Lecture 5: Introduction to Nuclear System and Operation (Khalifa University)
Document Details
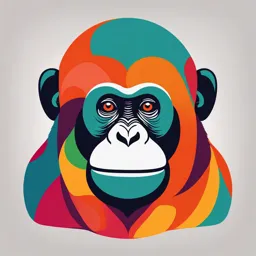
Uploaded by WellBacklitLivermorium8013
Khalifa University
Dr. Ahmed Alkaabi
Tags
Related
- PWR Overview PDF
- NUCE 402: Introduction to Nuclear System and Operation Lecture 6 PDF
- Nuclear Power, OntarioTech, MECE3260U, PDF
- NUCE 402: Introduction to Nuclear System and Operation Lecture Notes PDF
- NUCE 402: Introduction to Nuclear System and Operation Lecture Notes PDF
- NUCE 402: Introduction to Nuclear System and Operation Lecture 4 PDF
Summary
This lecture from Khalifa University covers heat removal from nuclear reactors using convection. Basic concepts such as Newton's law of cooling, thermal resistance, and heat transfer coefficients are introduced. Equations and calculations related to these concepts are also presented.
Full Transcript
NUCE 402: Introduction to Nuclear System and Operation Chapter 3.1 (Heat Removal from Nuclear Reactors – Convection) Dr. Ahmed Alkaabi Previous Lectures Conduction Fourier’s law q kT From energy balance...
NUCE 402: Introduction to Nuclear System and Operation Chapter 3.1 (Heat Removal from Nuclear Reactors – Convection) Dr. Ahmed Alkaabi Previous Lectures Conduction Fourier’s law q kT From energy balance q Steady state heat 2T 0 k conduction equation Solutions d 2T q q 2 0 T x Tm Plate-type dx 2 kf 2k f d 2T xa 0 T Ts (Ts Tc ) dx 2 b Tm Tc a b q Tm Tc q( ) a b 2k f A k c A Cylindrical 2k f A k c A 1 Tm Ts q( ) 4k f H T T Tm ( z ) Tc ( z ) q m c q( z ) ln( 1 b / a ) R f Rc generalization 2 (a b) H ( R f Rc ) Ts Tc q kc 2H 2 Convection Newton’s law of cooling Transfer of heat from a heated solid to a moving fluid q h(Tc Tb ) Heat flux Heat transfer coefficient Btu/hr-ft2 Btu/hr-ft2-oF Heat transfer coefficients Function of liquid, flow types, coolant temp., etc. Typical values in power reactor Light and heavy water: 5,000~8,000 Btu/hr-ft2-oF 25~45 kW/m2-K Gases: 10~100 Btu/hr-ft2-oF 0.055~0.55 kW/m2-K Liquid sodium: 4,000~50,000 Btu/hr-ft2-oF 20~300 kW/m2-K Total rate of heat flow across A 1 q qA hA(Tc Tb ) Introduce the thermal resistance, Rh Rh hA (Tc Tb ) q Rh 3 Thermal Resistance Total thermal resistance For plate-type fuel elements Tm Tc Tm Tb q q a b a b 1 Tb 2k f A k c A 2k f A kc A hA a b 1 then R 2k f A kc A hA For clad cylindrical fuel elements Tm Tb Tm Tc Tm Tb q q q R 1 ln( 1 b / a ) 1 ln( 1 b / a) 1 4k f H 2kc H 4k f H 2kc H hA 1 ln( 1 b / a) 1 then R where A 2 (a b) H 4k f H 2kc H hA For space dependent heat source T ( z ) Tb ( z ) Tm ( z ) Tb ( z ) q( z ) m q( z ) R 2 (a b) H ( R f Rc Rh ) Tc ( z ) Tb ( z ) q( z ) q( z) h(Tc ( z) Tb ( z)) 2 (a b) HRh 4 Temperature along the Coolant Channel Coolant channel A coolant volume associated with a single fuel rod Heat generated within the fuel is Ac transferred Coolant temperature will rise Consider a coolant slab of t = dz Volume of coolant: Ac dz Ac : c x area of fuel channel Mass of coolant: Ac dz Amount of heat needed to increase dTb: Ac dz c p dTb 1 Rate of heat addition to increase dTb = dq: Ac dz c p dTb dt Therefore, Ac c p dTb dq c p dTb qAf dz => Coolant flow rate z where, q qmax cos( ) for fuel rod at the center H 5 Temperature along the Coolant Channel For the central fuel rod Bulk coolant temperature qA f dq c p dTb qAf dz dTb dz z c p where, q qmax cos( ) H Tb Tb Tb 0 Tb q’’’ z A f qmax z cos( )dz H / 2 c H p Vf Therefore, the maximum coolant temp. A f H qmax z Tb Tb 0 1 sin( ) V f 2qmax c p H Tb ,max Tb 0 Cc c p Tb0 Cladding temperature Ac 1 dq dq qAf dz qdAc qCc dz h(Tc Tb )Cc dz Tc Tb Substituting Tb and the integration hCc dz V f qmax z qmax A f z qA f Tc Tb 0 1 sin( ) cos( ) c p H hCc H hCc A f qmax z V f qmax z z Tc Tb cos( ) V f cos( ) cos( ) Rh qmax hCc H hA H H 6 Temperature along the Coolant Channel z z rewrite V f cos( Tc Tb Rh qmax A f H cos( ) Rh qmax ) H H For fuel rod central temp. =q Tm Tb z A f H cos( ) qmax Substituting Tb and the integration R H Total thermal resistance V f qmax z z Tm Tb 0 1 sin( ) q V R cos( ) c p max f H H Factors affecting maximum fuel temp. Maximum heat generation at mid-point Largest heat transfer q’’’ Slow decreasing away from the mid-point Rising bulk temperature along z Tm must increase to provide q” => Maximum Tm occurs above the mid-point 7 Temperature along the Coolant Channel Locations of maximum temp. H zc ,max cot 1 (c p Rh ) H z m ,max cot 1 (c p R ) Values of maximum temp. 1 1 2 V f Rh Tc ,max Tb 0 qmax c p Rh 1 1 2 V f R Tm ,max Tb 0 qmax c p R Limitations: - qualitative simple power distribution constant k and h does not account for boiling - not applicable to liquid metal coolants If thermal resistance is small, the maximum temp. can be lowered increase conductivity of fuel/cladding increase heat transfer coefficient of coolant 8 Heat Transfer Coefficients Heat removal by coolant Conduction When every portion of fluid moves parallel to the channel => Laminar flow No net motion of fluid in radial direction => conduction dominate Convection When there are significant velocity fluctuation in radial direction => Turbulent flow Heat carried away from the wall to the bulk of liquid => convection dominate In NPP Coolant is pumped Forced convection => turbulent flow More or less uniform bulk temperature Rapid drop of coolant temperature in the vicinity of fuel 9 Heat Transfer Coefficients Reynolds number Useful in characterizing the flow of a fluid Dimensionless parameter De Re Fluid viscosity lb/hr-ft, or poise Equivalent diameter (g/cm-sec) c x area of coolant channel De 4 wetted perimeter of coolant channel s 2 a 2 s 2 a 2 Condition for turbulent (typical) De 4 2 2a a Laminar: Re < 2000 Mixture: 2000 < Re < 10000 High Re => more turbulent => large h Turbulent: Re > 10000 => high rate of heat transfer to coolant Values of heat transfer coefficients Determined by experiments Provided as experimental correlations Need other dimensionless parameters hDe cp Nusselt number Nu Prandtl number Pr k k 10 Heat Transfer Coefficients For flow through long straight channel under turbulent conditions k Nu C Re Pr m n h( )C Re m Pr n De For long, straight, circular tube Limitations: - valid for only for the reference k h 0.023( ) Re 0.8 Pr 0.4 temperatures De - not applicable when conduction become non-negligible Dittus-Boelter Eq. - significant error if channel deviate significantly from circular shape Liq. Na: 46.4 Btu/hr-ft-oF For liquid metals Water: 0.381 Btu/hr-ft-oF Large conductivity He: 0.115 Btu/hr-ft-oF Less steep temp. gradient in coolant Correlation for Liq. Metal through a hexagonal lattice of rods Nu 0.66 3.126(s / d ) 1.184(s / d ) 2 0.0155(Pe)0.86 Valid for s/d > 1.35 Ratio of lattice pitch Pe Re Pr to rod diameter Peclet number 11 Summary Newton’s law of cooling q h(Tc Tb ) Total thermal resistance Tb a b 1 R 2k f A kc A hA Tm Tb q 1 ln( 1 b / a) 1 R R 4k f H 2kc H hA Temp. along the channel z V f V f cos( Tc Tb Rh qmax ) qmax z H Tb Tb 0 1 sin( ) c p H z V f cos( ) Tm Tb Rqmax H Heat transfer coefficients Dimensionless numbers High Re value for turbulent flow k h 0.023( ) Re 0.8 Pr 0.4 D hDe cp De Re e Nu Pr k k Dittus-Boelter Eq. 12