Processes of Evolution PDF
Document Details
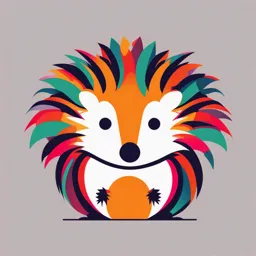
Uploaded by AstonishedDaisy8276
Tags
Related
- Natural Selection and Evolution 2024 Year 10 Science PDF
- Biology 1 – Cells, Molecular Biology and Genetics (Biol 1000) Lecture Notes PDF
- Lecture 8: Evolution and Natural Selection PDF
- Bio125 Study Questions: Natural Selection & Evolution - PDF
- Evolution of Populations & Population Genetics - PDF
- Selection & Evolution A2 Level Chapter 17 - Cambridge Assessment PDF
Summary
This document covers the processes of evolution, including key concepts such as how mutations, natural selection, and other factors contribute to evolution. It also explores examples like Darwin's observations. The document is likely suitable for an undergraduate biology course.
Full Transcript
19 Processes of Evolution © Oxford University Press Chapter 19 Processes of Evolution Key Concepts 19.1 Evolution Is Both Factual and the Basis of Broader Theory 19.2 Mutation, Selection, Gene Flow, Genetic Drift, and Nonrandom Matin...
19 Processes of Evolution © Oxford University Press Chapter 19 Processes of Evolution Key Concepts 19.1 Evolution Is Both Factual and the Basis of Broader Theory 19.2 Mutation, Selection, Gene Flow, Genetic Drift, and Nonrandom Mating Result in Evolution 19.3 Evolution Can Be Measured by Changes in Allele Frequencies 19.4 Selection Can Be Stabilizing, Directional, or Disruptive 19.5 Selection Can Maintain Variation within and among Populations 19.6 Evolution Is Constrained by History and Trade-Offs © Oxford University Press 19.1 Evolution Is Both Factual and the Basis of Broader Theory Evolution is the change in genetic composition of populations over time. Evolutionary theory is the understanding of the mechanisms of evolutionary change. © Oxford University Press 19.1 Evolution Is Both Factual and the Basis of Broader Theory Applications of evolutionary theory: Study and treatment of diseases Developing better agricultural crops and industrial processes Understanding the diversification of life and how species interact Allows predictions about the biological world © Oxford University Press Figure 19.1 Darwin and the Voyage of the Beagle 19.1 Evolution Is Both Factual and the Basis of Broader Theory In the Galápagos Islands he observed species that were similar to, but not the same as, species on the mainland of South America, and that species varied from island to island. Darwin postulated that species had reached the islands from the mainland, but then had undergone different changes on different islands. © Oxford University Press 19.1 Evolution Is Both Factual and the Basis of Broader Theory These observations led Darwin to propose a theory for evolutionary change based on three propositions: Species change over time. Divergent species share a common ancestor, and species have diverged gradually through time (descent with modification). The mechanism that produces the change is natural selection: the differential survival and reproduction of individuals based on variation in their traits. Darwin also observed that humans practice artificial selection by breeding plants and animals with desired characteristics. Darwin published his book, On the Origin of Species, in 1859. © Oxford University Press 19.2 Mutation, Selection, Gene Flow, Genetic Drift, and Nonrandom Mating Result in Evolution Population: A group of individuals of a single species that live and interbreed in a particular geographic area. Individuals do not evolve; populations do. In addition to natural selection there are four other mechanisms of evolution: Mutation Gene flow Genetic drift Nonrandom mating © Oxford University Press 19.2 Mutation, Selection, Gene Flow, Genetic Drift, and Nonrandom Mating Result in Evolution Mutation is the origin of genetic variation. Mutation is any change in the nucleotide sequences of DNA. Mutations are random with respect to the needs of an organism; selection acting on the random variation results in adaptation. Most mutations are harmful or neutral. A few are beneficial; or if conditions change, a mutation could become advantageous. © Oxford University Press 19.2 Mutation, Selection, Gene Flow, Genetic Drift, and Nonrandom Mating Result in Evolution As a result of mutation, different forms of a gene (alleles) may exist at a particular chromosomal locus. The gene pool is the sum of all copies of all alleles at all loci in a population. The gene pool is the sum of the genetic variation in the population. © Oxford University Press Figure 19.2 A Gene Pool 19.2 Mutation, Selection, Gene Flow, Genetic Drift, and Nonrandom Mating Result in Evolution Allele frequency: Proportion of an allele in the gene pool. Genotype frequency: Proportion of each genotype in the population. Calculation of these frequencies is used to measure evolutionary change. © Oxford University Press 19.2 Mutation, Selection, Gene Flow, Genetic Drift, and Nonrandom Mating Result in Evolution Selection acts on genetic variation to produce new phenotypes. Example: Artificial selection for different traits in a species of wild mustard produced many important crop plants. © Oxford University Press 19.2 Mutation, Selection, Gene Flow, Genetic Drift, and Nonrandom Mating Result in Evolution Darwin bred pigeons and recognized that artificial selection results in traits preferred by human breeders; natural selection results in traits that help organisms survive and reproduce more effectively. In both cases, selection simply increases the frequency of the favored trait from one generation to the next. © Oxford University Press 19.2 Mutation, Selection, Gene Flow, Genetic Drift, and Nonrandom Mating Result in Evolution In experiments with Drosophila, researchers selected for high or low numbers of body bristles from an initial population with intermediate numbers. After 35 generations, numbers for both high-bristle and low-bristle fell outside the range of the original population. © Oxford University Press 19.2 Mutation, Selection, Gene Flow, Genetic Drift, and Nonrandom Mating Result in Evolution Darwin suggested that differences among individuals affect the chance that a given individual will survive and reproduce, increasing the frequency of the favored trait in the next generation. Adaptation: A favored trait that spreads through a population by natural selection. © Oxford University Press 19.2 Mutation, Selection, Gene Flow, Genetic Drift, and Nonrandom Mating Result in Evolution Gene flow results from migration of individuals and movements of gametes (e.g., pollen) between populations. New individuals can add alleles to the gene pool or change allele frequencies. Genetic drift results from random changes in allele frequencies. Harmful alleles may increase in frequency, and rare advantageous alleles may be lost. In large populations, genetic drift can influence frequencies of neutral alleles. © Oxford University Press 19.2 Mutation, Selection, Gene Flow, Genetic Drift, and Nonrandom Mating Result in Evolution In small populations, genetic drift can be significant. Population bottleneck: Environmental conditions result in survival of only a few individuals. Genetic drift can reduce genetic variation in the population. © Oxford University Press 19.2 Mutation, Selection, Gene Flow, Genetic Drift, and Nonrandom Mating Result in Evolution Genetic drift also affects small populations that colonize a new region. The colonizing population is unlikely to have all the alleles present in the whole population. This is called a founder effect (equivalent to a population bottleneck). © Oxford University Press 19.2 Mutation, Selection, Gene Flow, Genetic Drift, and Nonrandom Mating Result in Evolution Nonrandom mating occurs when individuals choose mates with particular phenotypes. Example: Self-fertilization is common, especially in plants. If individuals choose the same genotype as themselves, homozygote frequencies will increase. © Oxford University Press 19.2 Mutation, Selection, Gene Flow, Genetic Drift, and Nonrandom Mating Result in Evolution Sexual selection is nonrandom mating that favors traits that increase the chances of reproduction. Darwin proposed that traits such as bright colors, long tails, and elaborate courtship displays may improve ability to compete for mates or to be more attractive to the opposite sex. Sexual selection may favor traits that enhance an individual’s chances of reproduction but reduce its chances of survival. It often results in evolution of significant differences between males and females of a species. © Oxford University Press 19.2 Mutation, Selection, Gene Flow, Genetic Drift, and Nonrandom Mating Result in Evolution This has been shown experimentally in long- tailed widowbirds. Male tails were shortened or lengthened. Both were able to successfully defend their territories, but males with lengthened tails attracted more females. © Oxford University Press 19.3 Evolution Can Be Measured by Changes in Allele Frequencies Allele frequency: If a locus has 2 alleles, A and a, there could be 3 genotypes: AA, Aa, and aa. The population is polymorphic at that locus. Much of evolution occurs through gradual changes in the relative allele frequencies in a population. Allele frequencies are estimated by counting alleles in a sample of individuals. © Oxford University Press Figure 19.10 Calculating Allele and Genotype Frequencies 19.3 Evolution Can Be Measured by Changes in Allele Frequencies If p is the frequency of allele A, and q is the frequency of allele a, p+q=1 q=1–p If there is only one allele at a locus, its frequency equals 1. The population is monomorphic at that locus; the allele is said to be fixed. © Oxford University Press 19.3 Evolution Can Be Measured by Changes in Allele Frequencies The Hardy–Weinberg equilibrium describes a model situation in which allele frequencies do not change. Conditions that must be met for Hardy–Weinberg equilibrium: No mutation No selection among genotypes No gene flow Population size is infinite Mating is random © Oxford University Press 19.3 Evolution Can Be Measured by Changes in Allele Frequencies If these conditions hold: Allele frequencies remain constant After one generation, genotype frequencies occur in these proportions: Genotype AA Aa aa Frequency p2 2pq q2 The Hardy–Weinberg equation: p2 + 2pq + q2 = 1 © Oxford University Press Figure 19.11 One Generation of Random Mating Restores Hardy–Weinberg Equilibrium (Part 1) Figure 19.11 One Generation of Random Mating Restores Hardy–Weinberg Equilibrium (Part 2) 19.4 Selection Can Be Stabilizing, Directional, or Disruptive Natural selection acts on the phenotype rather than directly on the genotype. The reproductive contribution of a phenotype to subsequent generations relative to other phenotypes is called its fitness. Only changes in the relative success of different phenotypes lead to change in allele frequencies. Fitness of a phenotype is determined by the relative rates of survival and reproduction of individuals with that phenotype. © Oxford University Press 19.4 Selection Can Be Stabilizing, Directional, or Disruptive Qualitative traits: influenced by alleles at only one locus (e.g., smooth vs. wrinkled). Quantitative traits: show continuous variation; influenced by alleles at more than 1 locus. Example: Distribution of body size in a population resembles a bell-shaped curve. © Oxford University Press 19.4 Selection Can Be Stabilizing, Directional, or Disruptive Natural selection can act on quantitative traits in three ways: Stabilizing selection preserves the average phenotype Directional selection favors individuals that vary in one direction from the mean. Disruptive selection favors individuals that vary in both directions. © Oxford University Press Figure 19.12 Natural Selection Can Operate in Several Ways 19.4 Selection Can Be Stabilizing, Directional, or Disruptive Stabilizing selection reduces variation but does not change the mean. Natural selection is often stabilizing (rates of evolution are slow). Example: Human birth weights—lighter or heavier babies die at higher rates than babies that weigh close to the mean. Stabilizing selection is often called purifying selection because there is selection against any deleterious mutations. © Oxford University Press Figure 19.13 Human Birth Weight Is Influenced by Stabilizing Selection 19.4 Selection Can Be Stabilizing, Directional, or Disruptive Directional selection: When individuals at one extreme are more successful. Directional selection results in an increase of the frequencies of alleles that produce the favored phenotype. Referring to a single gene locus, a particular variant may be favored—positive selection. © Oxford University Press 19.4 Selection Can Be Stabilizing, Directional, or Disruptive If directional selection operates over many generations, an evolutionary trend occurs. Example: Horns of Texas Longhorn cattle have evolved through directional selection; predation was the selection pressure. © Oxford University Press 19.4 Selection Can Be Stabilizing, Directional, or Disruptive In disruptive selection, individuals at either extreme are more successful than average individuals. Variation in a population is increased. © Oxford University Press 19.4 Selection Can Be Stabilizing, Directional, or Disruptive Disruptive selection: bill size in black-bellied seedcrackers Birds with large bills can crack the hard seeds of a sedge. Birds with small bills feed more efficiently on soft seeds of a different sedge species. Birds with intermediate sized bills cannot use either kind of seed efficiently and survive poorly. © Oxford University Press Figure 19.15 Disruptive Selection Results in a Bimodal Character Distribution 19.5 Selection Can Maintain Variation within and among Populations Frequency-dependent selection: A polymorphism can be maintained when fitness depends on its frequency in the population. Example: A scale-eating fish in Lake Tanganyika. o “Left-mouthed” and “right- mouthed” individuals are both favored. o The host fish can be attacked from either side. © Oxford University Press 19.5 Selection Can Maintain Variation within and among Populations Different alleles of a gene may be advantageous under different environmental conditions. Heterozygote advantage: In changing conditions, heterozygous individuals are likely to outperform homozygotes. © Oxford University Press 19.5 Selection Can Maintain Variation within and among Populations Example: Colias butterflies live in an environment with temperature extremes. o The population is polymorphic for an enzyme (PGI) that influences flight at different temperatures. o Heterozygotes are favored because they can fly over a larger temperature range. © Oxford University Press 19.6 Evolution Is Constrained by History and Trade-Offs Evolution is constrained in many ways. Lack of genetic variation can prevent evolution of potentially favorable traits. If the allele for a given trait does not exist in a population, that trait cannot evolve, even if it would be favored by natural selection. © Oxford University Press 19.6 Evolution Is Constrained by History and Trade-Offs All evolutionary innovations are modifications of existing structures. Example: Bottom-dwelling fishes. o Skates and rays evolved from a common ancestor with sharks. o They started with a flat body plan and evolved to swim along the ocean floor. © Oxford University Press 19.6 Evolution Is Constrained by History and Trade-Offs Adaptations impose both costs and benefits. Benefit must outweigh cost if an adaptation is to evolve—the trade-off must be worthwhile. Conspicuous features used by some males to compete with other males are a trade-off with reproductive success. © Oxford University Press 19.6 Evolution Is Constrained by History and Trade-Offs Trade-offs can result in traits that are adaptive in one context but not in another. Example: Rough-skinned newts make the neurotoxin tetrodotoxin (TTX), which paralyzes nerves and muscles by blocking sodium channels. o Most vertebrate predators will die if they eat one of these newts. o In some populations of garter snakes, TTX-resistant sodium channels have evolved, and they can eat the newts. o But, TTX-resistant snakes cannot move as fast as nonresistant snakes and are thus more vulnerable to their own predators. o TTX-resistance is selected against in areas outside the range of the newts. © Oxford University Press Figure 19.20 Resistance to a Toxin Comes at a Cost 19.6 Evolution Is Constrained by History and Trade-Offs Short-term changes in allele frequencies (microevolutionary changes) can be observed directly, manipulated experimentally, and they demonstrate the actual processes by which evolution occurs. Long-term patterns of evolutionary change (macroevolutionary) can be strongly influenced by events that occur so infrequently or so slowly that they are unlikely to be observed during short-term studies. Evolutionary mechanisms may change over time with changing environmental conditions. © Oxford University Press