Lecture 12 Translation II BIOL 23373 Fall 2024 PDF
Document Details
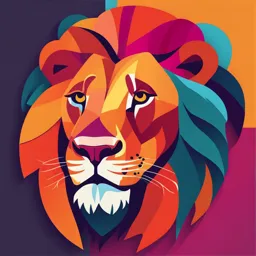
Uploaded by UnconditionalEuropium
University of Arizona
2024
Tags
Related
- Tema 6. Traducción PDF
- General Biology 1 Past Paper 2024-2025 PDF
- BIOL 23373 - General Genetics Lecture 10 - Translation I PDF
- BIOL 23373 General Genetics Fall 2024 Lecture 11 Translation I Lecture Notes PDF
- BIOL 23373 General Genetics Lecture 12 Translation II PDF
- Molecular & Cellular Biology 3rd Year Pharmacy Syllabus 2024-2025 PDF
Summary
This lecture presentation covers the topic of translation II within a general genetics course for undergraduates. It looks at the details of the process and associated elements like amino acids and ribosomes. The content is geared towards further understanding of general genetics.
Full Transcript
BIOL 23373 – General Genetics Fall 2024 Lecture 12 Translation II Announcements Exam 1 was last Friday (Sept. 13). You will be able to view your submission and the answers after the last student completes the makeup exam (Wed. evening). We will di...
BIOL 23373 – General Genetics Fall 2024 Lecture 12 Translation II Announcements Exam 1 was last Friday (Sept. 13). You will be able to view your submission and the answers after the last student completes the makeup exam (Wed. evening). We will discuss class scores (mean, median, SD, distribution, etc.) in class on Friday, Sept. 20. Don’t forget that you get to drop your lowest exam score among the four hour exams and the final exam. If you want to meet (in person or via Zoom), email me 3 or 4 meeting day/time options. Tutoring Tutoring @ the CORD offers 1-on-1 and small group assistance in over 100 U of A courses. Students can meet with tutors in person or online by scheduling an appointment or accessing drop-in services. Learn more about Tutoring services and book an appointment at: https://success.uark.edu/academic-initia tives/tutoring.php Corresponding Readings Chapter sections: 14.1-14.4, 14.8-14.10 Amino Acids are the Fundamental Units of Proteins Proteins are polymers of amino acids Reactive R group side chain 20 options 5 Peptide Bonds Join Amino Acids Peptide bonds link the amino end of one amino acid to the carboxyl end of another 6 Polypeptide Elongation Elongation begins with recruitment of elongation factor (EF) proteins that use energy from GTP 1. Recruit charged tRNAs to the A-site 2. Form peptide bonds between sequential amino acids 3. Translocate the ribosome in the 3 direction along the mRNA 7 Polypeptide Elongation in Bacteria Several different EFs help carry out elongation in a series of steps Charged tRNAs affiliated with an EF inspect the open A site; the tRNA with the correct anticodon sequence enters the A site 8 Polypeptide Elongation in Bacteria cont. Peptidyl transferase catalyzes formation of peptide bonds between amino acids at the P and A sites The polypeptide is transferred to the tRNA at the A site while the tRNA from the P-site exits through the E site 9 Polypeptide Elongation in Bacteria cont. EFs next translocate the ribosome along the mRNA, moving it three nucleotides in the 3 direction This moves the tRNA at the A site to the P site and opens the A site for the tRNA specified by the next codon 10 Polypeptide Elongation in Eukaryotes Distinct elongation factors carry out elongation in eukaryotes; steps are similar to those of bacteria Charged tRNAs inspect the A site, but only the one with the anticodon sequence that is complementary and antiparallel to the codon sequence can bind the A site 11 Polypeptide Elongation in Eukaryotes cont. Peptidyl transferase catalyzes formation of a peptide bond between sequential amino acids This transfers the polypeptide chain from the tRNA in the P site to the tRNA in the A site 12 Polypeptide Elongation in Eukaryotes cont. Ribosome is translocated along the mRNA to the next codon This moves the tRNA at the A site (and the attached polypeptide) to the P site and opens the A site for the next tRNA 13 Translation Termination Elongation cycle continues until one of the three stop codons (UAA, UAG, UGA) enters the A site of the ribosome Bacteria and eukaryotes both use release factors (RF) to bind a stop codon in the A site The polypeptide bound to the tRNA at the P site is released when the GTP attached to the RF is hydrolyzed 14 Release Factors In bacteria, release factor RF1 recognizes UAG and UAA while RF2 recognizes UAA and UGA Eukaryotic termination is accomplished by a single release factor called eRF, which recognizes all three stop codons 15 Translation in Action https://www.youtube.com/watch?v=8Hsz_Vmcy-Y Translation Is Fast and Efficient Translation is an active and ongoing process that is highly efficient Each bacterial cell contains about 20,000 ribosomes, collectively accounting for ~25% of the cell’s mass In eukaryotes, mRNAs are produced in the nucleus and must be processed to form mature mRNAs and then exported to the cytoplasm for translation In bacteria, the coupling of transcription and translation allows ribosomes to begin translating mRNAs before they are completed 17 Bacterial Polyribosomes Electron micrographs reveal structures in bacteria called polyribosomes: groups of ribosomes actively translating the same mRNA 18 Translation of Bacterial Polycistronic mRNA Each polypeptide-producing gene in eukaryotes produces monocistronic mRNA: an mRNA that directs synthesis of a single polypeptide Groups of bacterial genes called operons share a single promoter and produce polycistronic mRNAs Polycistronic mRNAs have multiple polypeptide- producing segments and direct synthesis of several different polypeptides Genes of operons are regulated as a unit and proteins function in the same metabolic pathway 19 Polycistronic mRNA Multiple polypeptide-producing segments of polycistronic mRNAs have a Shine-Dalgarno site for ribosome binding and initiation of translation, and are separated by intercistronic spacer sequence, which is not translated 20 Translation Is Followed by Polypeptide Processing and Protein Sorting Production of functional proteins from polypeptides is often not complete until polypeptides are chemically modified and transported to their final destinations, which may be inside or outside the cell Two categories of post-translational events modify and transport polypeptides 1. Post-translational polypeptide processing 2. Protein sorting 21 Polypeptide Processing Post-translational polypeptide processing modifies polypeptides into functional proteins by removal or chemical alteration of amino acids e.g., remove Met e.g., add -CH , -OH, -COCH 3 3 Phosphorylation: kinases attach phosphate groups to hydroxyl groups, phosphatases remove them—changes charge and thus shape of protein 22 Polypeptide Processing cont. Polypeptides may be cleaved into multiple segments that have separate functions or that aggregate to form a functional protein The hormone insulin is first produced as preproinsulin, from which the “pre-amino” segment at the N-terminus is cleaved to produce proinsulin Proinsulin is cleaved again to produce insulin, a functional protein consisting of two separate chains held together by disulfide bonds 23 Protein Sorting Protein sorting uses signal sequences to direct proteins to their cellular destinations First 15-20 amino acids on N-terminus used by endoplasmic reticulum and Golgi apparatus to determine where to transport protein 24 Role of the Golgi Apparatus in Protein Sorting In the Golgi apparatus glycosylation (addition of carbohydrate side chains) of proteins determines their ultimate locations based on which receptors recognize the modified polypeptide Receptor binding causes particular proteins to be included in vesicles that bud off from the Golgi The vesicles then move to their cellular destinations 25 Protein Structure Following translation & processing, polypeptides fold up and assume higher order structures, and may interact with other polypeptides. There are four levels of structure in proteins: 1. Primary 2. Secondary 3. Tertiary 4. Quaternary 26 Protein Structure Primary structure: the sequence of amino acids comprising the polypeptide O O– OH C SH H3C CH3 H H O CH3 O CH2 O CH2 O CH2 O CH O CH2 O CH2 O H N+ C C N C C N C C N C C N C C N C C N C C N C C O– H H H H H H H H H H H H H H H H Free carboxyl Free amino group group 1 2 3 4 5 6 7 8 H3N+ COO– Gly Ala Ser Asp Phe Val Tyr Cys N-terminus C-terminus 27 Protein Structure Secondary structure: polypeptide chain folds into localized, repeating shapes based on bonds formed between nearby amino acids R side chains extend outward α helix β-pleated sheet 28 Protein Structure Tertiary structure: short regions of secondary structure fold into a three-dimensional shape determined by hydrophobic and ionic interactions as well as H bonds and Van der Waals interactions This is the final conformation of proteins composed of a single polypeptide. 3-D model of lysozyme 29 Protein Structure Quaternary structure: 2 or more polypeptides associate with one another (weak bonds) to make a functional protein Hemoglobin A 30 Protein Stability Factors that promote protein folding and stability: 1. Hydrogen bonds 2. Ionic bonds and other polar interactions 3. Hydrophobic effects 4. Van der Waals forces Copyright © The McGraw-Hill Companies, Inc. Permission required for reproduction or display. 5. Disulfide bridges NH3+ 2 Ionic bond: Bonds form Hydrogen bonds: Bonds form O 1 between oppositely between atoms in the polypeptide CH2 C charged side chains. + backbone and between atoms in O– NH3 different side chains. CH2 CH2 CH2 H O CH2 H O COO– CH 3 HC CH3 CH CH 2 H CH2 O 2 CH3 2 CH CH2 OH H N CH2 3 Hydrophobic effect: CH 3 CH O Nonpolar amino 3 CH H acids in the center NH2 C CH2 of the protein avoid N contact with water. CH 3 CH 2 CH 3 H CH 3 OH CH2 CH CH 3 CH 2 CH 3 CH CH2 S S CH2 CH 3 van der Waals 5 Disulfide bridge: 4 forces: Attractive A covalent bond forces occur forms between 2 between atoms cysteine side chains. that are optimal distances apart. 31 Structure = Function Proteins have many roles: Structural, contractile, transport, hormones, receptors, histones, transcription factors, enzymes, etc. Glucose Correct folding and structure are ATP critical for proper function Active site Mutations can cause defects in Hexokinase proteins by changing the amino acid sequence, which affects protein folding and stability Incorrectly folded proteins do not function normally 32 Chaperones and Protein Folding Within the endoplasmic reticulum, incorrectly folded proteins are bound by other proteins called chaperones that help them fold correctly Once a protein is folded correctly, the chaperone releases the protein Proteins that cannot fold properly are irreversibly bound to chaperones These chaperone-protein complexes are sequestered and destroyed Some diseases result from failures in this process 33 Disorders Associated with Incorrect Protein Folding Some diseases result when large amounts of mutant proteins accumulate without being destroyed They are often neurodegenerative disorders and dementias such as Alzheimer disease, Parkinson disease, and Huntington disease Prion diseases, such as mad cow disease (cattle), chronic wasting disease (deer, elk), and Creutzfeldt- Jakob disease (human), are also due to misfolded proteins, which are transmissible. 34 X