Lecture (Chp 11 - 13) Cardiovascular System 1 2024 PDF
Document Details
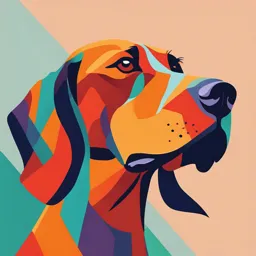
Uploaded by BalancedHeliotrope2087
The University of Zambia
2024
Dr. Houston
Tags
Summary
This lecture covers the Cardiovascular System; it includes topics on the applied human anatomy and physiology of the cardiovascular system.
Full Transcript
Department of Physician Assistant Medicine PAM 600 Applied Human Anatomy and Physiology Cardiovascular 1 Fall Dr. Houston 1 Lecture CARDIOVASCULAR PHYSIOLOGY...
Department of Physician Assistant Medicine PAM 600 Applied Human Anatomy and Physiology Cardiovascular 1 Fall Dr. Houston 1 Lecture CARDIOVASCULAR PHYSIOLOGY Medical Physiology Big Picture Physiology Chapter 11 Chapter 4 Overview of the Cardiovascular Fundamentals System and Hemodynamics Organization of the CV System Hemodynamics Chapter 12 Structure & Function of Cardiac Muscle Electrical Activity of the Heart Cardia Electrophysiology Chapter 13 The Cardiac Cycle Cardiac Muscle Mechanics and the Cardiac Output Cardiac Pump 2 Chapter 11 - Lecture Overview Overview of the Cardiovascular System and Hemodynamics Functional Organization Driving Pressure Resistance and Smooth Muscle Other factors affecting resistance – Vessel length – Blood viscosity 3 Chapter 11: Overview of the Cardiovascular System and Hemodynamics CHAPTER 11 LEARNING OBJECTIVE SLIDE # 1 Explain why the outputs of the left and right ventricle are interdependent. 5 2 Explain how the parallel arrangement of arteries supplying systemic organs 5 allows independent control of each organ’s blood flow. 3 Explain the mechanisms by which major physiological vasoconstrictors and 7-10 vasodilators contract or relax arteries and veins. 4 Explain how vascular compliance is altered by vascular smooth muscle 17 contraction and aging. 5 Explain why changes in blood volume alter volume in the venous side of 8 & Chp 14 the circulation more than on the arterial side of the circulation. 6 Explain why standing causes blood to drain from regions above the heart Chp 14 and pool in the lower extremities. 8 Explain how changes in arterial pressure and vascular resistance alter 7-13 blood flow and how blood flow and vascular resistance affect arterial pressure. 9 Predict how a vasoactive agent will directly affect blood pressure or organ 9-10 blood flow. 10 Explain the hemodynamic consequences of polycythemia and dehydration 16 on the ability of the heart to supply adequate blood flow to systemic organs. FUNCTIONAL ORGANIZATION Blood flow depends on the pressure difference between arteries and veins and on how much resistance to flow is offered by the vascular system. Arterioles are most significant point of control of flow to capillary beds – On proximal side of capillary beds and best positioned to regulate flow into the capillaries – Outnumber any other type of artery, providing the most numerous control points – More muscular in proportion to their diameter. Highly capable of changing radius Flow (Q) is proportional to driving pressure gradient (ΔP) and inversely proportional to resistance (R): 5 Figure 4–1. Overview of the cardiovascular system. Driving Pressure 11B: Physics of movement Rate of flow depends on the pressure difference, NOT the absolute pressure The greater the pressure difference between two points, the greater the flow; the greater the resistance, the less the flow Figure X: Systemic blood flow Figure X: Pressure and flow ©McGraw-Hill Education. All rights reserved. Authorized only for instructor use in the classroom. No reproduction or further distribution permitted without the prior written consent of McGraw-Hill Education. Resistance A tube will offer greater resistance (R) to fluid flow if its length (L) increases or if the radius (r) is decreased. Resistance is also greater if the fluid moved has a higher viscosity (η). Poiseuille’s law describes the determinants of resistance: Figure 4–2. Effect of changing vessel radius on flow. Radius is the dominant variable that determines resistance because radius is raised to the fourth power; for example, doubling the vessel radius increases flow by a factor of 16 Physiologic control of vascular resistance is achieved by altering the blood vessel diameter through vasoconstriction and Figure X. Effect of smooth muscle on radius. vasodilation. 7 Vascular smooth muscle actively controls the diameter of arteries and veins. “ne ws c k” ock s o ” “old Veins are Arteries are the blood the blood volume pressure reservoir reservoir r o n vascula More W ee k 5 A& P i n Figure X: Blood vessel histology 8 TABLE 11.1: Factors that physical forces 11A: Organization chemical agents Contract or Relax Vascular hormones Smooth Muscle paracrine substances receptor-mediated hormonal and neurotransmitter agonists from sympathetic nerve 9 TABLE 11.1: Factors that 11A: Organization Contract or Relax Vascular Smooth Muscle 10 The series and parallel arrangement of blood vessels within an organ affects vascular resistance in the organ. Figure X: TPR in series vs. parallel flow general rule of thumb; adding similar-sized arteries in parallel reduces resistance, whereas losing similar-sized arteries in parallel raises vascular resistance. Disease can cause loss of parallel flow thus increasing resistance Long-term aerobic training such as distance running, in which the arteriolar and capillary network increase their numbers in parallel, thereby reducing resistance to blood flow. 11 DISTRIBUTION OF PRESSURE, FLOW, VELOCITY & BLOOD VOLUME Figure 11.8: Pressure and flow velocity profile in the systemic circulation. 12 Figure 4–28. Blood pressures throughout the systemic vasculature. Pressure is highest in the central arteries and lowest in the central veins. The largest pressure decrease occurs across the arterioles, indicating that they are the site of highest vascular resistance. 13 Part B: Pressure Figure X: Mean arterial pressure will increase if the cardiac output, TPR, or both increase. It also predicts that at a constant mean arterial pressure, blood flow through any portion of the vascular tree will increase if TPR decreases. Other factors influencing resistance (and thus flow) i. Vessel length ii. Blood Viscosity iii. Vessel Compliance i. Vessel length Blood pressure decreases over distance as potential energy is lost through friction between blood and blood vessel walls and between blood cells. Pressure and flow decline with distance - arterial vs. venous pressure 15 ii. Blood viscosity (“thickness”) RBC count and albumin concentration elevate viscosity the most Decreased viscosity with anemia and hypoproteinemia speed flow Increased viscosity with polycythemia and dehydration slow flow Figure 11.7: Effect of hematocrit and flow velocity on blood viscosity ©McGraw-Hill Education. All rights reserved. Authorized only for instructor use in the classroom. No reproduction or further distribution permitted without the prior written consent of McGraw-Hill Education. iii. Compliance. Compliance describes the distensibility of a structure and is defined as the volume change produced by a given pressure change: If a structure has low compliance (i.e., it is stiff), applying a normal pressure change (ΔP) will produce a small volume change (ΔV). Vessel compliance is seen in certain heart diseases and also decreases with aging (Figure 11.3) % increase in volume for a given increase in pressure = compliance 17 Figure 11.3: The relationship between volume and transmural pressure in arteries Chapter 12 - Lecture Overview Electrical Activity of the Heart Cardiac Cell Action Potential Overview Ventricular Myocyte Action Potential Pacemaker Cell Action Potential Refractory Periods Electrocardiogram Chapter 12: Electrical Activity of the Heart CHAPTER 12 LEARNING OBJECTIVE SLID E# 1 Contrast electromechanical coupling in cardiac muscle versus skeletal 23 muscle 2 Explain how changes in membrane voltage-gated channels for sodium, 20-24 potassium, and calcium create the five phases of atrial and ventricular muscle action potentials. 3 Explain why it is not possible to create tetanic contraction in cardiac 26 muscle. 9 Explain the mechanism for the effects of acetylcholine and norepinephrine 37-38 on sinoatrial node rhythmicity and how that relates to the effect of these neurotransmitters on heart rate. 11 Explain how the normal ECG waveform is created by electrical events in the 27 myocardium. Pace maker cells automaticity rhythmicity Cardiac Action Potential Intro duction Video ANS modulates cardiac function rather than initiates Cardiac Cells Nexi (gap junctions) functional syncytium Figures X: Extrinsic innervation of the heart 20 Two major types of unique action potentials characterize electrical excitation of the heart. 1. Those observed in the sinoatrial (SA) node and atrioventricular (AV) node are called pacemaker “slow response” action potentials 2. Those characteristics of ventricular and atrial muscle, as well as of Purkinje fibers, are called myocyte “fast response” action potentials 21 SA node action potential – Slow response SA nodal cells are pacemakers Membrane potential depolarizes spontaneously during phase 4 (called pacemaker potential). Action potential generation in the AV node similar to the SA node but has a slower phase 4 depolarization Nodal tissue does not contain fast voltage-gated Na + channels. Phase 4 no stable RMP maximum −60 mV Threshold voltage of about −40 mV triggered via “funny currents/channels If” Slow Na+ influx Phase 0 depolarization via slow L-type voltage-gated Ca2+ channels Ca+ influx slower and shorter than ventricular muscle phase 0 Figure 4–7. Nodal action potential. Phase 4 = unstable pacemaker potential; phase 0 = slow depolarization upon stimulation; and phase 3 = Phase 3 repolarization. If, nonselective cation current; ICa, Ca2+ current; IK, K+ repolarization via K+ efflux current. several ionic pumps restore “RMP” 22 Ventricular muscle action potential – Fast response Action potentials arriving at ventricular myocytes from the ventricular conducting system Triggers rapid spread of action potentials in all ventricular myocytes via gap junctions. Ventricular muscle action potential has a very long duration (250 ms) Five phases (Phase 0, 1, 2, 3 & 4) Phase 4 resting membrane potential (-90mV) interval between action potentials ventricular muscles relaxed Phase 0 initial rapid depolarization RMP to +30 mV via Na+ influx Phase 1 partial repolarization +30 mV to about 0 mV via K+ efflux Phase 2 plateau phase Ca2+ influx matches K+ efflux Phase 3 repolarization to RMP via K+ efflux & Na+/K+ pump Figure 4–6. Ventricular action potential. 23 Excitation-contraction coupling in cardiomyocytes i. Cardiac muscle cells contract without nervous stimulation. ii. Pacemaker cells spontaneously generate action potentials, which spread through gap junctions. iii. Action potentials conducted along T tubules open voltage-gated Ca2+ channels causing entry of extracellular Ca2+ into the cells. iv. Ca2+-induced Ca2+ release is triggered from internal sarcoplasmic reticulum stores. v. Almost all Ca2+ that interacts with troponin C to initiate contraction is derived from internal stores. vi. Contraction occurs via the same sliding filament mechanism as in skeletal muscle “calciu m-ind uc ed releas calciu Ca2+ f e” m rom Phase 2 la xation re 24 Figure X: Handling of calcium in a cardiac muscle cell. Hierarchy of pacemakers. SA node is the normal pacemaker because it has the most rapid rate of phase 4 depolarization. normal sinus rhythm = cardiac excitation progression from SA node through the entire conduction pathway. The intrinsic rate of SA node firing is about 100 beats/min. Normal parasympathetic tone reduces the SA node firing rate to about 70 beats/min at rest. Endurance athletes have enhanced parasympathetic tone, which results in the classic finding of a slow resting HR in trained individuals. The AV node becomes the pacemaker if the SA node fails or transmission to the AV node fails. These patients are in nodal rhythm and typically have resting heart rates of 45–55 beats/min. Ectopic pacemaker. 25 Figure 12.4: The timing of the excitation of various areas of the heart (in seconds) Refractory periods Figure 4–8. Cardiac action potentials. Cardiac action potentials have long refractory periods (RP). No stimulus can produce another action potential during the effective refractory period. The normal mechanical pumping cycle of the heart requires a single excitation event. Any additional action potentials spread rapidly through coupled myocardial cells and produce arrhythmias (inappropriate heart beats). If the ventricular rate is excessive, there is insufficient time between beats for the ventricles to fill. Long refractory = no tetany and allows for diastole 26 Figure X: ECG Electrocardiogram covered in more detail after Thanksgiving and in other courses 27 Chapter 13 - Lecture Overview Cardiac Muscle Mechanics and the Cardiac Pump Cardiac Action Potential Overview Cardiac Cycle – Wiggers Diagram – Pressure-volume loops Cardiac Output – Ejection Fraction – Heart Rate & Chronotropes – Stroke Volume – Frank-Starling Principle – Afterload – Contractility & Inotropes – ANS Effect on Cardiac Output 28 Chapter 13: Cardiac Muscle Mechanics and the Cardiac Pump CHAPTER 13 LEARNING OBJECTIVE SLIDE # 1 Explain how altering calcium regulatory mechanism in myocardial cells can 43-44 alter the inotropic state of the heart. 2 Identify the correct chronological sequence of any set of hemodynamic 30-32 variables associated with the cardiac cycle. 3 Identify the mechanisms responsible for the four primary heart sounds 32 4 Explain how changes in myocardial contractility can counteract reduced 35, 42-44 preload or increased afterload 5 Explain how changes in preload or afterload can counteract reduced 35, 40-41 contractility 6 Describe how changes in end-diastolic volume, aortic pressure, and 35, 39-42 contractility affect stroke volume. 7 Explain how Starling’s law permits matching of right and left ventricular 39 output. 8 Explain how Starling’s law of the heart helps maintain cardiac output when 35, 39 either afterload or heart rate is increased. 10 Describe factors that alter cardiac output through changes in ventricular 40, 42 filling 12 Understand how the reciprocal relationship between heart rate and stroke 35 volume helps maintain cardiac output. Cardiac Cycle Cardiac cycle — one complete contraction and relaxation of all four chambers of the heart Cycle of events in heart – Systole: contraction – Diastole: relaxation Although “systole” and “diastole” can refer to contraction and relaxation of either type of chamber, they usually refer to the action of the ventricles ©McGraw-Hill Education. All rights reserved. Authorized only for instructor use in the classroom. No reproduction or further distribution permitted without the prior written consent of McGraw-Hill Education. The cardiac cycle is the repetitive electrical and mechanical events that occur with each beat of the heart. Electrical events precede mechanical events, which result from the entry of Ca2+ into the myocytes during cardiac action potentials. ECG waves can be correlated with mechanical events Figure X: Cardiac Cycle Figure X. Wiggers diagram, a correlation of electrical and mechanical events during the cardiac cycle. 32 Pressure-volume (PV) loops. 33 Figure 4–22. Left ventricular pressure-volume loop. Cardiac Output CO = HR SV Stroke Volume: Volume of blood ejected from each ventricle per beat. SV = EDV – ESV EDV: End Diastolic Volume Volume of blood in ventricle at the end of ventricular relaxation ESV: End Systolic Volume Volume of blood in ventricle at the end of ventricular contraction Cardiac Output + or – incre chronot cont ase or ropes c racti a lity, decreas n resp e ectiv ely e n i n creas a ot ro pes c tractility, in on + or – ecrease c ely or d espectiv r Figure X: Average CO (QT) for adult is 5L/min. 35 Ejection fraction Ejection fraction is a simple measurement of ventricular performance and describes the fraction of end-diastolic volume ejected from the ventricle during systole: Example. A healthy man of average size is found to have a resting end- diastolic volume of 140 mL and a resting SV of 80 mL: 36 Heart Rate Normative heart rate 60 – 90 bpm Tachycardia — resting adult heart rate > 100 bpm – Stress, anxiety, drugs, heart disease, or fever – Loss of blood or damage to myocardium Bradycardia — resting adult heart rate < 60 bpm – In sleep, low body temperature, and endurance-trained athletes ANS does not initiate the heartbeat, but modulates rhythm and force Cardiac centers in medulla oblongata initiate autonomic output to the heart Several chemicals affect heart rate: 1. Autonomic neurotransmitters (NE and Ach) 2. Blood-borne adrenal catecholamines (NE and epinephrine) are potent cardiac stimulants 3. Potassium and calcium (can increase or decrease HR depending on concentrations of chemical) Sympathetic postganglionic fibers are adrenergic: – release norepinephrine (NE) – Causes depolarization of SA node – By accelerating both contraction and relaxation, NE (via cAMP) can increase heart rate as high as 230 bpm Parasympathetic vagus nerves have cholinergic, inhibitory effects on SA and AV nodes – Acetylcholine (ACh) binds to muscarinic receptors – Opens gates in the nodal cells, causing hyperpolarization – Cells fire less frequently, heart rate slows down – Without influence from cardiac centers, the heart has an intrinsic firing rate of 100 bpm – 37 Vagal tone—holds down the heart rate to 70 to 80 bpm at rest 12A: Electrophysiology “Chronotro pic effects” Figure 12.5: Sinoatrial (SA) nodal potential as a function of time. (b) Representation of a normal pacemaker potential. (a) Effect of NE (c) Effect of ACh The more rapidly rising phase 4 in the presence of NE results from enhanced Na+ permeability. The hyperpolarization and slower rise in phase 4 in the presence of ACh result from decreased Na+ permeability and increased K+ permeability, as a result of the opening of ACh-activated K+ channels. 38 The Frank-Starling principle Describes the relationship between SV and end-diastolic volume Increased diastolic filling produces greater stretch of heart muscle, resulting in a larger SV by two mechanisms: i. Optimizing overlap between the thin and thick muscle filaments. ii. Increased sensitivity of troponin C to Ca2+. As a result, end-diastolic volume is the most important determinant of SV in the healthy heart. Another result of the Frank-Starling principle is that left and right heart outputs are equalized since output of one side becomes the venous return of the other side. Figure 4–23. Frank-Starling relationship. End-diastolic volume is the most important determinant of stroke volume. Increased contractility of ventricular muscle produces a left shift in the Frank-Starling relationship; decreased contractility produces a right shift. 39 Stroke volume & Preload Stroke volume is determined by three factors: 1. Ventricular preload is the end-diastolic volume created by venous return. 2. Ventricular afterload is the sum of factors that oppose ejection of blood during systole. 3. Contractility is the intrinsic vigor of muscle contraction related to the biochemical state of the cell. In a healthy heart, increasing preload results in increased SV (Figure 4-24). Preload is enhanced by several factors: 4. Increased blood volume. 5. Rhythmic skeletal muscle contraction, which propels blood toward the heart due to the presence of one-way valves in veins = increased venous return. 6. Deep inspiration, which decreases intrathoracic pressure and increases abdominal pressure, promoting venous return to the thorax. 7. Atrial systole. 8. Venoconstriction, which reduces venous pooling and promotes the return of blood to the central circulation. Figure 4–24. Effect of increased preload on ventricular performance. A. Increase in the end-diastolic volume from point X to point Y increases stroke volume due to greater force of ventricular contraction via the Frank-Starling mechanism. B. B. Higher preload moves the end-diastolic pressure-volume point to the right from point X to point Y. The increased left ventricular systolic pressure and stroke volume result from greater force of ventricular contraction. 40 Afterload The major component of afterload is normally the resistance to blood flow created by small muscular arteries and arterioles. Aortic diastolic blood pressure is often used as an index of afterload because it is created by blood flow through vascular resistance. Other sources of afterload could be low compliance (stiffness) of the ventricle or great vessels, or stenosis of the semilunar valves. Figure 4–25. Effect of increased afterload on ventricular performance. Dashed lines indicate the afterload slope, which becomes steeper when the ventricle develops more pressure but delivers a smaller stroke volume, reflecting higher impedance opposing blood flow (increased afterload). 41 Contractility - A change in contraction force independent of preload or afterload. Figure 4–26. Representations of increased ventricular contractility. A. Increased velocity of muscle shortening at zero muscle load (Vmax) in isolated muscle. B. Increased rate of pressure development in the left ventricle (dP/dt). C. Left shift of Frank-Starling relation. D. Elevation of the end-systolic pressure-volume point. 42 13C: Myocardial Performance Inotropes Agents that increase contractility are called positive inotropes, and those that decrease contractility are called negative inotropes. At any given combination of preload and afterload, isometric force generation of cardiac muscle is increased by positive inotropic stimuli and decreased by negative inotropic stimuli Figure 13.7: The effects of changes in inotropic state on isotonic contractions in the heart. Catecholamines are the most important physiologic examples of positive inotropes. Catecholamines occupy myocardial β1- adrenoceptors, causing the generation of intracellular cyclic adenosine monophosphate (cAMP) and activation of protein kinase A (see next slide) Figures X: Inotropic agents 43 Intracellular inotropic mechanisms 13A: Exc-Con Coupling Force of contraction in cardiac muscle can be altered by changes in intracellular calcium concentration. Recall normal physiology - calcium-induced calcium release: Table 4–4. Mechanisms of Increased Myocardial Contractility Following β-Adrenergic Stimulation Figure 13.2: Handling of calcium in a cardiac muscle cell. 44 ANS effect on CO Summary = limited effect of PNS on SV Figures X: ANS and CO 45 CARDIAC OUTPUT SUMMARY 13E: Cardiac Output TABLE 13.1: Factors Influencing Cardiac Output 46