Lecture 5: Metabolism and Energy II: Oxidative Phosphorylation PDF
Document Details
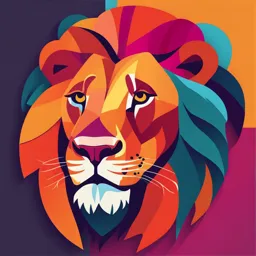
Uploaded by UnboundMaracas
Hong Kong Metropolitan University
Dr. Sophie Shi Ling
Tags
Summary
This document is a lecture, presenting the key points of oxidative phosphorylation. It details the process, including the electron transport chain, chemiosmosis, and the roles of various cellular components. The document was produced at Hong Kong Metropolitan University by Dr. Sophie Shi Ling.
Full Transcript
Lecture 5 Metabolism and Energy II: Oxidative Phosphorylation Dr. Sophie SHI Ling [email protected] Department of Applied Science School of Science and Technology 1 Contents I. Introduction to Oxidative Phosphorylation II. Electron Transpor...
Lecture 5 Metabolism and Energy II: Oxidative Phosphorylation Dr. Sophie SHI Ling [email protected] Department of Applied Science School of Science and Technology 1 Contents I. Introduction to Oxidative Phosphorylation II. Electron Transport Chain III. Chemiosmosis 2 Review —— Cellular Respiration Ø Key Stages of Cellular Respiration 1. Glycolysis Location: Cytoplasm Process: Glucose is broken down into two molecules of pyruvate 2. Pyruvate Oxidation Location: Mitochondrial matrix Process: Each pyruvate is converted to Acetyl-CoA, producing NADH and releasing CO2 3. TCA Cycle (Krebs Cycle) Location: Mitochondrial matrix TCA cycle Process: Acetyl-CoA is oxidized, generating NADH, FADH2, and GTP (or ATP) 4. Oxidative Phosphorylation Location: Inner mitochondrial membrane Process: Electrons from NADH and FADH2 are transferred through the electron transport chain (ETC), creating a proton gradient that drives ATP synthesis Review —— Cellular Respiration Ø Importance of Cellular Respiration q Primary Energy Source Provides ATP, the energy currency of the cell, essential for all cellular activities Supports processes such as muscle contraction, nerve impulse transmission, and biosynthesis q Metabolic Pathway Integration Central to various metabolic pathways, linking catabolism and anabolism q Oxygen Utilization Utilizes oxygen efficiently, allowing for high-energy yield compared to anaerobic (absence of oxygen) processes Essential for aerobic (require oxygen) organisms, including humans, to sustain energy needs q Regulation of Metabolism Regulates energy balance and metabolic homeostasis in response to dietary intake and energy demands Helps maintain blood glucose levels and metabolic flexibility Review —— Mitochondria Structure Mitochondria are vital organelles that act as the central hub for energy production and metabolic processes in eukaryotic cells q Structure of Mitochondria 1. Outer Membrane Smooth and permeable Contains proteins called porins that allow the passage of small molecules and ions 2. Inner Membrane Highly folded into structures called cristae Contains proteins involved in the electron transport chain and ATP synthesis Impermeable to most ions and small molecules, creating a distinct environment 3. Intermembrane Space The space between the outer and inner membranes Plays a role in the proton gradient essential for ATP production 4. Mitochondrial Matrix Enclosed by the inner membrane Contains enzymes for the TCA cycle, mitochondrial DNA (mtDNA), ribosomes, and various metabolic enzymes Site of key metabolic processes, including the TCA cycle and fatty acid oxidation Review —— Pyruvate Oxidation The oxidative decarboxylation of pyruvate is catalyzed by pyruvate dehydrogenase Pyruvate dehydrogenase is a multienzyme complex: i. Pyruvate dehydrogenase (E1) ii. Dihydrolipoyl transacetylase (E2) iii. Dihydrolipoyl dehydrogenase (E3) Bind to three cofactors: thiamin pyrophosphate (TPP), lipoamide, and flavin adenine dinucleotide (FAD) Three step reactions: v Pyruvate dehydrogenase is strictly regulated Review —— Summary of TCA cycle Ø For each acetyl-CoA molecule, the products of the TCA cycle are: Two CO2, three NADH molecules, one FADH2 molecule, and one GTP/ATP molecule Oxidative Phosphorylation Ø What is oxidative phosphorylation? The process by which ATP is produced through the transfer of electrons in the electron transport chain (ETC) and the creation of a proton gradient Oxidative: The oxidation reactions that take place in the electron transport chain (ETC) Phosphorylation: The addition of a phosphate group (Pi) to ADP to form adenosine triphosphate (ATP) Ø Location Occurs in the inner mitochondrial membrane Ø Components 1. Electron Transport Chain (ETC) Transfers electrons from electron carriers (NADH and FADH₂) to oxygen Composed of four main protein complexes (I, II, III, IV) and mobile carriers Coenzyme Q (ubiquinone) and cytochrome c Electrons move through the protein complexes, releasing energy This energy is used to pump protons (H⁺ ions) from the mitochondrial matrix into the intermembrane space, creating a proton gradient 2. Chemiosmosis (ATP Synthesis) Utilizes the proton gradient established by the ETC to synthesize ATP ATP synthesis is catalyzed by the enzyme ATP synthase Oxidative Phosphorylation Ø Process of Oxidative Phosphorylation 1. Electron Donation NADH and FADH₂ generated from glycolysis and TCA cycle donate electrons to the electron transport chain (ETC) 2. Electron Transport Electrons travel through four protein complexes (I-IV) embedded in the inner mitochondrial membrane Energy released during this transfer is used to pump protons (H⁺) from the mitochondrial matrix into the intermembrane space Oxidative Phosphorylation Ø Process of Oxidative Phosphorylation 3. Proton Gradient Creation The pumping of protons creates a proton gradient across the inner mitochondrial membrane, establishing a chemiosmotic potential (proton motive force) 4. ATP Synthesis via Chemiosmosis Protons flow back into the matrix through ATP synthase The flow drives the production of ATP 5. Final Electron Acceptance Electrons are transferred to molecular oxygen (O₂) at Complex IV, forming water (H₂O) as a byproduct https://www.youtube.com/watch?v=fHoL-vcMENw Function of Oxidative Phosphorylation 1. ATP Production Primary Role: Generates approximately 26-28 ATP molecules per glucose molecule, providing energy for cellular processes 2. Energy Conversion Converts energy from reduced cofactors (NADH and FADH₂) into chemical energy stored in ATP 3. Cellular Respiration Integration Completes the process of cellular respiration by utilizing oxygen as the final electron acceptor, producing water 4. Metabolic Regulation Regulates key metabolic pathways by responding to changes in cellular energy demands; ATP levels influence various biochemical reactions 5. Heat Generation Some energy is dissipated as heat, aiding in thermoregulation and maintaining body temperature in humans Contents I. Introduction to Oxidative Phosphorylation II. Electron Transport Chain III. Chemiosmosis 12 Overview of electron transport chain (ETC) Ø What is ETC? A series of proteins that transfer electrons through a membrane within mitochondria to form a gradient of protons that drives the creation of ATP Ø Where does ETC occur? In eukaryotes, ETC takes place in the inner membrane of mitochondria Protons are transported from the matrix to the intermembrane space across the inner mitochondrial membrane Ø What are the roles of ETC in cellular respiration? ETC serves as electron carriers in the final stage of cellular respiration ETC generates proton gradients for chemiosmosis (ATP synthesis) Ø What are the components of ETC? Complex I: Type I NADH dehydrogenase Complex II: Succinate dehydrogenase Complex III: Coenzyme Q : cytochrome c – oxidoreductase Complex IV: Cytochrome c Oxidase Complex I: Type I NADH dehydrogenase Ø Basics Also known as: NADH: ubiquinone oxidoreductase, NADH dehydrogenase (ubiquinone) Complex I is the electron acceptor from NADH and the largest complex found in ETC Composition: NADH dehydrogenase, flavin mononucleotide (FMN), iron-sulfur clusters (Fe-S) Ø Function & Characteristics Complex I is the first enzyme in ETC, marks the beginning of ETC Complex I collects the pair of electrons from NADH and passes them to ubiquinone (Coenzyme Q) Ø Reaction NADH + H+ + CoQ + 4H+in→ NAD+ + CoQH2 + 4H+out Ø Electron Transfer q Step 1 2 electrons are transferred from NADH to FMN, and reduce it to FMNH2 q Step 2 The electrons are transferred from FMNH2 to Coenzyme Q and convert it into CoQH2 Complex II: Succinate dehydrogenase Ø Basics Also known as succinate-coenzyme Q reductase The only enzyme that participates in both the citric acid cycle and the electron transport chain Composed of four protein subunits: succinate dehydrogenase (SDHA); succinate dehydrogenase [ubiquinone] iron–sulfur (Fe-S) subunit mitochondrial (SDHB); succinate dehydrogenase complex subunit C (SDHC); and succinate dehydrogenase complex subunit D (SDHD) Ø Function & Characteristics catalyzes the transfer of electrons from other donors like fatty acids and glycerol-3 phosphate to coenzyme Q A parallel electron transport pathway to Complex I Unlike Complex I, No protons are transported to the intermembrane space in Complex II Complex II contributes less energy to the overall ETC Ø Reaction Succinate + FADH2 + CoQ → Fumarate + FAD+ + CoQH2 Ø Electron Transfer q Step 1 Succinate is converted to fumarate, with 2 electrons released q Step 2 Electrons provided by succinate are transferred to FAD, reducing FAD into FADH2 q Step 3 FADH2 passes electrons to coenzyme Q through Fe-S clusters Complex III: Coenzyme Q (Q) : cytochrome c – oxidoreductase Ø Basics Also known as: cytochrome bc1 complex A multi-subunit transmembrane protein encoded by both the mitochondrial and the nuclear genomes Composition: cytochrome b, cytochrome c and a specific Fe-S center Ø Function & Characteristics Complex III is the destination for electrons arriving from both Complex I and Complex II Catalyzes the transfer of electrons from the reduced coenzyme Q to cytochrome c for transport to Complex IV Complex III uses energy released in downhill electron transfers to pump more protons across the inner mitochondrial membrane The proton gradient across the membrane is used to drive ATP formation Ø Reaction CoQH2 + 2 cytochrome c (Fe3+) → CoQ + 2 cytochrome c (Fe2+) + 4H+ Ø Electron Transfer q Q cycle Complex III: Coenzyme Q (Q) : cytochrome c – oxidoreductase Ø Q cycle q First half cycle Two electrons of QH2 are transferred One electron goes to cytochrome c, forming the reduced form of cytochrome c The second electron goes to another Q molecule, producing semi-reduced Q ( Q-) Two protons are released into the intermembrane space q Second half cycle A second QH2 releases its two electrons One electron goes to reduce cytochrome c again The other goes to semi-reduced Q ( Q-) in order to pick up two protons from the matrix The fully reduced QH2 is formed Two protons are pumped into the intermembrane space Complex IV: Cytochrome c Oxidase Ø Basics Complex IV is the last enzyme in ETC Composition: cytochrome a, cytochrome a3, and two copper centers, the CuA and CuB centers Ø Function & Characteristics Catalyzes the transfer of two electrons from cytochrome c to molecular oxygen (O2), reducing it to water Electrons travel from the intermembrane space side to the mitochondrial matrix side, against the charge gradient Ø Reaction 4 cytc c (Fe 2+) + O2+ 4H+ → 4 cytc c (Fe3+) + 2H2O Ø Electron Transfer Four electrons are removed from four molecules of cytochrome c and transferred to molecular oxygen (O2) and four protons, producing two molecules of water Eight protons are removed from the mitochondrial matrix, with four translocated across the membrane, contributing to the proton gradient Electron Transport Chain Steps Ø Step 1: Transfer of electrons from NADH to Coenzyme Q NADH, produced during glycolysis and the citric acid cycle, donates electrons to complex I Complex I transfers the electrons to Coenzyme Q while pumping protons across the inner mitochondrial membrane, creating a proton gradient Ø Step 2: Transfer of electrons from FADH2 to Coenzyme Q The oxidation of succinate to fumarate results in the reduction of FAD to FADH2 The electrons from FADH2 enter the electron transport chain catalyzed by complex II Complex II doesn’t pump any protons across the membrane Ø Step 3: Transfer of electrons from CoQH2 to cytochrome c Complex III receives electrons from Coenzyme Q and transfers them to cytochrome c As electrons are transferred in complex III, protons are pumped across the inner mitochondrial membrane. Cytochrome c accepts electrons from complex III and shuttles them to complex IV Ø Step 4: Transfer of electrons from cytochrome c to molecular oxygen Complex IV receives electrons from cytochrome c and transfers them to molecular oxygen (O2) The transfer of electrons to oxygen leads to the formation of water (H2O) Complex IV also pumps protons across the inner mitochondrial membrane https://www.youtube.com/watch?v=nCr3iCzX4lc Summary of Electron Transport Chain Ø Electron Transfer NADH and FADH₂ donate electrons ü NADH → Complex I two electrons transfer ü FADH₂ → Complex II two electrons transfer Ø Proton Pumps Energy from electron transfer drives proton pumping ü Complex I: Pumps 4 protons (H⁺) ü Complex III: Pumps 4 protons (H⁺) ü Complex IV: Pumps 2 protons (H⁺) NADH: 10 protons pumped into the intermembrane space per 2 electrons transferred FADH2: 6 protons pumped into the intermembrane space per 2 electrons transferred Ø Role of Oxygen Final Electron Acceptor ü Oxygen is reduced at Complex IV ü Accepts 2 electrons and combines with protons to form water (H₂O) ü Requires 4 electrons to reduce 1 O₂ molecule Electron Transport Chain Inhibitors Ø Complex I inhibitor Rotenone: Inhibits the reduction of Coenzyme Q, e.g. Amytal and Halothanes: Inhibits the transfer of electrons from the Fe-S centers to Coenzyme Q Ø Complex II inhibitor Competitive inhibitors with the substrate succinate Examples: carboxin, thenoyltrifluoroacetone and malonate Ø Complex III inhibitor (antibiotics) Antimycin: Inhibits the transfer of electrons from cytochrome b to Coenzyme Q Myxothiazol and stigmatellin: Inhibits the transfer of electrons from reduced form of Coenzyme Q to cytochrome c Ø Complex IV inhibitor Cyanide (CN-) and azide (N3-): block the transfer of electrons from cytochrome a to CuA center Carbon monoxide (CO): binds to the reduced from of cytochrome a3, and prevents electron transfer to O2 Contents I. Introduction to Oxidative Phosphorylation II. Electron Transport Chain III. Chemiosmosis 22 Overview of Chemiosmosis Ø What is chemiosmosis? Chemiosmosis is the movement of ions across a semipermeable membrane bound structure, down their electrochemical gradient An important example is the formation of ATP by the movement of H+ across a membrane during cellular respiration Ø Where does chemiosmosis occur? In eukaryotes, chemiosmosis also occurs in the inner mitochondrial membrane Protons are pumped into the intermembrane space, creating a proton gradient that drives ATP synthesis via ATP synthase Ø What are the roles of chemiosmosis in cellular respiration? Chemiosmosis plays a crucial role in oxidative phosphorylation by enabling ATP production It assists in establishing a proton gradient, converting energy, regulating metabolism, maintaining membrane potential, and facilitating transport processes Proton Motive Force (PMF) Ø Definition The proton motive force is the electrochemical gradient of protons (H⁺ ions) across a biological membrane, primarily the inner mitochondrial membrane in eukaryotes Ø Components Difference in H⁺ concentration (more H⁺ outside than inside) Difference in charge (more positive outside due to H⁺ accumulation) Ø Formation Active transport of protons by complexes I, III, and IV in the ETC Ø Function Drives ATP synthesis via ATP synthase ATP Synthase Ø Definition ATP synthase is a multi-subunit enzyme complex responsible for ATP production using the energy from the proton motive force Ø Structure F₀ Component ü Embedded in the inner mitochondrial membrane ü Forms a channel for protons (H⁺) to flow back into the matrix, driven by proton motive force ü Composed of a ring of c-subunits that rotate upon proton passage F₁ Component ü Projects into the mitochondrial matrix ü Catalyzes the conversion of ADP and inorganic phosphate (Pi) into ATP ü Contains three α and three β subunits that form the catalytic sites Mechanism of ATP Synthesis Ø Process 1. Proton Gradient Formation Electrons are transferred through the electron transport chain (ETC) Protons (H⁺) are pumped from the mitochondrial matrix to the intermembrane space, creating a proton gradient 2. Proton Flow through ATP Synthase Protons flow back into the matrix through the F₀ component of ATP synthase This flow is driven by the gradient and electrochemical potential 3. Rotation of F₀ The influx of protons causes the c-ring in the F₀ component to rotate This mechanical energy is transmitted to the F₁ component 4. ATP Formation The rotational energy induces conformational changes in the F₁ component, facilitating the binding of ADP and inorganic phosphate (Pi) This leads to the synthesis of ATP from ADP and Pi Mechanism of ATP Synthesis https://www.youtube.com/watch?v=k_DQ1FjFuYM P/O Ratio and ATP Production Ø Definition The P/O ratio (phosphate to oxygen ratio) is a measure of the efficiency of oxidative phosphorylation in the electron transport chain (ETC) and ATP synthesis in mitochondria The P/O ratio indicates the number of ATP molecules produced during the transfer of two electrons in the ETC, terminated by reduction of one oxygen atom Ø Typical P/O Ratio q From NADH: Each NADH donates two electrons to the ETC, 10 protons (H+) pumped Typically yielding about 2.5 to 3 ATP per oxygen atom q From FADH2: Each FADH₂ donates two electrons to the ETC, 6 protons (H+) pumped Typically yields about 1.5 to 2 ATP per oxygen atom ATP Yield Ø Total Electron Carriers C6H12O6 + 6 O2 → 6 CO2 + 6 H2O + 30-32 ATP 10 NADH 2 FADH₂ Assuming 2.5 ATP per NADH, 1.5 ATP per FADH2 Ø ATP Yield Glycolysis ü 2 ATP from substrate-level phosphorylation ü 2 NADH yields 3 ATP or 5 ATP Pyruvate Oxidation (Two Pyruvates) ü 2 NADH yields 5 ATP 26-28 ATP TCA Cycle (Two Acetyl-CoA) ü 6 NADH yields 15 ATP v NADH produced in the cytoplasm during glycolysis must be transported into the mitochondria for ATP generation ü 2 FADH₂ yields 3 ATP ü 2 ATP from substrate-level phosphorylation v Two main shuttle systems are involved 1. Glycerol-Phosphate Shuttle Converts NADH to FADH2, enter complex II Total: 30 or 32 ATP 2. Malate-Aspartate Shuttle Transfers electrons directly to NAD+, enter complex I Theoretical Maximum Yield of ATP Ø Total Electron Carriers C6H12O6 + 6 O2 → 6 CO2 + 6 H2O + 36-38 ATP 10 NADH 2 FADH₂ Assuming 3 ATP per NADH, 2 ATP per FADH2 Ø ATP Yield Glycolysis ü 2 ATP from substrate-level phosphorylation ü 2 NADH yields 4 ATP to 6 ATP Pyruvate Oxidation (Two Pyruvates) ü 2 NADH yields 6 ATP 32-34 ATP TCA Cycle (Two Acetyl-CoA) ü 6 NADH yields 18 ATP v NADH produced in the cytoplasm must be transported into ü 2 FADH₂ yields 4 ATP the mitochondria for ATP generation ü 2 ATP from substrate-level phosphorylation v Two main shuttle systems are involved 1. Glycerol-Phosphate Shuttle Converts NADH to FADH2, enter complex II Total: 36 or 38 ATP 2. Malate-Aspartate Shuttle: Transfers electrons directly to NAD+ Coupling of Electron Transport and Chemiosmosis The ETC and chemiosmosis are coupled processes essential for cellular respiration The transfer of electrons through the ETC generates a proton gradient that drives ATP synthesis q Importance of coupling ETC & Chemiosmosis 1. Efficient ATP Production Maximizes ATP yield from nutrients using the proton gradient 2. Energy Transfer Converts energy from electron carriers (NADH, FADH₂) into ATP 3. Regulation of Metabolism Influences metabolic pathways based on cellular energy needs 4. Prevention of Energy Loss Minimizes energy dissipation as heat, conserving energy for ATP synthesis 5. Key Role in Cellular Respiration Essential for converting biochemical energy into usable ATP 6. Mitochondrial Function Maintains mitochondrial integrity; disruptions can lead to decreased ATP and increased reactive oxygen species (ROS) Uncouplers of Oxidative Phosphorylation Ø Definition Uncouplers are substances that disrupt the coupling between the ETC and ATP synthesis Cause protons to flow back into the mitochondrial matrix independently of ATP synthase Ø Mechanism of Action Proton Leakage: Uncouplers create a pathway for protons (H⁺) to re-enter the matrix without generating ATP Dissipation of Proton Gradient: This reduces the proton motive force, leading to decreased ATP production Ø Examples q 2,4-Dinitrophenol (DNP) A chemical compound that transports protons across the mitochondrial membrane Used historically as a weight-loss agent due to increased metabolic rate q Carbonyl Cyanide m-Chlorophenyl Hydrazone (CCCP) A potent chemical uncoupler that collapses the proton gradient, used in research to study mitochondrial function q Uncoupling Proteins (UCPs) Mitochondrial inner membrane proteins that are regulated proton channels or transporters Play important roles in energy metabolism and thermogenesis Ø Effects on Cellular Metabolism Increased Oxygen Consumption: Cells consume more oxygen as the ETC attempts to maintain the proton gradient Heat Production: Energy is released as heat, which can be beneficial (e.g., thermoregulation) or harmful (e.g., overheating) Uncoupling Proteins Protein Location Function Key role in thermogenesis; uncouples oxidative phosphorylation to UCP1 Brown Adipose Tissue (BAT) generate heat Modulates energy metabolism, insulin secretion, and reduces UCP2 Various (brain, pancreas) oxidative stress (ROS) Involved in fatty acid metabolism; protects against oxidative stress; UCP3 Skeletal Muscle, BAT regulates energy homeostasis May protect neurons from oxidative damage; involved in neuronal UCP4 Brain energy metabolism Similar to UCP4; suggested role in neuronal protection and energy UCP5 Brain regulation Heat Generation: Primarily associated with UCP1 Other UCPs: While they can uncouple oxidative phosphorylation, their roles are more focused on metabolic regulation, protection against oxidative stress, and energy homeostasis rather than significant heat generation Oxidative Phosphorylation Disorder Genetic or acquired defects in the electron transport chain (ETC) impair ATP production Caused by mutations in mitochondrial DNA or nuclear DNA affecting ETC complexes q Common Disorders 1. Leigh Syndrome Cause: Genetic mutations affecting mitochondrial function Symptoms: Neurological decline, developmental delays, respiratory distress 2. Mitochondrial Myopathy Cause: Impaired oxidative phosphorylation in muscle tissue Symptoms: Muscle weakness, pain, and exercise intolerance 3. Mitochondrial Encephalomyopathy, Lactic Acidosis, and Stroke-like episodes (MELAS) Cause: Defects in oxidative phosphorylation leading to lactic acidosis Symptoms: Muscle pain, seizures, stroke-like episodes, hearing loss 4. NARP Syndrome (Neuropathy, Ataxia, Retinitis Pigmentosa) Cause: Mutations affecting ATP synthase and ETC components Symptoms: Neuropathy, balance issues, vision problems