Lecture 4 2025 - Cytoskeletal Filaments PDF
Document Details
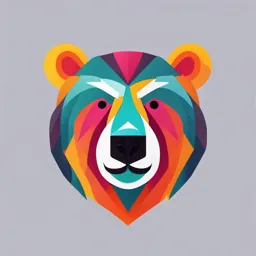
Uploaded by YouthfulGothicArt
2025
Tags
Summary
This document is a lecture covering how cells regulate their cytoskeletal filaments, including microtubules and actin filaments. Key topics explored include how accessory proteins modify cytoskeletal dynamics, nucleation, and different types of filaments.
Full Transcript
https://www.youtube.com/watch?v=YGU0uN_RAIo 1 Lecture 4 How cells regulate their cytoskeletal filaments 2 How accessory proteins modify the dynamics and structure of the cytoskeleton γ-tubulin n...
https://www.youtube.com/watch?v=YGU0uN_RAIo 1 Lecture 4 How cells regulate their cytoskeletal filaments 2 How accessory proteins modify the dynamics and structure of the cytoskeleton γ-tubulin nucleates microtubules from the microtubule- organizing center (MTOC). Microtubules are nucleated from their minus ends with the plus end growing outward from the MTOC Figure 16-29 Molecular Biology of the Cell (© Garland Science 2008) This has a lot to do with where the specific cytoskeletal elements nucleate. MTs nucleate at the MTOC, it is usually oriented in one place in the cell – there are exceptions which we will mention, but Gamma tubulin nucleates MTs from the MTOC and the MTs are always nucleated from their minus ends, their plus ends grow out. Gamma tubulin is anchored to accessory proteins, this complex makes up the first part of the 13 part ring structure- it allows for the first tubulin dimers to be added and thus bypassing that rate limiting nucleation step. If we mutate or remove the gamma tubulin from a cell the MTOC formation is extremely slow and less organized. A and C just show electron micrographs that show the morphology of the MTOC, mainly you can see the globular morphology of the accessory proteins that the MT starts polymerizing from. IMPORTANT to note, that the MT is growing in one direction- in the plus end direction. This is because the minus end is (usually) stuck/stabilizing by this MTOC, so in cells in MOST cases the minus end is stabilized and the plus end is the one that is growing or shrinking – this is still an area that requires more research as it isn’t overly well understood. We have been teaching you that both ends of the MTs are dynamic, this IS true if the minus end is not bound by the MTOC (like in in vitro experiments). Alpha is always toward the gamma tubulin and beta toward the plus or growing end of the MT 3 Microtubules emanate from the centrosome in animal cells Most animal cells have a single well-defined MTOC called the centrosome located near the nucleus. The cytoplasmic microtubules emanate in a star- like conformation. A centrosome is composed of a fibrous matrix that contains 50 copies of γ-tubulin. Most of the proteins that form this matrix are unknown. 4 You can see in the middle we have the centrosome (MTOC), this is where the minus end is anchored and stabilized. The plus end grows outwards from the centrosome and they will grow or shrink depending on a few factors, in part simply due to the concentration of available subunits that are present. This representation is called a MT aster, because you have spikes emanating out from one bright spot and it looks like a star. Most MTs do grow from the MTOC, but in some cases they can get severed or moved and this can be in response to a few things including trauma, but also differentiation, when we highly polarized cells and more stable MTs they do not always need to start at an MTOC but they can be associated with and stabilized by other accessory proteins. 5 Embedded in the centrosome is a pair of somewhat mysterious structures called centrioles arranged at right angles to each other. Centrioles duplicate during cell division and move to opposite poles of the nucleus when mitosis begins. The molecular basis for their duplication is not well understood. Centrioles are rod like structures that appear at right angles to each other 6 Figure 16-30a Molecular Biology of the Cell (© Garland Science 2008) Here you can see the pair of centrioles, they are surrounded by a centrosome matrix, we know the make of centrioles but when we are discussing this full complex there are things are remain unknown. What is known is that part of this complex is made up of these gamma tubulin ring complexes. The centrosome has many many tubulin rings that are all capable are polymerizing MTs. In the video you saw how dynamic MTs are, and that largely based on the plus end of the MT, the minus end is relatively stable. 7 In animal cells, the astral configuration, with plus ends pointing out, acts as a device to survey the outlying regions of the cell to position the centrosome at its center and to provide an internal coordinate system to properly position organelles. This is true in dividing cells, not necessarily in differentiated cells Figure 16-32b Molecular Biology of the Cell (© Garland Science 2008) As the MTs are pushing towards the edge of the cell they are reaching resistance against the actin cortex and the PM, if you have a full star like structure pushing outwards, it will orient the centrosome to the center of the cell which is usually right near the nucleus and tends to be there the cis golgi network is found (transport vesicles and the trans golgi etc- anything that is going to be transported within the cell). This allows the cell to interpret a direction that the things need to move 8 For epithelial cells that form cell-cell junctions and become highly polarized, microtubules may arrange differently. MTs in this case are not localized in the MTOC Figure 16-5 Molecular Biology of the Cell (© Garland Science 2008) This is an example of a differentiated cell, where we do not have an MTOC, MTs can arrange differently, we still have a minus end oriented towards the apical side (towards the lumen- inside of the blood vessel) and plus end oriented towards the basal side of the cell where you would have the ECM. This is where the orientation of the MTs is important- it gives directionality for transport. In this case the minus end is not oriented towards the MTOC. But this would happen after cell division, once the cell is terminally differentiated and taking on this polarized structure. 9 Actin filaments are often nucleated at the plasma membrane In contrast to microtubule nucleation, actin nucleation occurs most frequently at or near the plasma membrane. Actin structures can form different types of cell surface projections including spiky bundles microvilli or filopodia, protrusive veils called lamellipodia, and the phagocytic cups in macrophages. In the last lecture we looked at diagrams of these structure but now we are going to talk about how those structures are formed 10 Figure 16-7 Molecular Biology of the Cell (© Garland Science 2008) We have many small subunits of proteins that are polymerizing into a large filament- this filament is very dynamic, it can depolymerize and repolymerize in response to a signal and the filaments can be arranged in different orientations depending on which structure they are going to be making up, branched (lamellipodia, filamentous – filopodia) 11 External stimuli frequently regulate the nucleation of actin filaments allowing the cell to change its shape and stiffness in response to a stimulus. This nucleation can be catalyzed by two different types of regulated factors: the ARP complex and the formins. Figure 16-34b Molecular Biology of the Cell (© Garland Science 2008) When you have an activating factor (a signal) the ARP complex will go from the inactivate to active state, but what I want you to see is the orientation of the ARP2 and 3 proteins are what gives the proper shape to nucleate the actin monomers, they fit into the ARP complex and will start to grow. The filament that is growing is linear. But ARP actually is responsible for making branched actin 12 *Filament nucleation by ARP occurs at 70 degree angles Ie polymerizes branched actin Figure 16-34c Molecular Biology of the Cell (© Garland Science 2008) The ARP complex binds to existing polymers of actin and it binds at a 70 degree angle, you will have 1 polymer of straight actin and the ARP complex will bind somewhere in the center. At the growing tip, what type of actin is present? ATP, so if the ARP complex always binds in the middle you can hypothesize that it has a higher affinity for the ADP bound actin. And as a result of binding at a 70 degree angle we start to build these big branched networks 13 The mechanism of nucleation influences large-scale filament organization The ARP complex nucleates the growth of a new actin filament most efficiently when it is bound to the side of an old actin filament (ie higher affinity for ADP than ATP actin). However, many large-scale actin structures are made up of parallel bundles of unbranched actin filaments nucleated by the formins. 14 Formins are a family of dimeric proteins that have a binding site for monomeric actin. As the nucleated filament grows, formin remains associated with the rapidly growing plus end. Very different than ARP which remains stably bound to the minus end. Figure 16-36 Molecular Biology of the Cell (© Garland Science 2008) Visualize this as a spinning top that spins and rotates on one pin, formin rotates at the surface of the growing (plus) end of the polymer, it is spinning and adding one actin monomer at a time to the growing end. This is very different than the ARP where when it start polymerizing the actin filament it will remain a part of the filament until the whole thing depolymerizes. Formin is constantly moving 15 Figure 16-47 Molecular Biology of the Cell (© Garland Science 2008) When we go back to the cell and think about how we have 2 different types of actin that we can make (branched or filamentous) where are they and what do they do. We have the big lampelopodia that is made up of branched actin and filopodia made up of filamentous actin. Then at the back we have stress fibers and they are going to become very important as we talk about motor proteins and get into cell migration, the stress fibers provide anchoring point so the cell can pull itself along, and they are made up of parallel bundles, but they are different than what is found in the filopodia- where you can see have a distinct directionality, they are all pushing towards the PM. In stress fibers, because they are making multiple contacts and have to pull many things together, they tend to be in opposite orientation to facilitate this. (this will become more clear next lecture) 16 Proteins that bind to the free subunits modify filament elongation In most vertebrate cells, approximately 50% of actin is in filaments and 50% is soluble. The soluble monomer concentration is 50-200 μM, Surprising given that the critical concentration of actin in a test tube is less than 1 μM. Specialized proteins such as thymosin prevent actin nucleation by binding to monomeric actin. So based on the high concentration of soluble actin molecules – they should always be in a state of growth- but they aren't. And the reason for this is that there are specialized proteins that prevent actin polymerization by sequestering them, such as thymosin. Thermodynamically we are in a constant state that would favor actin polymerization, this is not what we want so we have to stop that and we do that with these specialized proteins that limit the amount of available actin monomers that can actually be added to the polymer. The cell is expending energy to limit the amount of actin monomers available at any time for polymerization, this is all just to allow for an extremely quick response to a stimulus, so the cytoskeleton can change rapidly. 17 How is "locked“ actin recruited for polymerization? The recruitment depends on another monomeric binding protein called profilin. Profilin binds to actin at the face that normally binds the minus end, promoting the attachment of the monomer to a free growing plus end. Profilin competes with thymosin, therefore, a shift toward the activation of profilin molecules promotes polymerization. 18 * Formin whiskers contain several binding sites for profilin or the profilin-actin complex. These flexible domains serve as a staging area for addition of actin to the growing plus end of the actin filament when formin is bound Figure 16-38 Molecular Biology of the Cell (© Garland Science 2008) Start at the top – we have in dark blue our actin monomer and that is being sequestered by thymosin, then we have profilin in yellow and formin in red, formin whiskers (green) contain several binding sites for profilin or the profilin-actin complex. These flexible domains serve as a staging area for addition of actin to the growing plus end of the actin filament when formin is bound. What is happening, remember formin is the table top spinner, formin is spinning along the growing end, it has binding sites for profilin and the profilin competes with thymosin to have actin available to add- so you get actin added. The formin will then add these available actin subunits to the growing chain (grabbing it and adding it like a hat as it spins). 19 Several intracellular mechanisms regulate profilin activity including profilin phosphorylation and profilin binding to inositol phospholipids. Profilin is localized at the cytosolic face of the plasma membrane where it binds to phospholipids awaiting activation by extracellular signals. This produces a rapid polymerization of actin at the surface of the membrane. Inositol PL- are at the PM. So profilin is associated with the cytosolic side of the PM where it would be able to bind to these PL and await activation in response to some extracellular signal. So you have actin locked by thymosin and you have the key that unlocks actin – profilin that is bound to the PM. You get some signal from the extracellular signal – RTK activation, this results in profilin being released where it can go an “unlock” actin and make it available for polymerization 20 What about Tubulin? As with actin monomers, the cell sequesters unpolymerized tubulin subunits through the stathmins. Stathmins bind to two tubulin heterodimers preventing their addition onto growing filaments. Stathmin phosphorylation release tubulin and enhances polymerization. Cancer cells frequently overexpress stathmins resulting in an increased rate of microtubules turnover that contributes to the morphological differences between cancer cells and normal cells. 21 Severing proteins regulate the length and kinetic behaviour of actin filaments Severing existing long filaments into smaller filaments accelerates the assembly of new filaments by exposing more plus ends. Severing also obviously can accelerate depolymerization by 10-fold by breaking up old filaments. The proteins we have talked about so far have dealt with polymerization of the filament/tubule. But there is also another types of protein that results in the cleavage or severing of the filaments. If you sever a filament in the center you do not have the ATP (or GTP) cap anymore and you will have catastrophe right away. 22 13 longitudinal bonds must be broken to sever a microtubule. This task is performed by the protein katanin. Katanin hydrolyzes ATP to perform this task and is directed to the centrosome where it releases microtubules directly from the centrosome Gelsolin performs this task for actin severing without consuming any energy although the mechanisms for this process are unclear. 23 Proteins that bind along the sides of filaments can either stabilize or destabilize them Different filament-associated proteins use their binding energy to either lower or raise the free energy of the polymer state. Microtubule-associated proteins (MAPs) such as MAP2 and tau are prominent in neurons and stabilize microtubules. Large aggregates of tau filaments called neurofibrillary tangles are present in Alzherimer's disease. Now we have talked about controlling polymerization via various proteins for both MT and MF, but now we are going to talk about stabilization. Tau stabilizes MT present in axons, in AD we see hyperphosphorylation of Tau proteins, so they cannot stabilize the MTs. So the dementia we see associated with AD is a result of impaired communication between neurons because the MT is not able to maintain a stable connection, cannot maintain the synapses needed for communication. 24 Second MT binding site * Long tail Short tail Highly spaced Tightly packed As a result, they cluster to different regions of a because of long tail because of short tail neuron, MAP2 (orange) dendrites, Tau (green) axon Figure 16-41 Molecular Biology of the Cell (© Garland Science 2008) A neuron is a polar cell, it has only 1 axon but many dendrites you can see here. We have MAP2 labelled in orange and Tau labelled in green. MAP2 is present in dendrites they generally receive input from other neurons, the neuron communicates through a single axon, it can be highly branched but is one extension from the cell body, this is where you find Tau (in the axon). And you can see that Tau takes on a structure so that both the N and C terminals are bound to one MT, it stabilizes it but also creates a SHORT spacer. Whereas MAP2 bind the first MT on one end and a different MT on the other end. That ends up with different spacing that occurs between MTs within each of these structures. The MTs in the dendrites have a larger space between them when compared to the MTs in the axon. 25 Actin filaments are stabilized by the binding of tropomyosin, an elongated protein that binds to 7 adjacent actin subunits. Cofilin destabilizes actin filaments by binding and forcing the filament to twist more tightly. It preferentially binds to old ADP-containing filaments, and tropomyosin protects actin filaments from cofilin. Figure 16-42 Molecular Biology of the Cell (© Garland Science 2008) The length actin changes in the presence of cofilin because it twists the filament tighter putting more stress on the bonds and increasing the chances of them breaking. Tropomyosin protects against this by interacting with the actin filament and not allowing cofilin to bind. 26 Proteins that interact with filament ends can dramatically change filament dynamics Proteins that bind along side a filament need to be present in high stoichiometric ratios such as 1 tropomysion/7 actin subunits or 1 cofilin/1 actin subunit. One tau for every 4 tubulin. However, proteins that bind to filament ends can have dramatic effects at low concentrations (1 molecule/200- 500 actin monomers) Proteins such as CapZ bind and stabilize the plus end of actin, while the ARP complex stabilizes the minus end. CAPZ = capping protein 27 Intermediate filaments are cross-linked and bundled into strong arrays Filaggrin bundles keratin filaments in the outer layer of the skin to give them toughness Plectin cross-links intermediate filaments to microtubules and actin filament bundles. Mutations in the gene for plectin cause a devastating disease in humans that combines epidermis bullosa, muscular dystrophy, and neurodegeneration. Epidermolysis bullosa simplex with muscular dystrophy (EBS-MD) 28 Actin parallel filaments vs. actin webs Villin and fimbrin cross-link actin into parallel bundles. A bundle of parallel actin filaments cross-linked by the actin-bundling proteins villin and fimbrin forms the core of a microvillus. All the plus ends of the actin filaments are at the tip of the microvillus, where they are embedded in an amorphous, densely staining substance of unknown composition Figure 16-50a Molecular Biology of the Cell (© Garland Science 2008) You see here microvilli, we have actin filaments in red, we have myosin and calmodulin and then we have these crosslinking proteins (villin and Fimbrin). 29 Actin parallel filaments vs. actin webs Web-forming/stabilizing proteins are the filamins and spectrins. Some types of cancer cells, especially malignant melanomas lack filamin and cannot crawl properly. Spectrins allow red blood cells to spring back to their original shape after squeezing through a blood vessel and provide flexible stiffness to most other types of vertebrate cells. 30 Each filamin homodimer forms a flexible, high angle link between two adjacent actin filaments Figure 16-51 Molecular Biology of the Cell (© Garland Science 2008) 31 Proteins we discussed… MICROTUBULES MICROFILAMENTS γ-tubulin Tropomyosin Stathmins Cofilin Katanin Villin Gelsolin Fimbrin Filamins MAPs Spectrins – MAP2 CAPZ – Tau ARP Formins Thymosin Profilin Plectin**IF Filaggrin**IF 32 How does the centrosome “know” when it has found the center of the cell? How are the γ-tubulin ring complex and the ARP complex similar, and how are they different? How does cofilin distinguish between old and new actin filaments? The concentration of actin in cells is 50-100 times greater than the critical concentration observed for pure actin in a test tube. How is this possible? Why is it advantageous? * Many answers for the second question, if asked on an exam I would have to accept many answers or be more specific. Mainly here looking for: Similarities: both bind to and stabilize the minus end of a cytoskeletal element and promote nucleation. Difference – ARP is associated with branched actin given it interacts with ‘older’ actin filaments at a 70 degree angle, gamma tubulin builds microtubules which are not branched. 33