BCM 201 General Biochemistry I Lecture Notes PDF
Document Details
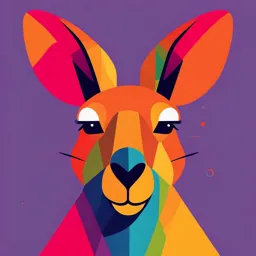
Uploaded by TranquilObsidian5543
University of Calabar
Tags
Summary
These lecture notes cover the fundamental chemistry, properties, and functions of amino acids and proteins, including their roles in biological processes. The notes also discuss different types of amino acids and their structures, as well as common reactions of amino acids.
Full Transcript
**Lecture Notes on BCM 201 (General Biochemistry I)** **Topic Amino Acids/Protein: Chemistry, Properties, Functions, Reactions, isolation, purification and identification** **Introduction to Amino Acids** Proteins are complex molecules composed of amino acids, which serve as their fundamental bui...
**Lecture Notes on BCM 201 (General Biochemistry I)** **Topic Amino Acids/Protein: Chemistry, Properties, Functions, Reactions, isolation, purification and identification** **Introduction to Amino Acids** Proteins are complex molecules composed of amino acids, which serve as their fundamental building blocks. Present in all living organisms, proteins perform diverse functions, including structural support, regulation, contraction, protection, transport, storage, membrane integration, enzymatic activity, and toxin formation. They play essential roles in various life processes, such as metabolism, movement, immune defense, cellular communication, and molecular recognition. The building blocks of proteins are amino acids. Individually, these amino acids too have diverse roles in metabolism, signaling, and as precursors for biomolecules. **How many amino acids form proteins** In all known life, 22 amino acids are genetically encoded (proteinogenic). Of these, 20 are part of the standard genetic code, while two additional amino acids, selenocysteine and pyrrolysine, are incorporated through specialized translation mechanisms; The standard twenty amino acids are as follows: 1. Glycine (gly) 2. Alanine (Ala) 3. Valine (Val) 4. Leucine (Leu) 5. Isoleucine (ile) 6. Proline (Pro) 7. Glutamate (Glu) 8. Glutamine (Gln) 9. Aspartate (Asp) 10. Asparagine (Asn) 11. Lysine (Lys) 12. Histidine (His) 13. Arginine (Arg) 14. Phenylalanine (Phe) 15. Tryptophan (Trp) 16. Tyrosine (Tyr) 17. Serine (Ser) 18. Cysteine (Cys) 19. Threonine (Thr) 20. Methionine (Met) **1.3 Common Structural features of amino acids** - **Core Structure**: - Amino group (-NH₂) - Carboxyl group (-COOH) - Side chain (R-group) - Hydrogen atom - All attached to a central (α) carbon. The above general structural formula is common to all amino acids with each amino acids only differing in their R groups. However, only **PROLINE** doesn't obey this common structural formular. why? This is because the alpha amino group of Proline is fused in a cyclic ring structure with its R-group, unlike other amino acids with a stand-alone alpha amino group. Proline is therefore also known as **IMINO** acids. It must also be noted that due to the presence of a central carbon bonded to four different groups, amino acids are said to exhibit a phenomenon called; **CHIRALITY.** Therefore, all amino acids have a chiral carbon except **GLYCINE.** Glycine has hydrogen as its side chain; therefore it doesn't have four different groups attached to the central carbon. Due to their chirality, amino acids two possible stereoisomers. (L-isomer and D-isomer). The L- denotes **LEVOROTATORY**; which means it rotates plane polarized light to the left, while D- denotes **DEXTROROTATORY:** which means it rotates plane polarized light to the right. Both D and L isomers are collectively known as **ENANTIOMERS.** **NOTE:** All amino acid residues are in the **L-stereoisomer.** However when chiral compounds are formed by ordinary chemical reactions, they yield **RACEMIC** mixture which can be converted into a single enantiomer by enzymes known as **RACEMASE.** **Amino acids can be classified into five groups based on the properties of their R groups** Knowing the chemical properties of amino acids is very important to understanding their functions. The different R-groups of amino acids determine their properties in the proteins they predominate. for example, the amino acid Histatin is a cationic antimicrobial peptide because it is rich in the positively charged amino acids (Histidine). Below are the different classes of amino acids and the different amino acids belonging to the different classes. : - **Nonpolar aliphatic amino acids** These amino acids have nonpolar and hydrophobic R groups. Examples include glycine, alanine, valine, leucine, isoleucine, methionine, and proline. - **Aromatic amino acids** These amino acids have aromatic side chains and are relatively nonpolar. Examples include phenylalanine, tyrosine, and tryptophan. - **Polar, uncharged amino acids** These amino acids have R groups that are more soluble in water than nonpolar amino acids. Examples include serine, threonine, cysteine, asparagine, and glutamine. - **Acidic amino acids** These amino acids have acidic or negatively charged R groups. Examples include glutamic acid and aspartic acid. **Negatively charged amino acids** - **Basic amino acids** These amino acids have basic or positively charged R groups. Examples include lysine, arginine, and Histidine. Basic amino acids are also called **positively charged amino acids** **Uncommon amino acids ** These amino acids are not naturally encoded in the genetic code but may arise in plants or bacteria through post-translational modifications. They can also be chemically synthesized and used as pharmacological tools in drug development. Uncommon amino acids are structurally similar to the 20 standard amino acids used in protein synthesis but are less prevalent in nature. Despite their rarity, they play crucial roles in various biological processes and are of significant interest in fields such as biochemistry and molecular biology. ### Examples of Uncommon Amino Acids: - **Hydroxylysine and Hydroxyproline**\ Found in collagen, a fibrous protein, these amino acids require vitamin C for their conversion to hydroxy forms, which are essential for collagen stability. - **Selenocysteine (Sec) and Pyrrolysine (Pyl)**\ These amino acids are considered the 21st and 22nd in the genetic code. They are encoded by the UGA and UAG codons, typically stop signals, but are reassigned in specific translation mechanisms. C:\\Users\\toshiba\\Downloads\\Capture amino acids.JPG **Figure 1**: CLASSES OF AMINO ACIDS **AMINO ACIDS ARE CLASSIFIED AS ESSENTIAL and NON-ESSENTIAL DEPENDING ON THE ABILITY OF THE BODY TO SYNTHESIZE THEM** It is not uncommon to hear students define essential amino acids as those that are important while non-essential are not important. This definition is not correct as far as biochemistry is concerned. These amino acids are merely classified on the basis of their dispensability in diet due to the ability to produce them endogenously. All amino acids that can be produced by the body are dispensable in diet. I.e. it is not essential to be consumed via diet. This however doesn't in anyway imply that they should never be taken through diet rather it means; not taking in diet would not result in any deficiency since the body can produce them. Nonessential amino acids on the other hand are those the body lacks the capability to synthesize therefore it is essential they are consumed in our diet. **Essential** include; Phenyalanine, Valine, Tryptophan, Threonine Isoleucine Methionine, Histidine Arginine, Leucine and Lysine **(PVT TIM haLL).** **Note**: Some amino acids, like arginine and histidine, may be conditionally essential during certain periods of growth, such as pregnancy, adolescence, or recovery from trauma **Non-essential amino acids**: Gluamate, Glutamine, Aspartate, Asparagine, Glycine, Alanine, Serine, Cysteine, Proline, Tyrosine **1.5 AMINO ACIDS HAVE DUAL ROLES AS ACIDS AND BASES** Amino acids are amphoteric, meaning they can function as both acids and bases depending on the pH of their surroundings. This property arises from their structure, which includes an acidic carboxyl group (-COOH) and a basic amino group (-NH₂). ### 1.6 Acid-Base Properties of Amino Acids: - **Zwitterions**\ At physiological pH (approximately 7--7.4), amino acids exist as zwitterions---dipolar ions with equal positive and negative charges. - **Isoelectric Point (pI)**\ The isoelectric point is the specific pH at which an amino acid exists in its zwitterionic form, with no net electrical charge. - **Behavior in Acidic Conditions**\ In acidic environments, the carboxylate group (-COO⁻) gains a proton, resulting in a positively charged amino acid. - **Behavior in Basic Conditions**\ In basic environments, the amino group (-NH₃⁺) loses a proton, resulting in a negatively charged amino acid. - **Biological Significance**\ The acid-base properties of amino acids are essential for critical biological processes, including protein folding, peptide bond formation, and enzyme activity regulation. Note; Amino acids can exist in 3 state based on net charge. At isoelectric point (net charge:'0), at low ph (+1) at high ph (-1). A few amino acids can however exist as +2 or -2 due to the presence of additional amino or carboxyl groups. Glu and Asp are the only two amino acids that can exist as -2 while Histidine, Lysine and arginine are the only ones that an exist in +2 states. **Physical and Chemical Properties** **Physical Properties** 1. **Optical Activity**: - Most amino acids are chiral (except glycine) and exist as L- and D-isomers. - Proteins use L-isomers. 2. **Solubility**: - Polar amino acids are soluble in water. - Nonpolar amino acids are less soluble in water. 3. **Melting Point**: - High melting points due to ionic interactions in the solid state. **Reactions of Amino Acids** **3.1 Reactions with Functional Groups** 1. **With Ninhydrin**: - Forms a purple complex with primary amino acids; used in qualitative analysis. (will be discussed later in the latter parts of the note) 2. **With Carboxylic Acids**: - Peptide bond formation through condensation. 3. **Oxidation of Cysteine**: - Cysteine residues oxidize to form disulfide bonds (cystine). 4. **Decarboxylation**: - Produces amines (e.g., histamine from histidine). 5. **Deamination**: - Removal of the amino group to form α-keto acids. **Special Reactions** 1. **Peptide Bond Formation**: - Catalyzed by enzymes during protein synthesis. - Amide linkage between the α-carboxyl group of one amino acid and the α-amino group of another. 2. **Transamination**: - Transfer of an amino group to α-ketoglutarate to form glutamate. 3. **Maillard Reaction**: - Non-enzymatic browning reaction between amino acids and reducing sugars. 4. **Post-Translational Modifications**: - Phosphorylation, methylation, acetylation, glycosylation modify amino acid side chains in proteins. **Biological Significance of Amino Acids** Amino acids perform numerous essential biological functions, including: 1. **Protein Synthesis**: Amino acids are the fundamental building blocks of proteins, which are crucial for growth, development, and maintaining living systems. The sequence of amino acids determines each protein\'s specific function. 2. **Metabolism**: They participate in biosynthetic reactions, contributing to the production of nucleotide bases, hormones, and neurotransmitters. 3. **Body Growth and Repair**: Amino acids play a vital role in promoting growth and facilitating tissue repair. 4. **Osmotic Pressure Regulation**: They help maintain osmotic pressure stability within cells and tissues. 5. **Neurotransmission**: Amino acids are key components in neurotransmission, acting as precursors or direct signaling molecules. 6. **Chemical Messengers**: Certain amino acids function as chemical messengers, facilitating communication between cells. 7. **Skin, Hair, and Nail Health**: Amino acids like methionine and cysteine contribute to the strength and flexibility of skin, hair, and nails. 8. **Immune Support**: They aid in strengthening the immune system, supporting the body's defense mechanisms. 9. **Digestive Health**: Amino acids play a role in sustaining the normal functioning of the digestive system. 10. **Buffering roles**: Due to their amphoteric nature, amino acids act as physiological buffers -. **Chemistry of Peptides and proteins** **The early parts of this notes has discussed amino acids. Now we discuss proteins.** Peptides, oligopeptides, and polypeptides are all chains of amino acids linked by peptide bonds, but the main difference between them is their length: - **Peptides** Short chains of amino acids, typically 2--50 amino acids long - **Oligopeptides** Peptides with fewer than 20 amino acids, such as dipeptides, tripeptides, and tetrapeptides - **Polypeptides** Longer, unbranched chains of amino acids, typically 50 or more amino acids long - **Proteins** One or more polypeptides joined together, and are essentially very large peptides Peptide bonds are formed through a dehydration synthesis reaction, where the amino group of one amino acid and the carboxyl group of another amino acid combine to form a water molecule and a peptide bond **Note: though, polypeptides and proteins are used interchangeably, they actually are different. Polypeptides have molecular weights below 10,000 while proteins have larger molecular weights.**. NOTE: proteins could either exist as a single polypeptide chain or exist have two or more polypeptides chains. These are called multisubunit proteins.The following are important terms related to multisubunit proteins #### **1. Oligomeric Proteins** - :\ Oligomeric proteins are multimeric proteins composed of two or more polypeptide chains (called subunits) that are held together by non-covalent interactions or, less commonly, covalent bonds. These subunits may be identical (homooligomers) or different (heterooligomers). - The quaternary structure of oligomeric proteins defines the spatial arrangement of these subunits. - Subunits often work cooperatively or allosterically, influencing each other\'s activity. - **Specific Examples**: - **Hemoglobin**: A heterotetramer composed of two α and two β subunits; involved in oxygen transport in the blood. - **Insulin**: A heterodimer composed of an A-chain and a B-chain linked by disulfide bonds. - **Lactate Dehydrogenase (LDH)**: A homotetramer involved in the interconversion of pyruvate and lactate during glycolysis. #### **2. Protomers** - For example, in a homotetramer, each individual identical subunit is a protomer. - In a heterotetramer, the protomer may consist of a combination of different subunits. - **Specific Examples**: - **Hemoglobin**: The protomer is an αβ heterodimer, as each α and β subunit pair together to form functional dimers. - **Chaperonin (GroEL)**: Each protomer in the GroEL protein complex is a single polypeptide chain that assembles into a heptameric ring. - **ATP Synthase**: The F1 portion of ATP synthase contains protomers like α and β subunits that form a functional catalytic complex. Proteins can be classified based on their structure, composition, function, and solubility. Below is a detailed classification with specific examples **1. Classification Based on Structure** **A. Fibrous Proteins** - **Definition**: These are elongated, insoluble proteins with structural or support roles. They are often arranged in a repetitive sequence. - **Properties**: Strong, flexible, and resistant to stretching. - **Examples**: - **Collagen**: Found in connective tissues like tendons, ligaments, and skin. - **Keratin**: Found in hair, nails, and skin. - **Elastin**: Found in elastic tissues like blood vessels and lungs. **B. Globular Proteins** - **Definition**: Compact, spherical, water-soluble proteins that are involved in dynamic processes such as catalysis and transport. - **Properties**: Folded into a three-dimensional structure. - **Examples**: - **Hemoglobin**: Transports oxygen in blood. - **Myoglobin**: Stores oxygen in muscles. - **Enzymes (e.g., amylase)**: Catalyze biochemical reactions. **C. Membrane Proteins** - **Definition**: Embedded in or associated with cell membranes, playing roles in signaling and transport. - **Examples**: - **Ion Channels**: Transport ions across membranes. - **Receptors (e.g., G-protein coupled receptors)**: Mediate cellular signaling. **2. Classification Based on Composition** **A. Simple Proteins** - **Definition**: Proteins made only of amino acids. - **Examples**: - **Albumin**: Found in egg whites and blood plasma. - **Globulin**: Found in blood plasma and involved in immune responses. **B. Conjugated Proteins** - **Definition**: Proteins combined with a non-protein component (prosthetic group). - **Subtypes and Examples**: - **Glycoproteins**: Protein + Carbohydrate - Example: **Mucin** in mucus. - **Lipoproteins**: Protein + Lipid - Example: **LDL (Low-Density Lipoprotein)**, involved in cholesterol transport. - **Metalloproteins**: Protein + Metal ion - Example: **Ferritin**, stores iron. - **Nucleoproteins**: Protein + Nucleic acid - Example: **Histones**, associated with DNA in chromosomes. Note: The non-amino acid component of a conjugated protein is called prosthetic group **3. Classification Based on Function** **A. Structural Proteins** - **Function**: Provide support and structure to cells and tissues. - **Examples**: - **Collagen**: Gives strength to connective tissues. - **Actin and Myosin**: Components of muscle fibers. **B. Transport Proteins** - **Function**: Carry molecules across membranes or through the bloodstream. - **Examples**: - **Hemoglobin**: Transports oxygen. - **Albumin**: Transports fatty acids and hormones. **C. Enzymatic Proteins** - **Function**: Catalyze biochemical reactions. - **Examples**: - **Lipase**: Breaks down fats. - **DNA Polymerase**: Catalyzes DNA replication. **D. Regulatory Proteins** - **Function**: Regulate biological processes. - **Examples**: - **Insulin**: Regulates blood sugar levels. - **Growth Hormone**: Stimulates growth and cell reproduction. **E. Defensive Proteins** - **Function**: Protect the body from pathogens or injury. - **Examples**: - **Antibodies (Immunoglobulins)**: Target and neutralize pathogens. - **Fibrinogen**: Involved in blood clotting. **F. Storage Proteins** - **Function**: Store nutrients or molecules for later use. - **Examples**: - **Ferritin**: Stores iron. - **Casein**: Stores amino acids in milk. **4. Classification Based on Solubility** **A. Albumins** - **Properties**: Soluble in water. - **Examples**: - **Serum Albumin**: Maintains osmotic pressure in blood. **B. Globulins** - **Properties**: Soluble in dilute salt solutions but insoluble in water. - **Examples**: - **Immunoglobulins**: Play a role in immune responses. **C. Prolamins** - **Properties**: Soluble in alcohol. - **Examples**: - **Gliadin**: Found in wheat; contributes to gluten. **D. Glutelins** - **Properties**: Soluble in acids or bases. - **Examples**: - **Glutenin**: Found in wheat; contributes to elasticity of dough. This classification highlights the diversity of proteins and their roles in biological systems, illustrating their significance in life processes. **ISOLATION, PURIFICATION AND IDENTIFICATION OF PROTEINS** **Biochemists are able to understand the structure and functions of proteins by studying them individually. Before a protein could be studied in details, the researcher must first separate the protein of interest from other proteins in a pure form and use appropriate biochemical techniques to identify and determine their properties..** **The importance of separation when working with proteins stems from the fact that cells contain thousands of different kinds of protein. Conventional separating techniques utilize properties that differ from one protein to another including size, charge and binding properties.** **ISOLATION OF PROTEINS** Breaking open cells to extract proteins is a crucial step in various biological research and applications. The choice of method depends on the type of cells, desired protein yield, and sensitivity of the proteins to different treatments. Below are some common methods: **Mechanical Methods:** - Manual Grinding: This method involves grinding cells using a mortar and pestle, often with liquid nitrogen to freeze the tissue, making it more brittle. It\'s suitable for small-scale and tough samples like plant tissues.  - Bead Beating: Cells are lysed by vigorous shaking with small, hard beads (glass, ceramic, or metal) in a specialized machine. This method is efficient for disrupting a wide range of cells, including bacteria and yeast. - C:\\Users\\toshiba\\Desktop\\lecture 201 images\\images (1).jpg - Sonication: High-frequency sound waves disrupt cell membranes. It\'s a versatile technique suitable for various cell types but can generate heat, potentially damaging proteins.  - Homogenization: Cells are forced through a narrow opening at high pressure, shearing them apart. This method is effective for large volumes of cells but can also generate heat. C:\\Users\\toshiba\\Desktop\\lecture 201 images\\images (3).jpg - Freeze-Thaw Cycles: Repeated cycles of freezing and thawing disrupt cell membranes as ice crystals form and expand. This method is gentle but can be time-consuming. Chemical Methods: - Detergents: Detergents solubilize cell membranes, releasing proteins into solution. Different detergents have varying strengths and are chosen based on the type of cells and proteins of interest. - Enzymatic Lysis: Enzymes like lysozyme (for bacteria) or cellulase (for plant cells) can specifically degrade cell wall components, releasing cellular contents. - Osmotic Shock: Cells are exposed to a sudden change in osmotic pressure, causing them to lyse. This method is suitable for bacteria with weak cell walls. **Considerations**: - Protein Stability: The chosen method should minimize protein degradation and denaturation. - Cell Type: Different cell types have varying cell wall and membrane structures, requiring different lysis methods. - Protein Yield: The method should maximize protein extraction while minimizing cell debris. - Downstream Applications: The lysis method can impact subsequent steps like protein purification and analysis. By carefully considering these factors, researchers can select the most appropriate method for their specific protein extraction needs. The resulting products from all the above processes are known **as crude extracts.** **PURIFICATION of CRUDE PROTEIN EXTRACTS** After the crude protein has been obtained. Various methods are available for purifying one or more of the proteins it contains. Generally, the extract is subject to methods that separate the proteins based on different properties such as size or charge. These processes are collectively known as **FRACTIONATION.** Different methods of fractionation are explained below: **Principle and Procedure of Separating Crude Protein by Centrifugation**  **Principle:** Centrifugation is a technique that uses centrifugal force to separate particles from a solution based on their size, shape, and density. When a sample is spun at high speed, the denser particles are forced to the bottom of the tube, forming a pellet, while the lighter components remain in the supernatant. **Procedure:** **1. Sample Preparation:** - **Cell Lysis:** If working with cells, lyse them using appropriate methods like sonication, homogenization, or chemical lysis to release cellular contents, including proteins. - **Crude Extract:** Collect the cell lysate or other crude protein mixture. **2. Centrifugation:** - **Low-Speed Centrifugation:** - **Purpose:** To remove large cellular debris, such as unbroken cells and nuclei. - **Procedure:** 1. Transfer the crude extract to centrifuge tubes. 2. Spin the tubes at a low speed (e.g., 10,000 x g) for a short duration (e.g., 10 minutes). 3. The pellet will contain cellular debris, while the supernatant will contain smaller particles, including proteins. 4. Carefully transfer the supernatant to a new tube. - **High-Speed Centrifugation:** - **Purpose:** To separate smaller particles, such as organelles and membrane fragments, from soluble proteins. - **Procedure:** 5. Centrifuge the supernatant from the previous step at a higher speed (e.g., 100,000 x g) for a longer duration (e.g., 1 hour). 6. The pellet will contain organelles and membrane fragments, while the supernatant will contain soluble proteins. **3. Pellet Recovery (Optional):** - If the pellet contains the desired protein, resuspend it in a suitable buffer. - Further purification steps, such as chromatography, can be applied to isolate specific proteins. **Additional Considerations:** - **Centrifuge Type:** Choose a centrifuge with appropriate speed and capacity for your sample volume. - **Centrifuge Tubes:** Use tubes that can withstand the required centrifugal force and are compatible with your centrifuge. - **Temperature Control:** Consider using a refrigerated centrifuge to minimize protein degradation. - **Buffer Conditions:** The buffer used should maintain protein stability and prevent aggregation. - **Multiple Rounds of Centrifugation:** For more precise separation, multiple rounds of centrifugation at different speeds can be performed. By carefully controlling the centrifugation parameters, it is possible to effectively separate crude protein mixtures into different fractions based on their size and density. **Dialysis: A Gentle Method for Protein Purification** C:\\Users\\toshiba\\Desktop\\lecture 201 images\\download (1).jpg **Dialysis** is a technique used to separate molecules on the basis of size, primarily used to remove small molecules like salts and buffers from a protein solution while retaining the larger protein molecules. It\'s a gentle method that avoids denaturing proteins. **How it Works:** 1. **Semi-Permeable Membrane:** A dialysis membrane, a thin, porous film, is used. The pores are large enough to allow small molecules like salts and buffers to pass through but too small for larger molecules like proteins. 2. **Diffusion:** The protein solution is placed inside a dialysis bag or tube made of this membrane. The bag is then immersed in a large volume of buffer solution. 3. **Concentration Gradient:** Due to the concentration gradient, small molecules diffuse out of the dialysis bag into the surrounding buffer, while the larger proteins remain inside. 4. **Equilibrium:** Over time, an equilibrium is reached where the concentration of small molecules inside and outside the bag is equal. **Applications of Dialysis in Protein Extraction:** - **Buffer Exchange:** Replacing the buffer solution surrounding a protein with a different buffer. This is crucial for subsequent experiments or storage. - **Salt Removal:** Removing excess salts that can interfere with protein activity or subsequent purification steps. - **Small Molecule Removal:** Removing small molecule contaminants like detergents or reagents that might have been used during protein purification. **Key Considerations:** - **Membrane Molecular Weight Cut-off (MWCO):** Choose a membrane with an appropriate MWCO to ensure efficient removal of small molecules while retaining the protein of interest. - **Dialysis Buffer:** Select a buffer that is compatible with the protein and the desired experimental conditions. - **Dialysis Time:** The time required for dialysis depends on the size of the molecules to be removed, the membrane MWCO, and the volume of the dialysis buffer. - **Dialysis Temperature:** Temperature can influence the rate of diffusion. By carefully selecting the dialysis conditions, dialysis can be a powerful tool for purifying and preparing proteins for further analysis or experimentation **Chromatography: A Powerful Tool for Protein Purification** Chromatography is a versatile technique used to separate mixtures of substances, including proteins. It involves the distribution of components between a stationary phase and a mobile phase. The principle is that different components interact differently with the stationary phase, leading to their separation. Here are some common types of chromatography used in protein purification: **1. Size-Exclusion Chromatography (Gel Filtration Chromatography)**  - **Principle:** Separates proteins based on their size and molecular weight. - **Mechanism:** A porous gel matrix acts as the stationary phase. Larger proteins cannot enter the pores and elute first, while smaller proteins are retained and elute later. - **Application:** Used to determine the molecular weight of proteins and to remove small molecules like salts. **2. Ion-Exchange Chromatography** C:\\Users\\toshiba\\Desktop\\lecture 201 images\\download (2).jpg - **Principle:** Separates proteins based on their net charge. - **Mechanism:** The stationary phase is a resin with charged groups. Proteins with opposite charges bind to the resin, while those with the same charge elute. - **Application:** Used to separate proteins based on their pI (isoelectric point). **3. Affinity Chromatography**  - **Principle:** Separates proteins based on their specific binding to a ligand immobilized on the stationary phase. - **Mechanism:** The target protein binds to the ligand, while other proteins pass through the column. The bound protein can then be eluted by changing the buffer conditions or adding a competing ligand. - **Application:** Highly specific purification of proteins, such as antibodies or enzymes. 5. **Hydrophobic Interaction Chromatography (HIC)** C:\\Users\\toshiba\\Desktop\\lecture 201 images\\download (6).jpg - **Principle:** Separates proteins based on their hydrophobic properties. - **Mechanism:** A hydrophobic stationary phase binds hydrophobic proteins. Proteins can be eluted by decreasing the salt concentration, which reduces hydrophobic interactions. - **Application:** Purifying proteins that are stable in high salt concentrations. 6. **High-Performance Liquid Chromatography (HPLC)**  - **Principle:** A high-pressure liquid is used as the mobile phase to force the sample through a packed column. - **Mechanism:** The stationary phase can be various types, such as ion-exchange, size-exclusion, or reverse-phase. - **Application:** High-resolution separation of complex protein mixtures. By combining these techniques and optimizing the conditions, researchers can achieve highly purified proteins for various applications, including structural studies, functional assays, and therapeutic develop **Key Terms in Chromatography** Here are some key terms commonly used in chromatography: **Fundamental Terms** - **Stationary Phase:** The fixed phase in a chromatographic system, usually a solid or a liquid supported on a solid. - **Mobile Phase:** The phase that moves through the stationary phase, carrying the sample components. It can be a liquid or a gas. - **Analyte:** The substance being separated or analyzed. - **Eluent:** The mobile phase that carries the analyte through the stationary phase. - **Eluate:** The solution that emerges from the column containing the separated analytes. **The retention factor (Rf) is a ratio that measures how far a compound travels in chromatography compared to the distance the solvent travels: ** +-----------------------------------+-----------------------------------+ | | **Explanation** | +===================================+===================================+ | Formula | Rf = distance traveled by | | | compound / distance traveled by | | | solvent | +-----------------------------------+-----------------------------------+ | What it measures | How far a compound travels | | | compared to the distance it could | | | have traveled if it moved with | | | the solvent front | | | | | | C:\\Users\\toshiba\\Desktop\\lect | | | ure | | | 201 images\\download.png | | | | | | **Electrophoresis in Protein | | | Purification** | | | | | | Electrophoresis is a powerful | | | technique used to separate and | | | analyze proteins based on their | | | size and charge. It involves | | | applying an electric field to a | | | gel matrix containing ^1^ the | | | protein sample. The proteins | | | migrate through the gel at | | | different rates, allowing for | | | their separation. | | | | | |  | | | | | | **Electrophoresis in Protein | | | Purification:** | | | | | | **1. Sodium Dodecyl | | | Sulfate-Polyacrylamide Gel | | | Electrophoresis (SDS-PAGE):** | | | | | | - **Principle:** SDS-PAGE | | | separates proteins based on | | | their molecular weight. | | | | | | - **Process:** | | | | | | 1. **Denaturation:** | | | Proteins are denatured | | | using SDS, a detergent | | | that imparts a negative | | | charge to proteins, | | | masking their native | | | charge. | | | | | | 2. **Gel Electrophoresis:** | | | The denatured proteins | | | are loaded into wells in | | | a polyacrylamide gel and | | | an electric field is | | | applied. | | | | | | 3. **Migration:** Smaller | | | proteins migrate faster | | | through the gel pores | | | than larger ones. | | | | | | **2. Isoelectric Focusing | | | (IEF):** | | | | | | - **Principle:** IEF separates | | | proteins based on their | | | isoelectric point (pI), the | | | pH at which a protein has no | | | net charge. | | | | | | - **Process:** | | | | | | 1. **pH Gradient:** A pH | | | gradient is established | | | in the gel. | | | | | | 2. **Protein Migration:** | | | Proteins migrate until | | | they reach their pI, | | | where they stop moving. | | | | | | **3. Two-Dimensional Gel | | | Electrophoresis (2-DE):** | | | | | | - **Principle:** Combines IEF | | | and SDS-PAGE to separate | | | proteins based on both their | | | pI and molecular weight. | | | | | | - **Process:** | | | | | | 1. **First Dimension | | | (IEF):** Proteins are | | | separated by IEF. | | | | | | 2. **Second Dimension | | | (SDS-PAGE):** The IEF gel | | | is placed on top of an | | | SDS-PAGE gel and | | | electrophoresis is | | | performed. | | | | | | **Applications of Electrophoresis | | | in Protein Purification:** | | | | | | - **Protein Separation:** | | | Separating proteins based on | | | their size, charge, or both. | | | | | | - **Protein Identification:** | | | Identifying proteins using | | | techniques like mass | | | spectrometry. | | | | | | - **Protein Purity | | | Assessment:** Determining the | | | purity of a protein sample. | | | | | | - **Protein Quantification:** | | | Estimating the amount of | | | protein in a sample. | | | | | | - **Protein Characterization:** | | | Studying the properties of | | | proteins, such as their | | | molecular weight and | | | isoelectric point. | | | | | | By understanding the principles | | | and applications of different | | | electrophoresis techniques, | | | researchers can effectively | | | purify and analyze proteins for | | | various biological and biomedical | | | research purposes. | | | | | | **Spectrophotometry in Protein | | | Identification** | | | | | | **Principle:** Spectrophotometry | | | is a technique that measures the | | | amount of light absorbed by a | | | substance at a specific | | | wavelength. In the context of | | | protein identification, it | | | leverages the fact that proteins | | | contain amino acids with aromatic | | | side chains, such as tryptophan, | | | tyrosine, and phenylalanine, | | | which absorb ultraviolet (UV) | | | light. | | | | | | **Application:** | | | | | | 1. **Protein Concentration | | | Determination:** | | | | | | - **Direct UV Absorption:** | | | By measuring the | | | absorbance of a protein | | | solution at 280 nm, the | | | concentration can be | | | estimated. This is a | | | rapid and non-destructive | | | method. | | | | | | - **Colorimetric Assays:** | | | Protein assays like the | | | Bradford, Lowry, or BCA | | | assays involve adding | | | reagents to the protein | | | solution to form colored | | | complexes. The intensity | | | of the color, measured at | | | a specific wavelength, is | | | proportional to the | | | protein concentration. | | | | | | 2. **Protein Purity | | | Assessment:** | | | | | | - By monitoring the | | | absorbance at 280 nm and | | | 260 nm, the purity of a | | | protein sample can be | | | assessed. A high 280/260 | | | ratio indicates a purer | | | protein sample, as | | | nucleic acids absorb | | | strongly at 260 nm. | | | | | | 3. **Enzyme Activity | | | Measurement:** | | | | | | - Spectrophotometry can be | | | used to measure the rate | | | of enzyme-catalyzed | | | reactions by monitoring | | | the change in absorbance | | | of a substrate or | | | product. | | | | | | 4. **Protein-Ligand | | | Interactions:** | | | | | | - By measuring changes in | | | absorbance upon ligand | | | binding, information | | | about binding affinity | | | and stoichiometry can be | | | obtained. | | | | | | **Beer-Lambert Law:** The | | | Beer-Lambert Law is the | | | fundamental principle underlying | | | spectrophotometry. It states that | | | the absorbance of a solution is | | | directly proportional to the | | | concentration of the absorbing | | | species and the path length of | | | the light through the solution. | | | | | | Mathematically, it is expressed | | | as: | | | | | | A = εcl | | | | | | where: | | | | | | - A = absorbance | | | | | | - ε = molar absorptivity (a | | | constant specific to the | | | substance) | | | | | | - c = concentration of the | | | substance | | | | | | - l = path length of the light | | | through the solution | | | | | | By measuring the absorbance of a | | | protein solution at a specific | | | wavelength and applying the | | | Beer-Lambert Law, the | | | concentration of the protein can | | | be determined. | | | | | | **Limitations:** While | | | spectrophotometry is a valuable | | | tool, it has limitations, such | | | as: | | | | | | - **Sensitivity:** It may not | | | be sensitive enough for | | | detecting low concentrations | | | of proteins. | | | | | | - **Specificity:** It may not | | | be specific for a particular | | | protein, especially in | | | complex mixtures. | | | | | | - **Interference:** Other | | | substances in the sample may | | | interfere with the absorbance | | | measurement. | | | | | | To overcome these limitations, | | | spectrophotometry is often used | | | in conjunction with other | | | techniques like chromatography | | | and electrophoresis to provide a | | | more comprehensive analysis of | | | protein samples. | | | | | | **Mass Spectrometry in Protein | | | Analysis** | | | | | | Mass spectrometry (MS) is a | | | powerful analytical technique | | | that has revolutionized protein | | | analysis. It enables the precise | | | measurement of the mass-to-charge | | | ratio (m/z) of ions, providing | | | valuable information about | | | protein identity, structure, and | | | modifications. | | | | | | **Key Steps in Protein Mass | | | Spectrometry:** | | | | | | 1. **Sample Preparation:** | | | | | | - **Protein Digestion:** | | | Proteins are often | | | digested into smaller | | | peptides using enzymes | | | like trypsin. This | | | increases the efficiency | | | of ionization and mass | | | analysis. | | | | | | - **Sample Cleanup:** | | | Techniques like | | | solid-phase extraction | | | (SPE) or liquid-liquid | | | extraction are used to | | | remove contaminants and | | | concentrate the protein | | | sample. | | | | | | 2. **Ionization:** | | | | | | - **Electrospray Ionization | | | (ESI):** A solution | | | containing the protein | | | sample is sprayed through | | | a fine needle at high | | | voltage. The droplets | | | evaporate, leaving behind | | | gas-phase ions. | | | | | | - **Matrix-Assisted Laser | | | Desorption/Ionization | | | (MALDI):** The protein | | | sample is mixed with a | | | matrix compound and | | | crystallized on a target | | | plate. A laser pulse | | | vaporizes the matrix and | | | proteins, forming ions. | | | | | | 3. **Mass Analysis:** | | | | | | - **Time-of-Flight (TOF) | | | Mass Spectrometer:** Ions | | | are accelerated by an | | | electric field and their | | | time of flight to a | | | detector is measured. The | | | time of flight is | | | directly proportional to | | | the mass-to-charge ratio. | | | | | | | | | - **Quadrupole Mass | | | Spectrometer:** Ions are | | | filtered based on their | | | m/z ratio by applying | | | oscillating electric | | | fields to four rods. | | | | | | - **Orbitrap Mass | | | Spectrometer:** Ions are | | | trapped in an orbitrap | | | cell and their | | | oscillation frequency is | | | measured, which is | | | related to their m/z | | | ratio. | | | | | | 4. **Data Analysis:** | | | | | | - **Peptide Mass | | | Fingerprinting:** The | | | masses of peptide | | | fragments generated from | | | protein digestion are | | | compared to theoretical | | | peptide masses from a | | | protein database. | | | | | | - **Tandem Mass | | | Spectrometry (MS/MS):** | | | Peptide ions are | | | fragmented, and the | | | resulting fragment ions | | | are analyzed to determine | | | the amino acid sequence. | | | | | | | | | - **Bioinformatics Tools:** | | | Software tools are used | | | to analyze mass | | | spectrometry data and | | | identify proteins, | | | modifications, and | | | interactions. | | | | | | **Applications of Mass | | | Spectrometry in Protein | | | Analysis:** | | | | | | - **Protein Identification:** | | | Identifying proteins in | | | complex mixtures, such as | | | those from cells or tissues. | | | | | | | | | - **Protein Quantification:** | | | Measuring the relative | | | abundance of proteins in | | | different samples. | | | | | | - **Post-Translational | | | Modification Analysis:** | | | Detecting and characterizing | | | modifications like | | | phosphorylation, | | | glycosylation, and | | | acetylation. | | | | | | - **Protein Structure | | | Analysis:** Determining | | | protein structure and | | | conformation. | | | | | | - **Protein Interaction | | | Studies:** Identifying | | | protein-protein interactions | | | and protein-ligand | | | interactions. | | | | | | Mass spectrometry has become an | | | indispensable tool in proteomics | | | research, enabling scientists to | | | investigate a wide range of | | | biological questions. | | | | | | **Bioinformatics in Protein | | | Analysis** | | | | | | Bioinformatics plays a crucial | | | role in protein analysis, | | | enabling researchers to extract | | | valuable insights from complex | | | biological data. By combining | | | computational tools and | | | biological knowledge, | | | bioinformatics empowers | | | scientists to understand protein | | | structure, function, and | | | interactions at a molecular | | | level. | | | | | | **Key Bioinformatics Techniques | | | in Protein Analysis:** | | | | | | 1. **Sequence Analysis:** | | | | | | - **Homology Search:** | | | Identifying similar | | | sequences in databases | | | using tools like BLAST. | | | | | | - **Domain and Motif | | | Prediction:** Identifying | | | functional regions within | | | protein sequences. | | | | | | - **Multiple Sequence | | | Alignment:** Aligning | | | multiple protein | | | sequences to identify | | | conserved regions and | | | evolutionary | | | relationships. | | | | | | - **Phylogenetic | | | Analysis:** Constructing | | | evolutionary trees to | | | understand the | | | evolutionary history of | | | proteins. | | | | | | 2. **Structure Prediction:** | | | | | | - **Ab Initio Prediction:** | | | Predicting protein | | | structure from amino acid | | | sequence without relying | | | on experimental data. | | | | | | - **Homology Modeling:** | | | Building protein models | | | based on the structure of | | | homologous proteins. | | | | | | - **Threading:** Fitting a | | | protein sequence onto | | | known protein structures. | | | | | | 3. **Functional Prediction:** | | | | | | - **Functional | | | Annotation:** Assigning | | | functions to proteins | | | based on sequence | | | similarity and structural | | | information. | | | | | | - **Protein-Protein | | | Interaction Prediction:** | | | Identifying potential | | | interactions between | | | proteins. | | | | | | - **Enzyme Function | | | Prediction:** Predicting | | | the catalytic activity of | | | enzymes. | | | | | | 4. **Proteomics Data Analysis:** | | | | | | - **Peptide | | | Identification:** | | | Matching peptide mass | | | spectra to theoretical | | | peptide masses from | | | protein databases. | | | | | | - **Protein | | | Quantification:** | | | Estimating the abundance | | | of proteins in a sample. | | | | | | - **Post-Translational | | | Modification Analysis:** | | | Identifying and | | | characterizing | | | modifications like | | | phosphorylation, | | | glycosylation, and | | | acetylation. | | | | | | **Bioinformatics Tools and | | | Databases:** | | | | | | - **BLAST:** Basic Local | | | Alignment Search Tool for | | | sequence similarity searches. | | | | | | - **ClustalW:** Multiple | | | sequence alignment tool. | | | | | | - **HMMER:** Profile Hidden | | | Markov Models for protein | | | family identification. | | | | | | - **MODELLER:** Homology | | | modeling software. | | | | | | - **I-TASSER:** Ab initio | | | protein structure prediction | | | server. | | | | | | - **UniProtKB:** A | | | comprehensive protein | | | sequence and function | | | database. | | | | | | - **PDB:** Protein Data Bank, | | | containing experimentally | | | determined protein | | | structures. | | | | | | **Applications of Bioinformatics | | | in Protein Analysis:** | | | | | | - **Drug Discovery:** | | | Identifying potential drug | | | targets and designing novel | | | drugs. | | | | | | - **Disease Diagnosis:** | | | Developing diagnostic markers | | | for diseases. | | | | | | - **Agricultural | | | Biotechnology:** Improving | | | crop yields and resistance to | | | pests and diseases. | | | | | | - **Environmental Science:** | | | Studying the impact of | | | environmental factors on | | | protein function. | | | | | | By leveraging the power of | | | bioinformatics, researchers can | | | accelerate the pace of protein | | | research and contribute to | | | advancements in medicine, | | | agriculture, and other fields. | | | | | | **Protein Structures and Their | | | Relevance to Function** | | | | | | Proteins are the workhorses of | | | the cell, performing a vast array | | | of functions that depend on their | | | structure. Protein structure can | | | be organized into four | | | hierarchical levels: | | | | | | **1. Primary Structure** | | | | | | - **Definition**: The linear | | | sequence of amino acids in a | | | polypeptide chain. | | | | | | - **Relevance to Function**: | | | | | | - Determines how the | | | protein will fold (via | | | interactions between side | | | chains). | | | | | | - Mutations in the sequence | | | (e.g., a single amino | | | acid substitution) can | | | drastically alter | | | function, as seen in | | | diseases like sickle-cell | | | anemia. | | | | | | **2. Secondary Structure** | | | | | | - **Definition**: Localized | | | folding patterns stabilized | | | by hydrogen bonds between the | | | backbone atoms. The two main | | | types are: | | | | | | - **α-Helices**: Coiled | | | structures stabilized by | | | hydrogen bonds (e.g., | | | found in DNA-binding | | | proteins like | | | transcription factors). | | | | | | - **β-Sheets**: Extended, | | | pleated structures that | | | can be parallel or | | | antiparallel (e.g., seen | | | in structural proteins | | | like silk fibroin). | | | | | | - **Relevance to Function**: | | | | | | - Provides scaffolding and | | | contributes to protein | | | stability. | | | | | | - Misfolding of secondary | | | structures can lead to | | | diseases, such as | | | β-sheet-rich amyloid | | | plaques in Alzheimer's | | | disease. | | | | | | **3. Tertiary Structure** | | | | | | - **Definition**: The overall | | | 3D shape of a protein, | | | stabilized by interactions | | | such as: | | | | | | - Hydrophobic interactions, | | | hydrogen bonds, ionic | | | bonds, van der Waals | | | forces, and disulfide | | | bridges. | | | | | | - **Relevance to Function**: | | | | | | - Determines the active | | | site in enzymes, critical | | | for catalytic activity | | | (e.g., the | | | \"lock-and-key\" or | | | \"induced-fit\" models). | | | | | | - Enables interactions with | | | other molecules, such as | | | substrates, ligands, or | | | other proteins. | | | | | | - Structural changes in the | | | tertiary conformation can | | | regulate activity, such | | | as allosteric regulation | | | in hemoglobin. | | | | | | **4. Quaternary Structure** | | | | | | - **Definition**: The assembly | | | of multiple polypeptide | | | chains (subunits) into a | | | functional complex. | | | | | | - **Examples**: Hemoglobin | | | (tetramer) and ATP | | | synthase (multi-subunit | | | complex). | | | | | | - **Relevance to Function**: | | | | | | - Allows cooperative | | | binding (e.g., | | | hemoglobin\'s oxygen | | | transport). | | | | | | - Increases stability and | | | functionality (e.g., | | | collagen fibers provide | | | tensile strength). | | | | | | - Mutations affecting | | | subunit interfaces can | | | disrupt function (e.g., | | | certain inherited | | | anemias). | | | | | | **Folding and Misfolding** | | | | | | - Proper folding is essential | | | for function and is | | | facilitated by chaperones. | | | | | | - Misfolded proteins can form | | | aggregates, leading to | | | diseases such as: | | | | | | - Alzheimer's (amyloid | | | plaques). | | | | | | - Parkinson's (Lewy | | | bodies). | | | | | | - Prion diseases (misfolded | | | infectious proteins). | | | | | | **Based on the more complex | | | staructure of proteins. They can | | | be classified into fibrous and | | | globular proteins** | | | | | | **A. Fibrous Proteins** | | | | | | - **Description**: Elongated | | | and provide structural | | | support. | | | | | | - **Examples**: Collagen, | | | Keratin. | | | | | | **B. Globular Proteins** | | | | | | - **Description**: Compact and | | | spherical, usually | | | water-soluble. | | | | | | - **Examples**: Hemoglobin, | | | Enzymes. | | | | | | **Conclusion** | | | | | | The structure of a protein at all | | | levels determines its | | | functionality. Any disruption in | | | structure---whether due to | | | genetic mutations, environmental | | | factors, or misfolding---can | | | impair or entirely abolish its | | | function, often leading to | | | disease. Understanding protein | | | structure is thus fundamental to | | | fields like biochemistry, | | | molecular biology, and drug | | | design. | | | | | | ### **Basic Principles of Tests f | | | or Amino Acids and Proteins** | | | | | | Tests for amino acids and | | | proteins rely on their unique | | | chemical and structural | | | properties. These tests are | | | commonly used in laboratories to | | | identify, quantify, or confirm | | | the presence of proteins or | | | specific amino acids. | | | | | | ### **1. General Principles** | | | | | | - **Chemical Reactions**: Tests | | | exploit functional groups in | | | amino acids (e.g., amine, | | | carboxyl, hydroxyl, or | | | sulfhydryl groups) and | | | specific bonds in proteins | | | (e.g., peptide bonds). | | | | | | - **Color Changes**: Many tests | | | involve reactions that | | | produce a distinct color, | | | allowing for qualitative or | | | quantitative detection. | | | | | | - **Specificity**: Some tests | | | are general for all proteins | | | or amino acids, while others | | | target specific functional | | | groups or types of amino | | | acids. | | | | | | ### **2. Common Tests for Protein | | | s** | | | | | | #### **a) Biuret Test** | | | | | | - **Principle**: The test | | | detects the presence of | | | peptide bonds. In an alkaline | | | environment, copper(II) ions | | | react with peptide bonds to | | | form a violet-colored | | | complex. | | | | | | - **Procedure**: Add Biuret | | | reagent (a solution of copper | | | sulfate and sodium hydroxide) | | | to the sample. | | | | | | - **Positive Result**: | | | Appearance of a violet color. | | | | | | - **Relevance**: General test | | | for proteins but does not | | | detect free amino acids. | | | | | | #### **b) Xanthoproteic Test** | | | | | | - **Principle**: Aromatic amino | | | acids (e.g., tyrosine, | | | tryptophan, phenylalanine) | | | undergo nitration with | | | concentrated nitric acid, | | | forming yellow nitro | | | compounds. | | | | | | - **Procedure**: Add | | | concentrated nitric acid to | | | the sample and heat. | | | Neutralize with sodium | | | hydroxide. | | | | | | - **Positive Result**: Yellow | | | color changes to orange when | | | neutralized. | | | | | | - **Relevance**: Detects | | | proteins containing aromatic | | | amino acids. | | | | | | #### **c) Ninhydrin Test** | | | | | | - **Principle**: Ninhydrin | | | reacts with free amino groups | | | in amino acids or the | | | N-terminal amino group in | | | peptides, producing a | | | purple-blue complex | | | (Ruhemann's purple). | | | | | | - **Procedure**: Heat the | | | sample with ninhydrin | | | reagent. | | | | | | - **Positive Result**: | | | Purple-blue color. | | | | | | - **Relevance**: Used for | | | detecting free amino acids or | | | small peptides. | | | | | | #### **d) Millon's Test** | | | | | | - **Principle**: Tyrosine | | | reacts with mercuric ions in | | | Millon's reagent to form a | | | red complex. | | | | | | - **Procedure**: Add Millon's | | | reagent (mercury nitrate in | | | nitric acid) to the sample | |