transmits data without the ability to receive any feedback from the receiver. Common examples include keyboard-to- computer communication or television broadcasts, where information is sent from the source to the end-user without any return path. Half-Duplex Mode Half-duplex mode allows for two-way communication, but not simultaneously; only one device can send or receive data at a time. This can be likened to a single lane street where traffic can flow in both directions, but only one direction can move at a time. A practical example of half- duplex communication is the use of walkie- talkies, where one party must finish speaking before the other can respond. This mode is beneficial in scenarios where simultaneous communication isn't necessary, allowing for error detection and retransmission if needed. Full-Duplex Mode In contrast, full-duplex mode enables simultaneous two-way communication, allowing both devices to send and receive data at the same time. This mode maximizes efficiency and speed, as it utilizes the available bandwidth more effectively. Telephones are a classic example of full-duplex communication; both parties can talk and listen simultaneously without waiting for the other to finish. Full-duplex systems are essential in applications requiring real-time interaction, such as video conferencing or online gaming. The concept of synchronization levels in data transmission is crucial for understanding how data is effectively communicated between devices. This can be categorized primarily into synchronous and asynchronous transmission, each with distinct characteristics that affect the efficiency and reliability of data transfer. Synchronous Transmission In synchronous transmission, data is sent in a continuous stream, with both the sender and receiver synchronized by a shared clock signal. This synchronization allows for the transmission of data in fixed intervals, often organized into blocks or frames. Because there are no gaps between these blocks, synchronous transmission is typically faster and more efficient, making it suitable for high-speed applications such as video conferencing and real-time communications. The lack of start and stop bits reduces overhead, allowing for a more streamlined flow of information. This method is particularly advantageous for transferring large amounts of data quickly, as the continuous nature minimizes latency and maximizes throughput. Asynchronous Transmission Conversely, asynchronous transmission operates without a shared clock signal, relying instead on start and stop bits to indicate the beginning and end of each character or byte transmitted. This method allows for irregular intervals between characters, which can introduce delays but also provides flexibility in communication. Each piece of data is sent independently, meaning that the sender does not need to wait for the receiver to be ready before sending the next byte. While this can lead to increased latency due to the time gaps between transmissions, asynchronous transmission is simpler to implement and more cost-effective, making it suitable for applications like email or keyboard input where speed is less critical. Understanding bit transmission types is essential for grasping how data is communicated between devices, particularly in the context of digital and data communications. The two primary methods of bit transmission are serial transmission and parallel transmission, each with its unique characteristics, advantages, and limitations. Serial Transmission In serial transmission, data is sent one bit at a time over a single communication channel. This method can be likened to a single-lane road where vehicles (data bits) travel sequentially, one after the other. Each bit is transmitted in a specific order, often accompanied by start and stop bits to indicate the beginning and end of the data packet. This sequential approach allows for reliable data transfer over long distances, as it minimizes issues related to timing and synchronization. Serial transmission is commonly used in various protocols such as RS- 232 and USB, making it a versatile choice for connecting devices across significant distances. While it typically operates at lower speeds compared to parallel transmission, its simplicity and reliability make it ideal for applications where distance is critical. Parallel Transmission On the other hand, parallel transmission sends multiple bits simultaneously across multiple channels or wires. This method can be visualized as a multi-lane highway where several vehicles (data bits) can travel side by side at the same time. For instance, an 8-bit byte can be transmitted in one go using eight separate wires. This simultaneous transfer allows for much faster data rates compared to serial transmission, making parallel communication suitable for short- distance applications where speed is essential, such as within computer systems or between closely located devices. However, parallel transmission comes with its challenges; as the distance increases, signal degradation and timing issues can arise due to the need for all bits to arrive simultaneously. This makes parallel transmission less reliable over long distances. Protocols in data communication are essential frameworks that establish the rules and conventions governing how data is transmitted between devices in a network. These protocols ensure that communication is effective, reliable, and standardized across diverse systems. At their core, protocols define several critical aspects of data exchange, including what information is to be communicated, how it should be formatted, and when it should be sent. Syntax One of the primary functions of a protocol is to specify the syntax of the data being transmitted. This includes the structure or format of messages, ensuring that both the sender and receiver understand how to interpret the bits being exchanged. For example, protocols dictate whether data is sent as plain text, binary code, or in specific file formats like JPEG or MP3. Additionally, protocols encompass semantics, which provide meaning to the various sections of data packets. This ensures that both parties can accurately interpret the information being shared. Timing Timing is another critical element governed by protocols. They establish rules for when data can be sent and how quickly it should be transmitted. This aspect is particularly important in real-time applications such as video conferencing or online gaming, where delays can significantly impact user experience. Protocols also incorporate mechanisms for error detection and correction, enabling devices to identify and rectify any discrepancies that may occur during transmission. This is vital for maintaining data integrity and ensuring that the information received matches what was originally sent. Numerous protocols exist to facilitate different types of communication. For instance, the Transmission Control Protocol/Internet Protocol (TCP/IP) ensures reliable transmission by establishing a connection-oriented communication channel that guarantees delivery through acknowledgments and retransmissions. In contrast, the User Datagram Protocol (UDP) allows for faster communication by sending messages without establishing a connection first, making it suitable for applications where speed is more critical than reliability. Standards in Networking are critical frameworks that ensure interoperability, reliability, and efficiency in data communication across various devices and networks. These standards are established by recognized organizations and serve as guidelines that define the technical requirements, specifications, and protocols necessary for devices to communicate effectively. By adhering to these standards, manufacturers can create products that work seamlessly together, regardless of the vendor, which is essential in today’s diverse technological landscape. IEEE The Institute of Electrical and Electronics Engineers (IEEE) is one of the most prominent organizations responsible for developing networking standards. For instance, the IEEE 802 series defines standards for local area networks (LANs), including the widely used IEEE 802.3 for Ethernet and IEEE 802.11 for wireless LANs (Wi-Fi). These standards specify everything from physical connections to data link layer protocols, ensuring that devices can communicate over both wired and wireless networks. ISO Another significant body is the International Organization for Standardization (ISO), which develops international standards across various industries, including networking. The ISO/IEC 7498-1 standard outlines the Open Systems Interconnection (OSI) model, a conceptual framework that standardizes the functions of a telecommunication or computing system into seven layers. This model helps in understanding how different networking protocols interact and ensures that systems from different manufacturers can work together. OSI Model The ISO/IEC 7498-1 standard, commonly known as the Open Systems Interconnection (OSI) model, serves as a foundational framework for understanding and implementing network communication. The OSI model is structured into seven layers, which are organized hierarchically from the lowest physical layer to the highest application layer. The Physical Layer (Layer 1) deals with the transmission of raw bitstreams over a physical medium, including specifications for cables, switches, and electrical signals. The Data Link Layer (Layer 2) is responsible for node-to-node data transfer and error detection and correction, ensuring that data packets are transmitted accurately between directly connected devices. Above this, the Network Layer (Layer 3) manages routing and forwarding of data packets across multiple networks, determining how data should be sent from source to destination. OSI Model The Transport Layer (Layer 4) ensures complete data transfer and provides error recovery and flow control mechanisms. It segments messages into smaller packets for transmission and reassembles them at the destination. The Session Layer (Layer 5) establishes, manages, and terminates sessions between applications, handling communication sessions effectively. The Presentation Layer (Layer 6) translates data formats, encrypts information for secure transmission, and ensures that data is presented in a readable format for the application layer. Finally, the Application Layer (Layer 7) interfaces directly with end- user applications, providing network services such as file transfers and email communication. TIA & IETF The Telecommunications Industry Association (TIA) also plays a vital role in setting standards related to telecommunications cabling and equipment. Standards such as TIA-568 govern structured cabling systems, ensuring compatibility among different manufacturers’ equipment and facilitating efficient data transmission in both commercial and residential settings. Furthermore, the Internet Engineering Task Force (IETF) is instrumental in developing standards specifically for internet protocols. The IETF’s Request for Comments (RFC) documents outline protocols like the Transmission Control Protocol (TCP) and Internet Protocol (IP), which form the backbone of internet communication. These documents are crucial for ensuring that data packets are routed correctly across networks and that applications can communicate over the internet reliably.