Level 1 Genetics 2025 Study Notes PDF
Document Details
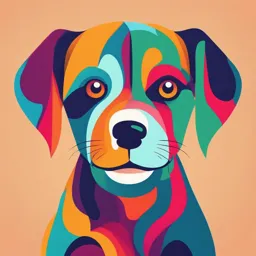
Uploaded by MindBlowingRose88
2025
Level 1 Science 2025
Tags
Summary
These Level 1 Science 2025 study notes cover genetics, DNA structure, genes, alleles, and homologous chromosomes. Key concepts like monohybrid inheritance and the genetic code are explained, along with examples and diagrams.
Full Transcript
Level 1 Science 2025 – Genetics Student Study Notes 1. Describe genetics as the study of the variation and inheritance of genes. 2. Define the terms; DNA, gene, allele and chromosome. 3. Explain the difference between genes and alleles with named examples. Introduction to Genetics Defi...
Level 1 Science 2025 – Genetics Student Study Notes 1. Describe genetics as the study of the variation and inheritance of genes. 2. Define the terms; DNA, gene, allele and chromosome. 3. Explain the difference between genes and alleles with named examples. Introduction to Genetics Definition: Genetics is the study of the variation and inheritance of genes. Definition: A gene is a section of DNA that codes for a protein that determines a particular trait. Definition: An allele is an alternative version of a gene Definition: DNA is a chemical that carries genetic instructions (as a code formed by the sequence of bases in DNA). DNA is stored as chromosomes inside the nucleus of a cell. Definition: A chromosome is an (organised) structure of DNA (found inside the nucleus of a cell). Genes vs Alleles A gene is a section of DNA that codes for a protein that determines a particular trait whereas an allele is an alternative version of a gene. Members of a species have the same genes but carry different alleles for these genes. For example, mice have a gene for coat colour. Some mice have alleles for white coat, some have alleles for brown coat colour. 4. Describe the structure of DNA as a double helix. 5. Label a diagram of a nucleotide (deoxyribose sugar, phosphate and base). 6. Describe the complementary base pairing rule A-T, C-G. DNA – Deoxyribose Nucleic Acid DNA is a double stranded molecule twisted into a double helix. DNA is made up of repeating sub-units called nucleotides. Each nucleotide is made up of a phosphate, sugar (deoxyribose), and base joined together. The phosphate of a nucleotide joins to the sugar of another nucleotide to form the backbone of each strand. Then the two strands are joined together by the bases on the different strands pairing together by complementary base pairing between bases on different strands. A (Adenine) only pairs with T (Thymine), and G (Guanine) only pairs with C (Cytosine). Full name of the base is not required - just learn the letters). 7. Explain the role of Rosalind Franklin in the development of the discovery of the structure of the DNA molecule The discovery of the structure of DNA in 1953 was made possible by Dr Rosalind Franklin's X-ray diffraction work at King's College London. Her creation of the famous Photo 51 allowed Maurice Wilkins, Francis Crick and James Watson to deduce the double-helix structure of deoxyribonucleic acid. 8. Define homologous pair of chromosomes 9. Draw a labelled diagram of homologous pair 10. Understand individuals inherit one copy of each pair from each parent. Homologous Pair of Chromosomes Definition: Homologous Chromosomes are pairs of chromosomes that are the same length, and carry the same genes at the same loci (position or location) as each other. Individuals inherit two copies of each chromosome - one from each parent. Therefore every person carries two copies of each gene (one from each chromosome they inherit). Note - homologous chromosomes may carry different alleles. Sister chromatids are duplicated chromosomes that are connected to each other at their center points (centromere). They have the same alleles as they are copies of one another. 11. Describe the genetic code as the base sequence of DNA 12. Explain that the genetic code (base sequence) is read in triplets. Triplets code for amino acids. Amino acids are ordered according to the base sequence to form different proteins 13. Describe primary protein structure as the sequence of amino acids linked together to form a polypeptide chain The Genetic Code Definition: The genetic code is the base sequence (the order of bases (A, T, C and G) in DNA) The genetic code is read in triplets. Triplets code for amino acids. Amino acids are ordered according to the base sequence to form different proteins. Hence different bases sequences produce different proteins (and different phenotypes). Different amino acid sequences form different proteins and therefore different phenotypes. For example hair is made of a protein called keratin. Different hair types and colours are due to different alleles altering the amino acid sequences. Definition: Monohybrid Inheritance is the inheritance of characteristics controlled by a single gene (mono = one). Inheritance of Genetic Variation Inheritance is the passing on of genetic information from parents to offspring. It is possible to predict and follow the inheritance of the genetic variation produced by mutations and sexual reproduction. The most basic unit of genetic variation that is inherited (passed from parents onto offspring) is the allele. Alleles - Remember: An allele is an alternative version of a gene Individuals inherit two alleles of each gene because they get an allele from each parent due to two gametes, one from each parent, being joined together to form a new individual. Each gamete has half the number of chromosomes (1 set of chromosomes), so one copy of each chromosome and gene, and so after fertilisation the offspring ends up with two copies of each chromosome and gene, and therefore will have two alleles of each gene (one from each parent). The two alleles that an individual has inherited for a gene can either be a dominant version or recessive version. Definition: Dominant means only one copy is required to be present (in the genotype) for it to be expressed as the phenotype. Definition: Recessive means it is only expressed as the phenotype if both alleles in the genotype are the same recessive allele. A dominant allele is represented by a capital letter, and the recessive allele is represented by a lower case letter e.g. for the fur colour gene, the black allele is dominant, while the white allele is recessive, so the black allele is given the capital letter “B” and the white allele is given the lower case letter “b”. Note: the same letter is used to indicate that they are alleles of the same gene (the fur colour gene). Definition: A genotype is the alleles an individual carries for a gene Different genotypes are possible depending on the two alleles an individual inherits from its parents. · Homozygous dominant genotype – both alleles present for a gene are the dominant allele e.g. BB, so the individual physically expresses the dominant phenotype because a dominant allele is present e.g. black fur · Heterozygous genotype – the two alleles present for a gene are different e.g. Bb, so the individual physically expresses the dominant phenotype e.g. black fur, but carries a recessive allele that is hidden/masked by the dominant allele because the dominant allele is always expressed as the phenotype if present · Homozygous recessive genotype – both alleles present for a gene are the recessive allele e.g. bb, so the individual physically expresses the recessive phenotype e.g. white fur (because a dominant allele is not present) Definition: A phenotype is the physical expression/appearance of the genotype e.g. black fur Note 1: When writing genotypes, capital and lower case of the same letter is used to show that they are alleles of the same gene and the dominant allele (capital) is always written first. Note 2: There are 3 different genotypes, but only two phenotypes because both the homozygous dominant genotype and the heterozygous genotype produce the dominant phenotype due to the dominant allele always being expressed as the phenotype if it is present in the genotype, and so it can mask/hide a recessive allele in the heterozygous genotype. Whereas, the recessive phenotype can only be expressed/produced by the homozygous recessive genotype because the recessive is only expressed if both alleles in the genotype are the same recessive allele (a dominant allele cannot be present). The likelihood that an offspring will inherit a particular allele from a parent, and therefore a particular genotype and phenotype can be predicted using Punnett squares. Punnett Squares When two parents are reproduced together it is called a “cross” e.g. Parent 1 is crossed with Parent 2, and a Punnett square allows you to predict the proportion of offspring that should have certain phenotypes from the cross of two parents, this is called the expected phenotype ratio of the cross. Note: Punnett squares DO NOT show the actual offspring produced, or the number of offspring the parents produce. A Punnett square only predicts the possible genotypes and phenotypes that offspring of those parents could have and the proportion that each phenotype is expected to occur in the offspring. The genotype of each parent needs to be known to be able to complete a Punnett Square, and the parents’ genotypes/alleles must be for the same gene(s) (so the alleles must be the capital or lower case of the same letter). From the cross above, there is a 50% chance that parent 1 will pass on either a dominant B allele or a recessive b allele to its offspring, while parent 2 can only pass on a dominant B allele to its offspring. Therefore, the expected phenotype ratio is that 100% of the offspring will show the dominant phenotype of black fur because all the offspring will receive a dominant B allele from parent 2. Note: if one of the parents can only ever pass on a dominant allele, then all the offspring will have the dominant phenotype. Note: When writing an expected phenotype ratio you always write the dominant phenotype first and the recessive phenotype last, and always include the phenotype inside brackets beside the number in the ratio e.g. 3 (black) : 1 (white) Expected phenotype ratios vs Observed phenotype ratios The expected phenotype ratio from a Punnett square might be different to the actual phenotype ratio observed in the offspring produced. The expected and observed phenotype ratios can be different because each fertilisation is an independent random chance event, which is unaffected by previous outcomes so the chance of an outcome (a genotype or phenotype) occurring remains the same for every offspring produced. Therefore, if the sample size is small (e.g. a small number of offspring are produced), then it is possible that the expected phenotype ratio might not observed due to random chance. If more offspring are produced then you are more likely to get closer to observing the expected phenotype ratio. · The cross between two heterozygous parents (cross 4 above) and the cross between a heterozygous parent and a homozygous recessive parent (cross 5 above) are the two crosses that you should remember how to write the expected phenotype ratios for. Therefore, if only a small number of offspring are produced (if the sample size is small), it is possible, due to random chance, that the heterozygous parent passes on its dominant allele for each fertilisation, and so only offspring with the dominant phenotype are produced, which would make you incorrectly think the heterozygous parent was pure breeding. However, if many offspring are produced, so the sample size is larger, then it is more likely you will get closer to observing the expected phenotype ratio of 50% of the offspring showing the recessive phenotype, which would identify that the parent with the dominant phenotype must have a heterozygous genotype and not be pure breeding so that the offspring to be able to inherit a recessive allele from each parent. 49. Be able to interpret pedigree charts to track inheritance over generations and to determine genotype of individuals Pedigree Charts A Pedigree chart is a diagram that allows you to follow the inheritance of genetic variation across generations, and can be used to determine the genotype and phenotype of individuals in the chart. To determine the genotypes and phenotypes of individuals in the chart, you will need to work out which characteristic/phenotype (colour shape) is dominant by finding two parents on the pedigree chart who show the same characteristic/phenotype (= shapes coloured the same), but who have offspring that show different phenotypes (= shapes coloured differently). This means both parents must be showing the dominant characteristic and have a heterozygous genotype because some of their offspring have the recessive phenotype so must be able to inherit a recessive allele from each parent, and some of their offspring have the dominant phenotype so must also be able to inherit at least one dominant allele (do a Punnett square to show this). If either parent had a homozygous dominant genotype then the offspring would always inherit one dominant allele and so the offspring could only show the same dominant phenotype (do a Punnett square to show this). If the parents were both showing the recessive phenotype they would both have to have homozygous recessive genotypes and so could only have offspring showing the recessive phenotype because each parent could only ever pass on a recessive allele, so offspring would always inherit two recessive alleles (do a Punnett square to show this). Explaining the genotypes of individuals in a pedigree chart: Once you have established which phenotype (colour shape) is dominant, you will need to explain the genotypes of other individuals in the chart based on the alleles they could possibly inherit from their parents, and/or if the individual has offspring, what alleles they must have to pass on to produce offspring with the phenotypes shown. E.g. In the pedigree chart above, the black shapes must be showing the dominant phenotype because the parents of individual 1 are both black shapes (so have the same phenotype), but produce offspring that have different phenotypes (indicated by the offspring having different coloured shapes), so the parents must be showing the dominant phenotype and have heterozygous genotypes. Therefore, individual 1 is showing the dominant phenotype because it is a black shape, but its genotype might be homozygous dominant or heterozygous because both its parents are heterozygous so there is a 25% chance it could inherit two dominant alleles, one from each parent, or a 50% chance it could inherit a dominant allele from one parent and a recessive allele from the other parent (support this with a Punnet square). The only way we could know its genotype from a pedigree chart is if it had offspring. Individual 2 is also showing the dominant phenotype because in this pedigree chart the black shapes are the dominant phenotype, and it must have a heterozygous genotype because it has produced offspring with different phenotypes (indicated by the different coloured shapes), which is only possible if individual 2 is able to pass on either a dominant or recessive allele (support this with a Punnett square). Note: if all 4 offspring of individual 2 showed the dominant characteristic then it is likely that individual 2 would have a homozygous dominant genotype. However, more offspring would need to be produced to be more certain of individual 2’s genotype because each fertilisation is an independent random chance event, not affected by previous outcomes, so the chance of an outcome remains the same, and 4 offspring is a small sample size so individual 2 could be heterozygous but by chance has only passed on its dominant allele to the offspring. 50. Describe DNA Sequencing 51.Discuss the advantages of using DNA sequencing over tracking inheritance with punnett squares and pedigree charts 52. Discuss how DNA sequencing is used in Genetic Screening of Stomach Cancer in NZ 53. Discuss how DNA sequencing is used in Genetic Screening of Cystic Fibrosis in NZ Definition: DNA sequencing is the process of identifying the exact order of the bases in a DNA strand. DNA sequencing has advantages over Punnett squares and pedigree charts in tracking inheritance because DNA sequencing: Provides exact information about an individual's genetic code, including specific base sequence changes (mutations) and any variations in genes, or alleles compared to normal versions. Identifies carriers of genetic conditions and silent mutations that do not appear physically but can be passed on to offspring. Provides information about genetic predispositions to diseases and guides personalized medicine and advice. E.g. Identification of a mutation in the CDH1 gene leads to regular checks for cancer or may choose to surgically remove the stomach. Does not require information about the individual’s family history of the disorder. Whereas with Punnett squares and pedigree charts: Punnett squares are only a prediction of inheritance and do not provide exact information about an individual's genetic code and which mutations/alleles have been inherited as fertilisation is a random process and each fertilisation is independent of others. Punnett squares/pedigree charts would require information about the individual’s family history of the disorder. Visible traits/phenotypes are required to be expressed or existing offspring to infer inheritance patterns, delaying the detection of diseases. Cannot detect carriers without observing the potential carrier’s offspring and/or traits being expressed in the phenotypes e.g. Stomach cancer developing. Context 1 = Stomach Cancer: · Stomach cancer can be caused by a mutation in the CDH1 gene. · Carriers of the mutated gene have a 70% higher chance of developing stomach cancer and female carriers a 30-40% higher risk of developing breast cancer. Māori have rates of stomach cancer 3x higher than Europeans due to a higher incidence of the CDH1 allele. · A blood or saliva sample is collected and the DNA is sequenced. Normal sequence chromatograms are compared with the sample chromatogram. · If a carrier of the gene is identified the patient can undergo regular checks for cancer or may choose to surgically remove the stomach. Context 2 = Cystic Fibrosis: · Cystic Fibrosis can be caused by a mutation in the CFTR gene which is recessive. · ·Individuals who inherit 2 copies of the mutated CF allele have a cystic fibrosis which affects the cells that produce mucus, sweat and digestive juices. These secreted fluids are normally thin and slippery but in people with CF, the defective gene causes the secretions to become sticky and thick. Instead of acting as lubricants, the secretions block passageways, especially in the lungs and digestive system causing a higher rate of lung infection and lower nutrition absorption. · Individuals with a family member with Cystic Fibrosis and their partner may choose to have their DNA sequenced to see if they are carriers of the mutated CFTR gene before having children. A blood or saliva sample is collected and the DNA is sequenced. Normal sequence chromatograms are compared with the sample chromatograms. · If a carrier of the gene is identified in both patients can undergo genetic counselling to decide whether or not to have biological children. 54. Describe selective breeding. Definition: Selective Breeding involves choosing parents with particular characteristics to breed together and produce offspring with more desirable characteristics. The aim is to produce a population that is pure bred for certain characteristics. 55. Explain what test crosses are and how they can be used to determine the genotype of individuals with dominant phenotypes Pure Breeding and Test Crossing Definition: Pure breeding is when an individual is homozygous for a trait So pure breeding individuals carry two of the same alleles in their genotype, and so always pass on the same type of allele to offspring, and so if they are bred with another individual showing the same phenotype they always produce offspring with the same phenotype (refer to Punnett squares 1, 3, and 6 above) However, an individual showing the dominant phenotype could have a homozygous genotype and be pure breeding, or have a heterozygous genotype and not be pure breeding, because the dominant allele is always expressed as the phenotype if it is present and so hides/masks the recessive allele in the heterozygous genotype that could be passed onto offspring. Therefore, you have to do a test cross to work out the genotype of an individual showing the dominant phenotype and identify whether it is pure breeding or not. Note: You do not need to do a test cross of an individual showing the recessive phenotype because the recessive phenotype can only be expressed when an individual carries two of the same recessive allele for a trait and so it is always homozygous and pure breeding. What is a Test Cross and How to Interpret the Results: A test cross is when an individual/parent showing the dominant phenotype is crossed with an individual/parent showing the recessive phenotype (so a parent that is homozygous recessive) to identify whether the individual showing the dominant phenotype has a homozygous genotype, so is pure breeding, or whether it has a heterozygous genotype, so is not pure breeding. See Punnett Squares below. If the parent is homozygous dominant then it will always pass on a dominant allele to its offspring and so all the offspring produced from the test cross will show the same dominant phenotype (refer to Punnett square of test cross 2 above). If the parent is heterozygous, the dominant allele hides/masks a recessive allele that the parent could pass on to its offspring, and so offspring with the recessive phenotype can be produced by the test cross because the offspring are able to inherit a recessive allele from each parent (refer to Punnett square of test cross 1 above). Therefore, if a test cross is carried out and any offspring show the recessive phenotype then the parent showing the dominant phenotype must have a heterozygous genotype and not be pure breeding. Remember: You should always support your explanation of pure breeding and a test cross by including both of the Punnett squares above. Note: When doing a test cross, many offspring have to be produced to be sure of the results/outcome because each fertilisation is an independent random chance event, which is unaffected by previous outcomes, so the chance of an outcome remains the same for every offspring produced. Therefore, if the parent in the test cross is heterozygous, there is a 50% chance for each fertilisation that it will pass on either the dominant or recessive allele and that the offspring will have either the dominant or recessive phenotype (refer to Punnett square of test cross 1). 56. Describe inbreeding 57. Discuss some positive and negative implications of selective breeding e.g. health implications, outbreeding negative traits of endangered species in captive breeding programs Definition: Inbreeding is the production of offspring from the mating or breeding of individuals or organisms that are closely related genetically. Selective Breeding Negative Implications: 1. Inbreeding depression: the loss of fitness and strength of a population which is mainly caused by inbreeding and the inheritance of the same recessive harmful alleles. When the relatives mate, the offspring may inherit two copies of the same recessive harmful allele and suffer the consequences of expressing the harmful allele. 2. Reduced genetic diversity: Only certain individuals with specific alleles are chosen to reproduce. Certain alleles are lost to the population, which removes variation. Natural selection works upon variation, so this may put the survival of the population at risk if a new disease or environmental change occurs. For example, in Kakapo, limited genetic variation means that the population is less adaptable to new diseases or environmental changes. A single disease outbreak could devastate the entire species. 3. Unintended Traits: Focusing on specific traits can inadvertently amplify undesirable ones. For instance, breeding for rapid growth in livestock may result in joint problems or reduced fertility. Selective Breeding Positive Implications 1. Agricultural Advancements: Livestock Improvement: New Zealand’s economy relies heavily on agriculture, particularly sheep, dairy, and beef production. Selective breeding has played a critical role in producing animals with higher yields, such as sheep with finer wool (e.g. merino) and dairy cows with greater milk production. Disease Resistance: Breeding programs for livestock focus on reducing susceptibility to diseases such as facial eczema in sheep, improving herd health and productivity. 2. Conservation of Native Species through captive breeding programs : Maintain genetic diversity: Selective breeding is employed in conservation projects for endangered native species, such as the kākāpō and the takahē. For example, genetic screening in kākāpō breeding programs helps maintain genetic diversity and reduce inbreeding. Eradication of Negative Traits: Captive breeding of endangered species sometimes aims to reduce traits that make them vulnerable in the wild, such as susceptibility to certain diseases or poor reproduction rates. 58.Describe cloning 59.Discuss some positive and negative implications of cloning e.g. De Extinction Definition: Cloning is the process of creating a genetically identical copy of a DNA, cell or an organism. DNA cloning for insulin production DNA cloning is a technique in molecular biology that allows scientists to create multiple, identical copies of a particular DNA segment, such as a gene. A typical cloning experiment involves inserting a DNA fragment into a plasmid—a small, circular piece of DNA—and introducing it into bacteria. The bacteria replicate the plasmid as they grow, producing many copies of the DNA. DNA cloning for insulin involves inserting the insulin gene into a plasmid, which is then introduced into bacteria. These bacteria grow and produce insulin, which is harvested and purified for use by people with Type 1 diabetes. Reproductive cloning Reproductive cloning is defined as the deliberate production of genetically identical individuals. Each newly produced individual is a clone of the original. Clones contain identical sets of genetic material in the nucleus. It involves growing a whole organism from one cell taken from an organism that is being cloned. This provides certainty that the individual will have the existing favourable genes. E.g. livestock with high milk production, efficient growth. It allows an animal with a particular genetic modification to be replicated more rapidly than does natural mating. This side steps meiosis where chance events such as segregation, independent assortment, and recombination during sexual reproduction may risk to interfere with fitness, productivity and overall profitability of offspring. Reproductive cloning starts by taking a body cell from the animal to be cloned and combining its nucleus with an empty egg cell from another animal using an electric current. This fused cell grows into an embryo, which is placed in a surrogate’s uterus. After the pregnancy, the surrogate gives birth to a clone, a genetic copy of the original animal. Implications of cloning - Benefits Help balance ecosystems (reviving endangered species) All species play important roles in their ecosystems. Reviving them can help restore natural balance, benefiting other species and the environment as well as conserving the endangered species. Reviving extinct species Reintroducing extinct species can increase biodiversity and help balance ecosystems Increase livestock output It could increase productivity of meat, milk and wool as well as consistency in quality. By cloning animals with proven resistance to certain diseases, farmers can also reduce the risks of outbreaks. Research Instead of cloning the whole animal, organs can be cloned to study the physiology of certain diseases. It can also be used to develop human drugs and medications Reviving deceased pet Animal cloning can also be used to clone a lost pet Implications of cloning - Concerns and risks Genetic biodiversity A cloned population lacks genetic diversity compared with other populations as they are all identical. If the environmental conditions change, they are less likely to survive, especially the introduction of a new pathogen. Evolution of populations Any population derived from a clone will have little or no genetic diversity. This greatly increases the risk of ‘re-extinction’. Cloning also does not address the problems that put the species in danger in the first place, such as habitat destruction and hunting. Health or survival of individuals If the genome of the cloned individual contains alleles for genetic disorder, all the clones inherit this disorder 60.Describe transgenesis 61.Discuss some implications of transgenesis e.g gene drives, and reduced need for insecticides/herbicides. Definition: Transgenesis is a process of introducing a gene from one organism into the genome of another organism. Transgenesis and Its Purpose The aim is that the resulting transgenic organism will express the gene and exhibit new characteristics. This method is widely used in both agriculture and medicine to create organisms with specific beneficial characteristics that would not occur naturally. Medical transgenesis - e.g. Insulin production Agricultural transgenesis - e.g. Insecticide/Pesticide resistance in crops Insulin production Transgenesis is used in the production of human insulin using genetically modified bacteria. The human insulin gene is inserted into the DNA of E. coli bacteria. The bacteria then produce insulin, which can be harvested and purified for use in treating diabetes. This method provides a reliable, cost-effective way to produce large amounts of insulin, making it more accessible to people with diabetes. Before this method, insulin was extracted from animal pancreases, which was less efficient and could cause allergic reactions. Insecticide/Pesticide resistance in crops Transgenesis is used to create genetically modified crops that are resistant to pests or herbicides. Bt crops are transgenic crops that are genetically engineered from the DNA of bacterium Bacillus thuringiensis For example, Bt crops are genetically engineered to produce a protein from the bacterium Bacillus thuringiensis, which is toxic to certain insects. These crops help farmers reduce the need for chemical insecticides, which are harmful to the environment and non-target species. By producing their own insecticide, the crops can protect themselves from pests, leading to higher yields and less pesticide use. However, over time, pests may develop resistance to the toxin, requiring new strategies to manage pest populations. Implications of Transgenesis - Benefits Medical Benefits: Transgenesis allows for the mass production of medicines like insulin, growth hormones, and vaccines, which can help improve human health and treat diseases. Agricultural Efficiency: GM crops can be designed to grow faster, resist pests, tolerate harsh conditions, and reduce the need for chemical inputs, which can improve food security and reduce environmental harm. Environmental Impact: Reduced pesticide and herbicide use through genetically modified crops can benefit ecosystems by limiting chemical runoff into soil and water. Implications of Transgenesis - Concerns and risks Ecological Risks: Genetically modified organisms (GMOs) could potentially spread their modified genes to wild relatives, disrupting ecosystems. For example, transgenic crops might cross-pollinate with wild plants, creating hybrids with unintended traits. Health and Safety: There are concerns about the long-term health effects of consuming GMOs, though most scientific studies have found GMOs to be safe. Still, public skepticism remains. Ethical Issues: Some people are concerned about "playing God" with nature, especially when it comes to altering animal or plant genomes. There are also ethical questions about patenting genes and organisms. 62.Describe DNA profiling 63.Explain how DNA Profiling is used in Forensics 64.Explain how DNA Profiling is used in establishing Paternity in Takahe Definition: DNA profiling is a technique in which individuals can be identified and compared to others via their genetic markers. DNA profiling (DNA fingerprinting) can be carried out through Gel Electrophoresis. Restriction enzymes are added to the samples which cut the sample DNA at known sequences. The DNA is cut into DNA fragments of various lengths. The DNA samples are added to wells in a gel and a buffer solution is added. Then, electrodes are attached. The DNA molecule is slightly negative so they move towards the positive terminal. Different sized fragments move at a different rate - Smaller fragments move faster, larger fragments move slower. Dye is added to make the bands visible and an x-ray image is produced DNA profiling in Forensics DNA profiling can be used to identify which suspect's DNA matches with a crime scene sample of DNA. A sample must be taken from the crime scene evidence and the suspect. If the crime scene DNA profile matches with the suspect DNA this can be used as evidence that the suspect was at the crime scene. DNA profiling for Paternity Testing in Takahe DNA profiling can be used to identify paternity in endangered species such as the Takahe. A sample must be taken from the whole family group (mother, chicks and father Takahe). The chicks DNA profile has fragments that have been inherited from the mother or father. If a fragment is not present in the mother it therefore must have originated from the father. Therefore paternity can be established. This allows us to learn more about the Takahe mating system to determine if they are socially and genetically monogamous. This knowledge can be in the conservation management plan and used to increase the population size and genetic variation in the species. 65.Describe DNA barcoding 66.Explain how DNA barcoding is used in identification of food fraud 67.Explain how eDNA is used in monitoring invasive pests Definition: DNA barcoding is a method of species identification based on the DNA sequence of short standardised segments of DNA. DNA barcoding is used to identify species or determine relatedness. Individuals in the same species will have the same DNA barcode. Closely related species will have similar DNA barcodes. Unrelated species have very different DNA barcodes. DNA barcoding can be carried out using Polymerase Chain Reaction (PCR). DNA is extracted from the food source or water to be tested. PCR is used to selectively amplify the DNA barcode region, which, in the case of animals, is often the COI gene. The amplified DNA is then sequenced to determine the nucleotide sequence of the barcode region. The obtained DNA barcode sequences are compared to reference libraries such as the CBOL (Consortium for the Barcode of Life) which serve as databases of known species and their corresponding barcode sequences. DNA barcoding in identification of food fraud Food fraud occurs when food is intentionally mislabelled for monetary gain e.g. meat from a patty labelled as beef but contains horse meat or a sample from meat that may contain protected whale meat. DNA barcoding can be used to determine whether the food is labelled correctly. When the obtained DNA barcode sequences are compared to reference libraries, the species in the food can be identified as correctly or incorrectly labelled. DNA barcoding for monitoring invasive species eDNA is environmental DNA (DNA shed by species into the environment). DNA barcoding eDNA can determine what species are present in the area the sample is taken. DNA barcoding eDNA can be used to check ship ballast water for invasive species before it is released from the ship. It can also be used to detect the presence of invasive species in waterways and harbours. When the obtained DNA barcode sequences are compared to reference libraries,invasive species that may be present in the water can be detected.