Lecture 7: The Cerebellar Circuit PDF
Document Details
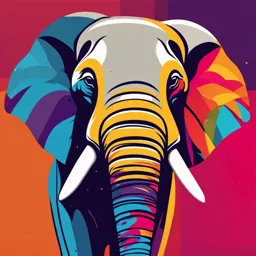
Uploaded by UnabashedLogic
Tags
Summary
This document provides lecture notes on the cerebellar circuit, covering its structure, function, and role in motor learning and cognitive functions. The notes detail how the cerebellum interacts with other parts of the brain and how damage to it can affect motor control. The information involves neuroanatomy and physiology concepts.
Full Transcript
Lecture 7: The Cerebellar Circuit Intro: ● Damage or loss of the cerebellum led to deficits in motor coordination ● Does not lead to paralysis, loss of sensation, or an inability to understand the nature of a task; rather → inability to perform movements well ● Cerebellum is proposed to play a criti...
Lecture 7: The Cerebellar Circuit Intro: ● Damage or loss of the cerebellum led to deficits in motor coordination ● Does not lead to paralysis, loss of sensation, or an inability to understand the nature of a task; rather → inability to perform movements well ● Cerebellum is proposed to play a critical role in the learning & execution of both voluntary and certain reflex movements Slide 2: ● Cerebellum is a central structure deeply integrated into major loops with the cerebral cortex, BS & sc ● Unique capability to act as a forward controller of movements, by predicting system states based on contextual (sensory) information & previous memory ● Operates as a feedforward controller learning to predict the precise timing of correlated motor events ● Role: almost every aspect of motor activities by performing feedback & feedforward input: ○ Maintenance of balance & posture ○ Coordination of voluntary movements ○ Motor learning ○ Cognitive functions that are related to motor functions ● In the scheme, the cerebral cortex sends the cerebellum a copy of motor commands (efference copy), which are then elaborated by cerebellar internal forward model to predict the sensory consequences of movement. ● Then, the cerebellum compares the sensory predictions (based on what the cerebral cortex has sent) to the actual sensory feedback from the periphery ● In the presence of errors (deviations from prediction), the cerebellum emits corrective signals that can affect movement directly (through the BS) or change the motor plan itself (through the cortex) ● In the presence of persistent deviations from prediction, it learns to modify the internal forward model through plasticity Slide 3: Functional Organization of the cerebellum: ● The figures show the 3 parts and the output & input to these parts and the somatotopic maps: ○ Vestibulocerebellum → flocculonodular lobe ○ Spinocerebellum → vermis & paravermis ○ Cerebrocerebellum → hemispheres ● Cerebellum is connected to the homolateral hemibody & to the contralateral cerebral cortex ● Therefore, the cerebellum exerts a homolateral control of movements ● Cerebellum is organized in cerebellar modules ● A module is a conglomerate of several nonadjacent parasagittal bands of Purkinje cells projecting to specific areas of deep cerebellar nuclei (DCN) & gating segregated projections from inferior olivary nucleus ● A summary of the cerebellar input & output: ○ Flocculonodular lobe: sensory inputs arrive from the vestibular system (8th CN), after projecting homolateral to vestibular nucleus (VN) & then to cerebellum (in addition some fibers reach the cerebellum directly). The output occurs through VN generating the medial (bilateral) & lateral (homolateral) vestibulospinal tracts. Axons in this tract then adjust the muscle tone to match the information from the vestibular system. ○ Cerebellar vermis: sensory inputs arrive from specific senses, face & homolateral spinocerebellar systems. The output occurs through the fastigial nucleus (FN) that projects primarily to VN & reticular nucleus (RetN), both homo- & contralateral. Fibers also ascend through the contralateral thalamocortical tract integrating into the ventral corticospinal system controlling VN & RetN; therefore the axial & proximal limb muscles. Visuo-vestibular interactions control ocular reflexes. ○ Cerebellar hemispheres, pars intermedia: receives sensory inputs mostly from spinocerebellar system. The output occurs through interpositus nucleus (IN) (made of nucleus globosus & embliformis) projecting to red nucleus (RN), mostly homolateral. Fibers also ascend through the contralateral thalamocortical tract integrating into the lateral corticospinal system controlling RN & therefore the (mostly distal) limb muscles. ○ Cerebellar hemispheres, pars lateralis: receives inputs mostly from the corticopontocerebellar pathway. The output occurs exclusively through DCN projecting to the contralateral thalamocortical tract. In addition to taking part in homolateral distal limb control through the lateral corticospinal system, the cerebrocerebellum is involved in executive functions & cognitive control. Slide 4: Examples of cerebellar functions: ● Cerebellar lesions cause a movement incoordination syndrome called ataxia ● It is also involved in cognition & executive control, which disturbances cause dyslexia & autism ● Control of muscle contraction by the cerebellum. The cerebellum regulates timing & gain of contraction in agonist & antagonist muscles through an anticipatory (feedforward) action that damps oscillations caused by system perturbations. ● Finger-pointing test: a normal subject correctly moves the fingertip along a nearly straight trajectory, with cerebellar ataxia the movement is delayed & trajectory becomes irregular ● Evoked movement: movement of a handle controlled by a motor extends the forearm stretching the biceps. In response, the biceps contracts bringing the handle back to its initial position, then the triceps contracts terminating the movement. ○ With an ataxic pt, there is no coordination between flexors & extensors and the timing is random ● Voluntary movement: voluntary flexion of the forearm starts with biceps contraction & triceps relaxation. Then the movement terminates due to triceps contraction. The functional inactivation of DCN (by cooling) causes remarkable ↑ of oscillations due to a lack of control on agonist & antagonist muscles. ● It dynamically regulates discharge of BS nuclei (RetN, VN, RN) during leg swing/stance phases of walking ○ So if it got damaged ie random contractions & relaxations → they have to walk slowly as the feedforward mechanism is lost ● The specific patterns of neuronal discharge in these nuclei are abolished by cerebellar ablation. Slide 5: cerebellar cortex layers: ● The Purkinje cell (PC) dendritic tree extends along the transverse axis of the folium, but only in the longitudinal direction, like a pancake that lies in a plane parallel to the transverse folial axis. ● A set of PCs dendritic trees are a stack of pancakes, which runs along the longitudinal folial axis. ● The dendritic trees' stellate & basket cells are oriented similar to PC dendritic tree. ● They form synapses with Purkinje cells ● Climbing fibers make connections with 1 or 2 purkinje cells but make hundreds of synapses with a single purkinje cell ● One parallel fiber on the purkinje cell can make synapses with hundreds of purkinje but only one or two with the same Purkinje ● This means that Input of one CF to a purkinje cell is much more than that of a parallel fiber Slide 6: afferent & efferent system: ● Only two afferent systems get to the cerebellum and only one efferent system leaves the cortex ● The signals entering the cerebellum through the mossy fibers are processed in the granular layer & transmitted to Purkinje cells, while a collateral pathway activates DCN ● Purkinje cells in turn inhibit DCN, so that the cerebellar cortex operates as a side loop controlling DCN Mossy fibers: Climbing fibers (CF): ● Excitatory, convey exteroceptive & proprioceptive information ● Form at least two somatotopic maps (complicated mosaics) of the body across the cerebellar cortex. ● Send collaterals to the cerebellar nuclei. ● The single largest source of mossy fibers comes from the basilar pontine nuclei, which serve to relay information from areas throughout much of the cerebral cortex. ● Provide collaterals to the cerebellar nuclei before heading up to cortex ● In sum, via the mossy fiber system, the cerebellum receives a wide variety of sensory information ● Olivary neurons are almost all projection cells; axons leave the nucleus without giving off collaterals ● Olivocerebellar axons are excitatory & send collaterals to DCN ● They form a special synaptic arrangement with Purkinje cells: each Purkinje cell receives input from a single climbing fiber, which “climbs” up its proximal dendrites ● Makes hundreds of excitatory synapses with Purkinje cells dendrites ● Each olivary axon will branch to form about 10 to 15 CFs Slide 7: Parallel fibers (PF) make synapses with 100s of PCs in a row: ● Stellate & basket cells are GABAergic interneurons, which inhibit PC located adjacent to (via stellate synapses) or at some distance from (basket synapses) the row of activated PF ● Basket & stellate cells do lateral inhibition so the signal is focused in the center & we can isolate a specific row of PC to activate & not activate the others ● So, they can define a central row, or beam, of an excited PC. ● Again, a single PF will pass through more than 100 PC dendritic trees & interneuron dendrites; however, it has the chance to make only one or two synapses with any cell bcz it crosses through the short dimension of the dendritic tree. Conversely, a given PC receives synapses from ~ 200,000 PF Slide 8: The Golgi cells are inhibitory interneurons: ● Thus, they participate in both feedback (when excited by PF) & feedforward (when excited by mossy fibers) inhibitory loops that control activity in the mossy fiber (MF) — PF pathway to the PC ● Golgi cells (GoC) are excited by mossy & CF and by granule cell axons (PF) & inhibited by basket, stellate & PC axon collaterals. ● They in turn inhibit granule cells ● In the granular layer, granule cells receive excitatory inputs from the MF and are inhibited by GoC ● The synaptic contacts among granule cell (GrC) dendrites, MF terminals & GoC axon & dendrites are enwrapped into a glial sheet, originating a peculiar anatomical structure known as cerebellar glomerulus. ● Each glomerulus is characterized by one MF rosette & several granule cell & GoC dendrites, as well as GoC axons. ● Each granule cell dendrite contacts different glomeruli, receiving inputs from different MF ● GrC axon ascends in the molecular layer, where it originates the PF, that forms excitatory synapses on GoC dendrites. ● MF terminals contact granule cell dendrites & GoC → inhibit granule cells in a feedforward inhibitory loop ie nothing is transmitted to PC ● PF originating from granule cell axons, activates GoC, giving rise to a feedback inhibition on granule cells Slide 9: Climbing Fibers (CF): ● Arise from neurons of the inferior olivary nucleus in the brainstem & mono-innervate Purkinje cells (PC) ● Convey instruction (teaching) signals to PCs and cerebellar circuit ● The multilayered afferent fibers from: locus coeruleus (NA), raphe nuclei (5-HT), hypothalamus (histaminergic:HIST): ○ ↓ spontaneous discharge rates of PCs ○ Alter responsiveness of PCs to excitation by CF and by the PF Slide 10: Summary of network connectivity and neurons of cerebellum 1. Divergence of mossy fibers on to different cell types ○ Formation of multiple glomeruli per mossy fiber ○ Multiple inputs on to the same granule cell but different inputs on each granule cell dendrite 2. Glomerular integration ○ Cerebellar glomerulus contains a mossy fiber terminal as well as Golgi cell axonal terminals and dendrites 3. Feedforward inhibitory loops ○ Pass through the mossy fiber (mf) → Golgi cell (GoC) → granule cell (GrC) circuit 4. Feedback inhibitory loops ○ Pass through the mf → granular cell (GC) → GoC → GrC circuit 5. GrCs activate GoCs both on basal dendrites and apical dendrites 6. GoC → GoC reciprocal inhibition through reciprocal synapses 7. GoC → GoC communication through gap junctions 8. Unipolar brush cell (UBC) pathway: mf → UBC → GrC (only in vestibulocerebellum) 9. Lugaro cell (LC) pathway: mf → LC → GoC 10. Multiple neuron types in deep cerebellar nuclei (DCN) 11.Gap junctions in the IO ● Simple spike: occurs when PF excite PC (long frequency) ● Complex spike: occur when CF excite PC Slide 11: Organization of the cerebellar cortex, cerebellar nuclei, & IO ● Functionally organized as 2 loops: ○ Cerebellar cortex has a deep excitatory loop, which is followed by a longer cortical inhibitory loop ■ How quickly the inhibitory loop follow the excitatory loop depends on the error that could happen in the system or on the status & activity of other inhibitory interneurons in the cortex ○ Each longitudinal strip of the cortex targets a specific region of the cerebellar nuclei ■ Input (from MF & CF) → cerebellar cortex → output goes to cerebellar nuclei → to different parts of the motor system ● GABAergic (inhibitory) output ● NonGABAergic (excitatory) ○ GABAergic cells (from the deep nuclei) → project back to specific part of IO → form a (-) feedback loop to one of cerebellum’s principal afferent sources. ■ Specific part of IO receives input from GABAergic cells & overlies with longitudinal strips of cortex (receiving CFs) Slide 12: The excitatory (nonGABAergic) nuclear cells from the cerebellum project to a variety of targets from the SC to the thalamus. The inhibitory cells project back to IO ● Each nucleus gives rise to crossed ascending & descending projections that leave the cerebellum via the superior cerebellar peduncle ● Ascending cerebellar projections target: ○ Red nucleus ○ Superior colliculus ○ VL nucleus of the thalamus ● Descending fibers target: ○ Basilar pontine nuclei ○ Several reticular nuclei ● The overall feedback pathway between the red nucleus & cerebellum: ○ Red nucleus projects to the cerebellum via a synapse in the IO nucleus (rubro-olivary projections & olivocerebellar fibers) ○ Cerebellum projects back to the red nucleus from the interposed nucleus (includes a PC axonal input to interposed nuclei) ○ The relationship of this circuit with the SC is indicated by the presence of the rubrospinal tract Slide 13: IO/CF & Motor Learning ● Motor learning occurs through synaptic plasticity at multiple synapses in the cerebellum. ● Critically depends on complex spikes induced by the CFs which produce a prolonged depression in parallel fiber synaptic efficacy. ● Motor-learning hypothesis: states that the parallel fiber system (simple spikes) are involved in generating ongoing movement ● Mismatch btwn intended & actual movement → activates IO → results in complex spikes → lead to long-term depression (LTD) of active parallel fiber synapses ● LTD induction: ○ Glutamate (released from parallel fiber terminals) binds to mGluR1 at the perisynaptic region of PC dendritic spines ○ Gq & PLCβ4 molecular cascade is activated in the spines → DG & IP3 are produced ○ IP3 triggers Ca2+ release from internal stores in the spines ○ CF-induced depolarization of PC dendrites → VGCC open → Ca2+ influx ○ ↑ levels of local Ca2+ in the spines → activate PKC & DG ○ PKC → phosphorylates AMPA receptor → phosphorylated AMPA receptors are internalized ○ Extracellular Ca2+ and/or ambient GABA split over from neighboring inhibitory synapses activates GABABR → enhances mGluR1 signaling (by direct interaction and/or through the action of Gi/o protein) ● Main point: more firing from CF → less AMPA receptors → nothing will happen to PC (ie no inhibition of DCN) → this is LTD Slide 14: Loss of cerebellar activity (e.g. unilateral infarctions that climbing fibers) affect motor learning, as revealed by inability to readjust eye- hand coordination during adaptation to prism glasses. ● Prism adaptation experiment: ○ Before prisms → gaze toward target → throw toward target ○ Just after wearing prisms → gaze along the bent light path AWAY from target → throw in direction of gaze AWAY from target ○ Still wearing prisms & after adapting to them → gaze along the bent light path AWAY from the target → throw TOWARD target ○ Just after taking off prisms → gaze toward target → adapted throw is to the right of the direction of gaze & to the right of target ○ After disadapting the gaze-throw coordination → gaze directed to target → throw in the direction of gaze TOWARD target as originally ● Data during & after prism use have been fit with exponential curves. ● Adaptation fails in pts with unilateral infarctions in PICA & involves inferior cerebellar peduncle (IO CF) and/or inferior lateral posterior cerebellar cortex. Slide 15: Motor learning in the cerebellum ● Motor learning theory predicts the neurophysiologic mechanism of adaptation of the vestibulo-ocular reflex ● Visual errors (ex: caused by magnifying goggles): ○ → relayed to IO → instructs some PCs through CF to change synaptic weights at the PF synapses conveying contextual vestibular information → LTD learning ○ MF → some convey vestibular & some efferent copies of eye velocity & retinal slip → change in PC activation → plastic changes at vestibular nucleus (VN) synapses → instruction line would go from PC to VN Slide 16: Clinical manifestations of damage to the cerebellum ● Vestibulocerebellum: ○ Nystagmus ○ Romberg’s test: determines balance issues related to the function of dorsal column by removing the visual and vestibular components that contribute to maintaining balance (assess impaired proprioception in sensory ataxia) ● Spinocerebellum: ○ Drunk sailor’s gait (truncal ataxia) ● Cerebrocerebellum: ○ Pass-pointing in finger-to-nose test (which assess coordination)