Chapter 19: Heart Lecture Outline PDF
Document Details
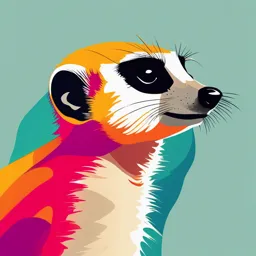
Uploaded by FlourishingWilliamsite1339
Tags
Related
- PDF (004) Cardiovascular Physiology: Heart as a Pump (University of Northern Philippines)
- Cardiovascular Physiology: Heart as a Pump PDF
- Physiology LC12 Cardiac Muscle - The Heart as a Pump - PDF
- Anatomy & Physiology Chapter 17 Part A: The Cardiovascular System: The Heart PDF
- Fisiología Humana I: Corazón PDF
- Chapter 18 The Cardiovascular System: The Heart PDF
Summary
This document outlines the structure and function of the heart, exploring topics such as cardiac muscle, the heart's conduction system, and the cardiac cycle. It delves into the microscopic structure of cardiac tissue, intercellular structures, and the metabolism of cardiac muscle. Additionally, the lecture outline covers the innervation of the heart, stimulation of the heart, and electrocardiograms (ECG).
Full Transcript
Chapter 19: Heart Lecture Outline Describe the general structure and function of the cardiac muscle including how its energy needs are met. Describe in detail the components and the processes associated with the heart’s conduction system, including autonomic control....
Chapter 19: Heart Lecture Outline Describe the general structure and function of the cardiac muscle including how its energy needs are met. Describe in detail the components and the processes associated with the heart’s conduction system, including autonomic control. Explain the events of cardiac muscle contraction, including refractory period and significance of the plateau phase. Describe and identify the components of the ECG reading and discuss the clinical significance. Explain in detail the cardiac cycle, its stages, and factors that influence the cycle. 19.4a Microscopic Structure of Cardiac Muscle Myocardium is composed of cardiac muscle tissue Cardiac muscle cells are short, branched House one or two central nuclei Supported by areolar connective tissue, called endomysium Sarcolemma (plasma membrane) Invaginates to form T-tubules extending into sarcoplasmic reticulum Sarcoplasmic reticulum Surrounds bundles of myofilaments Myofilaments arranged in sarcomeres Organization gives rise to striated appearance under microscope Optimal length (maximum overlap of filaments) is seen when heart is stretched by blood filling the chamber Allows filaments to contract with greater force with more blood Intercellular structures Sarcolemma is folded at connections between cells Increases structural stability of myocardium Facilitates communication between cells Cells are connected with intercalated discs Desmosomes mechanically join cells with protein filaments Gap junctions electrically join cells (allow ion flow) to make each heart chamber a functional unit (functional syncytium) Cross Section of Cardiac Muscle Cells (Figure 19.12a,b) Longitudinal View of Cardiac Muscle Cell (Figure 19.12c,d) Metabolism of cardiac muscle High demand for energy Extensive blood supply Numerous mitochondria Myoglobin and creatine kinase Able to use different types of fuel molecules Fatty acids, glucose, lactic acid, amino acids, and ketone bodies Relies mostly on aerobic metabolism Makes it susceptible to failure when ischemic (oxygen is low) Interference with blood flow to heart muscle can cause cell death 19.5a The Heart’s Conduction System Conduction system: initiates and conducts electrical events to ensure proper timing of contractions Specialized cardiac muscle cells that have action potentials but do not contract Its activity is influenced by autonomic nervous system Sinoatrial (SA) node initiates heartbeat (pacemaker) Located high in posterior wall of right atrium Atrioventricular (AV) node Located in floor of right atrium (near right AV valve) Atrioventricular (AV) bundle (bundle of His) Extends from AV node though interventricular septum Divides into left and right bundles Purkinje fibers Extend from left and right bundles at heart’s apex Couse through walls of ventricles Conduction System (Figure 19.13a) 19.5b Innervation of the Heart Cardiac center of medulla oblongata Contains cardioacceleratory and cardioinhibitory centers Receives signals from baroreceptors and chemoreceptors in cardiovascular system Sends signals via sympathetic and parasympathetic pathways Modifies (does not initiate) cardiac activity Influences rate and force of heart’s contractions Parasympathetic innervation decreases heart rate Starts at medulla’s cardioinhibitory center Relayed via vagus nerves (CN X) Right vagus innervates SA node Left vagus innervates AV node Sympathetic innervation increases heart rate and force of contraction Starts at medulla’s cardioacceleratory center Relayed via neurons from T1–T5 segments of spinal cord Extend to SA node, AV node, myocardium, and coronary arteries Increases coronary vessel dilation Innervation of the Heartv (Figure 19.13b) 19.6 Stimulation of the Heart Heart contraction involves two events The conduction system initiates and propagates an action potential Cardiac muscle cells initiate action potentials and contract This process happens first in the atria and then the ventricles Physiologic Processes Associated with Heart Contraction: Conduction System (Fig19.14a) Physiologic Processes Associated with Heart Contraction: Cardiac Muscle Cells (Fig19.14b) Clinical View: Ectopic Pacemaker Ectopic pacemaker: Pacemaker other than SA node Conduction system cells other than SA node Also have ability to spontaneously depolarize Depolarize at slower rates than SA node AV node the default pacemaker if SA node impaired AV node with inherent rhythm of 40 to 50 beats/min Fast enough to sustain life Cardiac muscle with rate of 20 to 40 beats/min Usually too slow to sustain life 19.7a Cardiac Muscle Cells at Rest Cardiac muscle cells Contain Na+/ K+ pumps, Ca2+ pumps, leakage channels for Na+ and K+ Have a resting membrane potential of -90 mV Contain specific voltage-gated channels: Fast voltage-gated Na+ channels Slow voltage-gated Ca2+ channels Voltage-gated K+ channels Voltage channels are closed when cell is at rest Cardiac Muscle Cell at Rest (Figure 19.18) 19.7b Electrical and Mechanical Events of Cardiac Muscle Cells Electrical events of cardiac muscle action potential 1) Depolarization Impulse from conduction system (or gap junctions) opens fast voltage-gated Na+ channels Na+ enters cell changing membrane potential from −90 mV to +30 mV Voltage-gated Na+ channels start to inactivate 2) Plateau Depolarization opens voltage-gated K+ and slow voltage-gated Ca2+ channels K+ leaves cardiac muscle cell as Ca2+ enters Stimulates sarcoplasmic reticulum to release more Ca2+ Membrane remains depolarized 3) Repolarization Voltage-gated Ca2+ channels close while K+ channels remain open Membrane potential goes back to −90 mV Electrical Events at the Sarcolemma of a Cardiac Muscle Cell (Figure 19.19) Mechanical events (crossbridge cycling) Ca2+ enters sarcoplasm from interstitial fluid and SR leading to contraction As in skeletal muscle, it binds to troponin and initiates crossbridge cycling Crossbridge formation, power stroke, release of myosin head, reset of myosin head Ca2+ levels decrease leading to relaxation Channels close and pumps move it into SR and out of cell 19.7c Repolarization and the Refractory Period Cardiac muscle cannot exhibit tetany Unlike skeletal muscle, cardiac cells have a long refractory period Cell cannot fire a new impulse during refractory period Cardiac muscle cell’s plateau phase leads to refractory period of about 250 ms The heart cell contracts and relaxes before it can be stimulated again Makes sustained (tetanic) contraction impossible Electrical and Mechanical Events in Skeletal Muscle Cells (Figure 19.20a,b) Electrical and Mechanical Events in Cardiac Muscle Cells (Figure 19.20c,d) 19.7d Electrocardiogram (ECG) Electrocardiogram (ECG/EKG) Skin electrodes detect electrical signals of cardiac muscle cells Common diagnostic tool Waves P wave - Reflects electrical changes of atrial depolarization originating in SA node QRS complex Electrical changes associated with ventricular depolarization Atria also simultaneously repolarizing T wave - Electrical change associated with ventricular repolarization Segments Two segments between waves correspond to plateau phases of cardiac action potentials (no electrical change) P-Q segment Associated with atrial cells’ plateau (atria are contracting) S-T segment Associated with ventricular plateau (ventricles are contracting) The Electrocardiogram (Figure 19.21a) The Electrocardiogram 2 (Figure 19.21b) Electrical events of the heart and an ECG Atria Atrial depolarization: recorded as the P wave, muscle cells of atria stimulated to contract Atrial plaeau: recorded as PQ segment, muscle cells of atria contract and relax Atrial repolarization: not visible on ECG Ventricles Ventricular depolarization: recorded as the QRS wave, muscle cells of ventricles stimulated to contract Ventricular plateau: recorded as ST segment, muscle cells of ventricles contract and relax Ventricular repolarization: recorded as T wave Electrical Events of the Heart and an ECG (Figure 19.22) Clinical View: Cardiac Arrhythmia Any abnormality in heart’s electrical activity Heart blocks: impaired conduction May result in light-headedness, fainting, irregular heartbeat, chest palpitations First-degree AV block: PR prolongation Slow conduction between atria and ventricles Second-degree AV block Failure of some atrial action potentials to reach ventricles Third-degree AV block: complete Failure of all action potentials to reach ventricles Premature ventricular contractions Result from stress, stimulants, or sleep deprivation Abnormal action potential within AV node or ventricles Not detrimental unless they occur in large numbers Atrial fibrillation: chaotic timing of atrial action potentials Ventricular fibrillation: chaotic electrical activity in ventricles Uncoordinated contraction and pump failure Leads to death of heart cells Treated with paddle electrode defibrillator or automated external defibrillator (AED) 19.8a Overview of the Cardiac Cycle Cardiac cycle: all events in heart from the start of one heart beat to start of the next Includes both systole (contraction) and diastole (relaxation) Contraction increases pressure; relaxation decreases it Blood moves down its pressure gradient (high to low) Valves ensure that flow is forward (closure prevents backflow) Ventricular activity is most important driving force Ventricular contraction raises ventricular pressure AV valves pushed closed Semilunar valves pushed open and blood ejected to artery Ventricular relaxation lowers ventricular pressure Semilunar valves close No pressure from below keeping them open AV valves open No pressure pushing them closed 19.8b Events of the Cardiac Cycle See Figure 19.23a-c and Figure 19.23d-f: Phases of the Cardiac Cycle As the cardiac cycle begins Four chambers at rest Blood returning to both atria Passive filling of ventricles AV valves open Atrial pressure > ventricular pressure Semilunar valves closed Pressure in ventricles < arterial trunk pressure Atrial contraction and ventricular filling SA node starts atrial excitation Atria contract pushing remaining blood into ventricles Ventricles filled to end-diastolic volume (EDV) Atria relax for remainder of cardiac cycle Isovolumic contraction Purkinje fibers initiate ventricular excitation Ventricles contract, pressure rises, and AV valves are pushed closed Ventricular pressure is still less than arterial trunk pressure, so semilunar valves still closed Ventricular ejection Ventricles continue to contract so that ventricular pressure rises above arterial pressure Semilunar valves forced open as blood moves from ventricles to arterial trunks Stroke volume (SV) is amount of blood ejected by ventricle End systolic volume (ESV) is amount of blood remaining in ventricle after contraction finishes ESV = EDV − SV For example, 60 mL = 130 mL − 70 mL Isovolumic relaxation Ventricles relax and start to expand, lowering pressure Arterial pressure greater than ventricular pressure By sliding back toward ventricles, blood closes semilunar valves AV valves remain closed When all valves are closed, blood neither enters nor leaves and the time is called “isovolumic” Atrial relaxation and ventricular filling All heart chambers are relaxed Atrial blood pressure forces AV valves open and blood flows into ventricles Semilunar valves remain closed since arterial pressure is greater than ventricular pressure Ventricular balance Equal amounts of blood are pumped by left and right sides of the heart Left heart pumps blood farther and so must be stronger (thicker) But ejected blood volumes must be the same or edema (swelling) can occur 19.9a Introduction to Cardiac Output Cardiac output (CO) Amount of blood pumped by a single ventricle in one minute Measured in liters per minute Measure of effectiveness of cardiovascular system Increases in healthy individuals during exercise Determined by heart rate (beats per minute) and stroke volume (amount of blood ejected per beat) HR × SV = CO For example, 75 beats/min × 70 ml/beat = 5.25 L/min Maintaining resting cardiac output CO must meet tissue needs Individuals with smaller hearts have smaller stroke volume and so must have faster heart rate For example, women and children Individuals with stronger hearts have larger stroke volume and slower heart rate For example, Endurance athletes have thicker heart walls and stronger hearts Cardiac reserve Capacity to increase cardiac output above rest level Subtract cardiac output at rest from output with exercise HR accelerates and stroke volume increases during exercise Gives measure of level of exercise an individual can pursue For example, CO can increase four-fold in healthy, nonathlete and up to seven-fold in athlete 19.9b Variables That Influence Heart Rate Chronotropic agents change heart rate Alter the activity of nodal cells (SA and/or AV node) Often work via autonomic nervous system or hormones Positive chronotropic agents increase heart rate Sympathetic nerve stimulation Causes norepinephrine (NE) release on heart Causes adrenal to release epinephrine (EPI) and NE NE and EPI bind to nodal cells and increase their firing rate Receptors are beta-one adrenergic receptors G-protein, adenylate cyclase, cAMP, protein kinase cascade Phosphorylated Ca2+ channels enhance Ca2+ influx so cell fires sooner Sympathetic Innervation of SA Nodal Cells (Figure 19.25) Thyroid hormone Increases number of β1-adrenergic receptors on nodal cells Caffeine Inhibits breakdown of cAMP Nicotine Increases release of norepinephrine Cocaine Inhibits reuptake of norepinephrine (more remains in cleft longer) Negative chronotropic agents decrease heart rate Parasympathetic activity Parasympathetic axons release acetylcholine (ACh) onto nodal cells ACh binds muscarinic receptors which are K+ channels Channels open, K+ exits cell making it more negative Longer time for nodal cell to reach threshold, so heart rate slower Beta-blocker drugs Interfere with EPI and NE binding to beta receptors Used to treat high blood pressure Autonomic reflexes Baroreceptors and chemoreceptors send signals to cardiac center Cardiac center influences sympathetic and parasympathetic systems to alter cardiac output as needed Atrial reflex (Bainbridge reflex) Protects heart from overfilling Baroreceptors in atrial walls stimulated by increased venous return Increased nerve signals to cardioacceleratory center Increased excitation of sympathetic axons to heart Heart rate increase to move blood through quickly (less atrial stretch) 19.9c Variables That Influence Stroke Volume Stroke volume is amount of blood ejected in one beat Stroke volume (SV) is influenced by: venous return, inotropic agents, and afterload Venous return: volume of blood returned to the heart Directly related to stroke volume Determines amount of ventricular blood prior to contraction That is, end-diastolic volume (EDV) Volume determines preload Pressure stretching heart wall before shortening Venous return may be increased by increased venous pressure or increased time to fill Venous pressure increases during exercise as muscles squeeze veins Time to fill increases with slower heart rate (for example in high-caliber athletes with strong hearts) Decreases with low blood volume (for example, with hemorrhage) or high heart rate Results in smaller end diastolic volume, preload Results in smaller stroke volume Balanced ventricular output due largely to Frank-Starling law As one side receives increased blood volume it contracts more forcefully Leads to greater fill (and more forceful contraction) on the other side Variables That Influence Stroke Volume: Venous Return (Figure 19.28a) Inotropic agents change stroke volume Alter contractility (force of contraction) Generally due to a changes in Ca2+ available in sarcoplasm Ca2+ levels directly relate to number of cross bridges formed Positive inotropic agents increase available Ca2+ EPI and NE work via β1-adrenergic receptors to increase Ca2+ Thyroid hormone increases number of β1 receptors Certain drugs, for example, digitalis, boost cardiac output by increasing contractility Negative inotropic agents decrease available Ca2+ and thus lower contractility Electrolyte imbalances such as increased K+ or H+ Certain drugs, for example, Ca2+ channel-blocking blood pressure drugs Variables That Influence Stroke Volume: Inotropic Agents (Figure 19.28b) Afterload Resistance in arteries to ejection of blood by ventricles Pressure that must be exceeded before blood ejected Atherosclerosis (plaque in vessel linings) increases afterload Seen with aging Smaller arterial lumen exerts greater resistance to movement of blood Results in decreased stroke volume 19.9d Variables That Influence Cardiac Output Cardiac output varies directly with heart rate and stroke volume Heart rate depends on chronotropic agents Influence SA node to change its firing rate Influence AV node to alter amount of delay Stroke volume Generally depends on state of myocardium Venous return alters stretch of heart Inotropic agents influence crossbridge formation Increased afterload (arteries) decreases stroke volume Relevant in elderly individuals Factors Affecting Cardiac Output (Figure 19.29)