Lecture_4_updated.pdf
Document Details
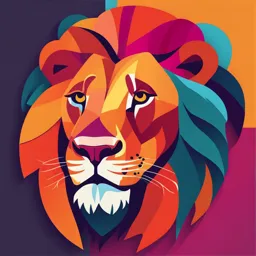
Uploaded by UnboundMaracas
香港都会大学
Full Transcript
Lecture 4 Metabolism and Energy I: Tricarboxylic Acid (TCA) Cycle Dr. Sophie SHI Ling [email protected] Department of Applied Science School of Science and Technology 1 Contents I. Introduction to Metabolism II. Pyruvate...
Lecture 4 Metabolism and Energy I: Tricarboxylic Acid (TCA) Cycle Dr. Sophie SHI Ling [email protected] Department of Applied Science School of Science and Technology 1 Contents I. Introduction to Metabolism II. Pyruvate Oxidation III. TCA Cycle 2 Energy and human life “ Metabolism refers to a series chemical reactions that occur in a living organism to sustain life.” 3 Metabolism Definition Living cells depend on a complex, intricately regulated system of energy-producing and energy-utilizing chemical reactions called metabolism Classification ✓ Catabolism Responsible for degradation of ingested foodstuffs or stored fuels such as carbohydrate, lipid, and protein into either usable or storable forms of energy Result in conversion of large complex molecules to small molecules, ultimately CO2 and H2O, and often require consumption of O2 Release energy (exergonic) and form ATP ✓ Anabolism Responsible for biosynthesis of large molecules from smaller precursors and then used up in cellular respiration Require energy (endergonic) and powered by the breakdown of ATP Polymerization, an anabolic pathway used to build macromolecules such as nucleic acids, proteins, and polysaccharides, uses condensation reactions to join monomers ✓ ATP links catabolism and anabolism 4 Review——Important Nucleotides ❑ Adenosine triphosphate (ATP) Consists of three main structures: the nitrogenous base, adenine; the sugar, ribose; and a chain of three phosphate groups (triphosphate) bound to ribose The main source of energy for most cellular processes, such as muscle contraction, nerve impulse propagation, and chemical synthesis The stored energy in ATP is primarily contained within the high-energy phosphate bonds that connect its three phosphate groups ATP is hydrolyzed into ADP in the following reaction: ATP+H2O→ADP+Pi+free energy ADP is combined with a phosphate to form ATP in the following reaction: ADP+Pi+free energy→ATP+H2O 5 Anabolism vs. Catabolism 6 Anabolism vs. Catabolism 7 Mitochondria——Central Hub for Cellular Metabolism Mitochondria are vital organelles that act as the central hub for energy production and metabolic processes in eukaryotic cells ❑ Structure of Mitochondria 1. Outer Membrane Smooth and permeable Contains proteins called porins that allow the passage of small molecules and ions 2. Inner Membrane Highly folded into structures called cristae Contains proteins involved in the electron transport chain and ATP synthesis Impermeable to most ions and small molecules, creating a distinct environment 3. Intermembrane Space The space between the outer and inner membranes Plays a role in the proton gradient essential for ATP production 4. Mitochondrial Matrix Enclosed by the inner membrane Contains enzymes for the TCA cycle, mitochondrial DNA (mtDNA), ribosomes, and various metabolic enzymes Site of key metabolic processes, including the TCA cycle and fatty acid oxidation 8 https://www.youtube.com/watch?v=c4JsEBI9u6I Cellular Respiration——The Key Metabolism in Human Definition Cellular respiration is the biochemical process by which cells convert nutrients into energy (ATP) using oxygen, while producing carbon dioxide (CO2) and water (H2O) as byproducts Mitochondria are the primary organelles where cellular respiration occurs, specifically in eukaryotic cells https://www.youtube.com/watch?v=Py4R_Up2uBc 9 Cellular Respiration——The Key Metabolism in Human Importance of Cellular Respiration ❑ Primary Energy Source Provides ATP, the energy currency of the cell, essential for all cellular activities Supports processes such as muscle contraction, nerve impulse transmission, and biosynthesis ❑ Metabolic Pathway Integration Central to various metabolic pathways, linking catabolism and anabolism ❑ Oxygen Utilization Utilizes oxygen efficiently, allowing for high-energy yield compared to anaerobic (absence of oxygen) processes Essential for aerobic (require oxygen) organisms, including humans, to sustain energy needs ❑ Regulation of Metabolism Regulates energy balance and metabolic homeostasis in response to dietary intake and energy demands Helps maintain blood glucose levels and metabolic flexibility 10 Cellular Respiration——The Key Metabolism in Human Key Stages of Cellular Respiration 1. Glycolysis Location: Cytoplasm Process: Glucose is broken down into two molecules of pyruvate 2. Pyruvate Oxidation Location: Mitochondrial matrix Process: Each pyruvate is converted to Acetyl-CoA, producing NADH and releasing CO2 3. TCA Cycle (Krebs Cycle or Citric Acid Cycle) TCA cycle Location: Mitochondrial matrix Process: Acetyl-CoA is oxidized, generating NADH, FADH2, and GTP (or ATP) 4. Oxidative Phosphorylation Location: Inner mitochondrial membrane Process: Electrons from NADH and FADH2 are transferred through the electron transport chain (ETC), creating a proton gradient that drives ATP synthesis 11 Biological Oxidations Biological oxidation is a fundamental process in cellular respiration, facilitating energy production and metabolic efficiency Biological oxidations are the combination of oxidation-reduction reactions of substances in living organisms In this process, cells convert nutrients into energy through the transfer of electrons, resulting in the oxidation of substrates During biological oxidations, the reacting chemical systems move from a higher energy level to a lower one and therefore there is release of energy The energy released as heat is converted to chemical energy by formation of energy rich compound ATP Biological oxidations are brought about by enzymes and co-enzymes ❑ Importance of Biological Oxidations ✓ Energy Production: Biological oxidation is essential for generating ATP, the energy currency of the cell ✓ Metabolic Regulation: Balances energy production and consumption, influencing various metabolic pathways 12 Oxidation-reduction reactions (Redox) Definition An oxidation-reduction (redox) reaction is a type of chemical reaction that involves a transfer of electrons between two species Comprised of two parts, a reduced half and an oxidized half, that always occur together The integration of metabolism and redox reactions is important for maintaining energy balance and supporting cellular activities, with redox processes playing essential roles in energy production, metabolic regulation, and biosynthesis ❑ Oxidation The loss of electrons during a reaction by a molecule, atom or ion Oxidizing agent: a substance in a redox chemical reaction that gains or accepts an electron ❑ Reduction The gain of electrons during a reaction by a molecule, atom or ion Reducing agent: a substance in a redox chemical reaction that donates an electron ✓ During a redox reaction, an oxidizing agent gains electrons, becoming reduced ✓ An reducing agent donates electron, becoming oxidized 13 Free energy Gibbs free energy (G) The portion of the total energy that is available for useful work ∆G: The change in free energy Complete glucose oxidation During complete stepwise oxidation of glucose, a large quantity of energy is made available, illustrated as: Maximum ATP yield: ∆G0’ = -2,864 kJ/mol (-684 kcal/mol) Lipid (i.e., palmitate or a triacylglycerol), has a caloric value nearly three times greater than carbohydrates and proteins Table 1. Free-energy changes and caloric values for total metabolism of various metabolic fuels Molecular ∆G0’ Caloric Values weight Compound kJ/mol kcal/mol kJ/g kcal/g Glucose 180 -2,864 -684 15.9 3.81 Lactate 90 -1,361 -325 15.1 3.62 Palmitate 256 -9,987 -2393 38.8 9.30 Tripalmitin 809 -31,772 -7612 38.8 9.30 Glycine 75 -976 -233 13.0 3.12 14 High-energy compounds and bonds High-energy compounds Implies that the products of their hydrolysis are in more stable forms than the original compound Also known as energy-rich compounds, present in the biological system that when hydrolyzed, produce free energy that is greater or equal to that of ATP (△G= -7.3 kcal/mol) Most of the high energy compounds contain phosphate group High-energy bonds found in the majority of high-energy compounds that produce energy upon hydrolysis Table 2. High-energy compounds and free energy released High-energy compounds ∆G0’ (kcal/mol) Phosphoenolpyruvate -14.8 Carbamoyl phosphate -12.3 Cyclic AMP -12.0 1,3-Bisphosphoglycerate -11.8 Phosphocreatine -10.3 Acetyl phosphate -10.3 Pyrophosphate -8.0 Acetyl coenzyme A -7.7 ATP -7.3 15 Enzyme and coenzyme in biological oxidation Enzyme Definition: proteins that act as biological catalysts by accelerating chemical reactions Enzymes involved in biological oxidation are called oxidoreductases, classified into: ✓ Oxidases ✓ Dehydrogenases ✓ Hydroperoxidases ✓ Oxygenases Coenzyme Definition: organic compounds required by enzymes for catalytic activity Coenzymes involved in biological oxidation include: ✓ Nicotinamide adenine dinucleotide (NAD+) ✓ Nicotinamide adenine dinucleotide phosphate (NADP+) ✓ Flavin mononucleotide (FMN) ✓ Flavin adenine dinucleotide (FAD) 16 NAD+ and NADPH in catabolism and anabolism Catabolic reactions are generally oxidative in nature, because the carbons in the substrates (carbohydrates, fats and proteins), are in reduced state Electrons and protons are transferred to nicotinamide adenine dinucleotide (NAD+) by enzymes with formation of NADH The reductive and oxidative reactions of the NAD+ — NADH cycle are central in conversion of the chemical energy of carbon compounds in foodstuffs into ATP Anabolism, by contrast, is largely a reductive process since small, more highly oxidized molecules are converted into large complex molecules The reducing power used in anabolism is provided by NADPH, A phosphorylated NADH 17 Contents I. Introduction to Metabolism II. Pyruvate Oxidation III. TCA Cycle 18 Pyruvate Oxidation in Cellular Respiration Pyruvate oxidation is a key metabolic step linking glycolysis and the TCA cycle Converting pyruvate into Acetyl-CoA Pyruvate Oxidation Occurs in the mitochondrial matrix TCA cycle Enabling the complete oxidation of glucose Critical for ATP production and overall cellular metabolism 19 Acetyl coenzyme A (Acetyl-CoA) Definition A metabolic intermediate that is involved in many metabolic pathways in an organism Composed of an acetyl group (CH₃CO−) linked to Coenzyme A Acetyl-CoA is produced during the breakdown of glucose, fatty acids, and amino acids Function Energy Production: A key molecule in the tricarboxylic acid (TCA) cycle, which is a series of chemical reactions that occur in the mitochondria of cells and is responsible for generating energy in the form of ATP Biosynthesis: Precursor for fatty acids, cholesterol and ketone bodies Amino Acid Metabolism: Participates in the metabolism of certain amino acids Structure Acetyl group 20 Acetyl coenzyme A (Acetyl CoA) Sources of Acetyl CoA 21 Acetyl coenzyme A (Acetyl CoA) Fates of acetyl CoA Complete oxidation of the acetyl group in the TCA cycle for energy generation Conversion of excess acetyl CoA into the ketone bodies, acetoacetate and β-hydroxybutyrate in liver Transfer of acetyl as citrate to the cytosol with subsequent synthesis of long-chain fatty acids and sterols 22 Pyruvate Definition Pyruvate is the conjugate base of pyruvic acid, a key intermediate in many biological processes Chemical Formula: C₃H₄O₃ Pyruvate is produced during glycolysis, it’s the end product of glucose breakdown Pyruvate is also formed by the degradation of amino acids Function Energy Production ✓ Can be converted to acetyl-CoA for entry into the TCA cycle ✓ Reduced to lactate in anaerobic conditions (e.g., during intense exercise) Metabolic Hub: Links carbohydrate metabolism to fat and protein metabolism Structure 23 Pyruvate Metabolic Sources and Fates of Pyruvate 24 Pyruvate oxidation Definition Pyruvate oxidation is the conversion of pyruvate into Acetyl-CoA, linking glycolysis to the TCA cycle Location Occurs in the mitochondrial matrix of eukaryotic cells Process: Three steps CoA-SH: Coenzyme A or CoA 25 Pyruvate oxidation The oxidative decarboxylation of pyruvate is catalyzed by pyruvate dehydrogenase Pyruvate dehydrogenase is a multienzyme complex: i. Pyruvate dehydrogenase (E1) ii. Dihydrolipoyl transacetylase (E2) iii. Dihydrolipoyl dehydrogenase (E3) Bind to three cofactors: thiamin pyrophosphate (TPP), lipoamide, and flavin adenine dinucleotide (FAD) ❖ Pyruvate dehydrogenase is strictly regulated 1. Regulated by reactant & product 2. Regulated by enzyme Pyruvate dehydrogenase can be phosphorylated (inactive) or Activators: NAD⁺, CoA dephosphorylated (active) Inhibitors: Acetyl-CoA and NADH Regulated by pyruvate dehydrogenase kinase (inhibits) and pyruvate dehydrogenase phosphatase (activates) 26 Contents I. Introduction to Metabolism II. Pyruvate Oxidation III. TCA Cycle 27 The tricarboxylic acid (TCA) cycle Basics Acetyl-CoA produced in the energy-generating catabolic pathways of most cells is completely oxidized to CO2 in a cycle of reactions termed the tricarboxylic acid (TCA) cycle Also called the citric acid cycle or the Krebs cycle after Sir Hans Krebs who postulated its essential features in 1937 Primary location of TCA cycle is in mitochondria matrix TCA cycle converts nicotinamide adenine dinucleotide (NAD+) and flavin adenine dinucleotide (FAD) into NADH and FADH2 The resulting NADH or FADH2 is then oxidized by the mitochondrial electron transport chain (respiratory chain) to generate energy that is used to form ATP by oxidative phosphorylation 28 Overview of the tricarboxylic acid (TCA) cycle 29 Reactions of TCA cycle Step 1 2C + 4C → 6C Step 5 4C → 4C Acetyl CoA (2-carbon) joins with oxaloacetate Succinyl CoA is converted to succinate (4-carbon) (4-carbon) to form citrate (6-carbon) One GTP molecule is produced Step 2 6C → 6C Step 6 4C → 4C Citrate is converted to isocitrate (an isomer of citrate) Succinate is converted into fumarate (4-carbon) One molecule of FADH₂ is produced Step 3 6C → 5C + 1C Isocitrate (6-carbon) is oxidized to alpha- Step 7 4C → 4C ketoglutarate (5-carbon) Fumarate is converted to malate (4-carbon) One NADH molecule is formed, and CO2 is released Step 8 4C → 4C Malate is converted into oxaloacetate Step 4 5C → 4C + 1C The third molecule of NADH is produced Alpha-ketoglutarate is oxidatively decarboxylated to form a succinyl-CoA (4- carbon) A second molecule of NADH molecule is produced, and CO2 is released 30 Step 1: Condensation of oxaloacetate with acetyl-CoA Condensation of acetate with oxaloacetate to form citrate (citric acid/ tricarboxylic acid) Acetate enters the cycle as acetyl-CoA and free coenzyme A is liberated in the reaction The enzyme catalyzing the reaction is citrate synthase The only step of the TCA cycle which a C-C bond is formed This reaction is irreversible, a key regulatory point in the TCA cycle 2-carbon 6-carbon 4-carbon ∆G0’ = -32.2 kJ/mol 31 Step 2: Isomerization An isomerization converts citrate to an isomer, isocitrate Citrate, a tertiary alcohol, is a poor substrate for oxidation Isocitrate, secondary alcohol, is a good substrate for oxidation The enzyme catalyzing the isomerization is called aconitase This reaction is reversible 6-carbon 6-carbon ∆G0’ = 13.3 kJ/mol 32 Step 3: First oxidative decarboxylation Oxidative decarboxylation ✓ Lose a carbon atom as CO2 ✓ Alcohol oxidized to ketone ✓ NAD+ reduced to NADH ✓ Irreversible reaction Catalyzed by isocitrate dehydrogenase Produces α-ketoglutarate, a key intermediate that continues through the TCA cycle This step is the rate-limiting step of TCA cycle, also considered as a critical regulatory point 6-carbon 5-carbon 1-carbon 33 Step 4: Last oxidative decarboxylation Oxidative decarboxylation: ✓ Lose a carbon atom as CO2 ✓ Converts α-ketoglutarate to the high-energy thioester succinyl-CoA ✓ NAD+ reduced to NADH ✓ Irreversible reaction Catalyzed by α-ketoglutarate dehydrogenase This step is an important control point in the TCA cycle 5-carbon 4-carbon 1-carbon 34 Step 5: Production of GTP(ATP) The high-energy thioester bond of succinyl CoA is hydrolyzed Converts succinyl CoA to succinate Released energy is used to synthesize GTP (a high-energy compound like ATP) GTP is produced directly in this step, which can be readily converted to ATP Catalyzed by succinyl CoA synthetase Reversible reaction 4-carbon 4-carbon ∆G0’ = -2.9 kJ/mol 35 Step 6: Oxidation of succinate Succinate is oxidized to fumarate, involving the removal of two hydrogen atoms FAD (flavin adenine dinucleotide) is reduced to FADH₂ Catalyzed by succinate dehydrogenase Reversible reaction, nearly equilibrium 4-carbon 4-carbon 36 Step 7: Hydration of fumarate to malate Fumarate is hydrated to the alcohol, malate Catalyzed by fumarase Reversible reaction 4-carbon 4-carbon 37 Step 8: Oxidation of malate to oxaloacetate Malate is oxidized to oxaloacetate NAD+ is reduced to NADH Back to the beginning of the TCA cycle Catalyzed by malate dehydrogenase Reversible reaction 4-carbon 4-carbon 38 Summary of TCA cycle Reversible or Step Reaction Reactant Product Enzyme Not 1 Condensation Acetyl-CoA Citrate Citrate synthase No Oxaloacetate 2 Isomerization Citrate Isocitrate Aconitase Yes 3 Oxidative Isocitrate α-ketoglutarate Isocitrate No decarboxylation NAD+ CO2 dehydrogenase NADH 4 Oxidative α-ketoglutarate Succinyl-CoA α-ketoglutarate No decarboxylation CoA CO2 dehydrogenase NAD+ NADH 5 Phosphorylation Succinate-CoA Succinate Succinyl-CoA Yes / hydration GDP CoA synthetase GTP (ATP) 6 Dehydration Succinate Fumarate Succinate Yes FAD FADH2 dehydrogenase 7 Hydration Fumarate Malate Fumarase Yes H2O 8 Dehydration Malate Oxaloacetate Malate Yes NAD+ NADH dehydrogenase 39 Summary of TCA cycle For each acetyl-CoA molecule, the products of the TCA cycle are: Two CO2, three NADH molecules, one FADH2 molecule, and one GTP/ATP molecule 40 Roles of TCA cycle 1. Energy Production ATP Generation: Produces NADH and FADH₂ for ATP synthesis in the electron transport chain Substrate-Level Phosphorylation: Directly generates GTP (or ATP) 2. Metabolic Intermediates (Metabolic Hub) Sources of Biosynthesis: Supplies intermediates for amino acids and nucleotides Anaplerotic Reactions: Replenishes cycle intermediates for other pathways 3. Linking Pathways Integration with Glycolysis: Connects carbohydrate metabolism (via pyruvate) to energy production Fatty Acid Oxidation: Acetyl-CoA from fat metabolism enters the cycle, integrating lipid and carbohydrate metabolism 4. Regulation of Metabolism Regulated by substrate availability; sensitive to energy needs (ATP, ADP, NADH) Feedback Mechanisms: Ensures homeostasis based on cellular energy levels 5. Removal of Metabolic Wastes CO₂ Production: Facilitates removal of carbon waste, maintaining acid-base balance 41 Roles of TCA cycle ❑ TCA cycle is amphibolic pathway Amphibolic: Serve in both catabolic and anabolic processes Catabolism of carbohydrates to provide energy Anabolism to provide precursors for biosynthetic pathway ✓ α-ketoglutarate can serve as precursor of glutamate ✓ Oxaloacetate can serve as precursor of aspartate 42 Anaplerotic reactions Oxaloacetate, is regenerated during TCA cycle However, metabolic pathways remove intermediates of TCA cycle biosynthetic pathways In order to maintain a functional cycle, a source of four-carbon acids is required to replenish the loss of oxaloacetate Anaplerotic reactions are the act of replenishing four- or five-carbon intermediates in TCA cycle Anaplerotic means “fill up” The most important enzyme is pyruvate carboxylase, which converts pyruvate and CO2 to oxaloacetate 43 Regulation of TCA cycle activity ❑ TCA cycle can be regulated by: Enzymes 1. Pyruvate dehydrogenase —— Production of acetyl-CoA 2. Citrate synthase —— Step 1 3. Isocitrate dehydrogenase —— Step 3 4. α-ketoglutarate dehydrogenase —— Step 4 Activated by substrate availability The presence of substrates (e.g., Acetyl-CoA, oxaloacetate) influences the cycle's activity Inhibited by product accumulation ❖ Overall products of the pathway are NADH and ATP ▪ NADH (NAD+) and ATP (AMP) affect all regulated enzymes in the cycle ▪ Inhibitors: NADH and ATP ▪ Activators: NAD+ and AMP 44 Diseases associated with TCA cycle ❑ Mitochondrial Disorders Genetic defects affecting mitochondrial function can impair the TCA cycle Leigh Syndrome: Neurological disorder due to energy production failure ❑ Cancer Altered metabolism: Many cancers exhibit altered TCA cycle activity (e.g., mutations in succinate dehydrogenase) Cancer cells often rely on glycolysis over oxidative phosphorylation, affecting TCA cycle function ❑ Metabolic Disorders Hyperlipidemia: Impaired TCA cycle can lead to lipid accumulation Diabetes: Altered energy metabolism and TCA cycle activity contribute to insulin resistance ❑ Neurodegenerative Diseases Alzheimer's Disease: Reduced TCA cycle activity linked to energy deficits in neurons Parkinson‘s Disease: Impairment in mitochondrial function affects TCA cycle efficiency, contributing to neurodegeneration 45 Diseases associated with TCA cycle Mutations in TCA cycle enzymes may result in various kinds of cancers These diseases may possibly be treated through pharmacological and genetic therapeutic approaches IDH, isocitrate dehydrogenase; SDH, succinate dehydrogenase; FH, fumarate hydratase; MDH, malate dehydrogenase 46