Lecture 3 Outline - B10 - Fall 2024 PDF
Document Details
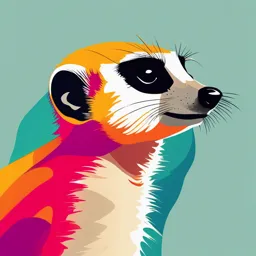
Uploaded by ThrivingDiction
2024
Tags
Summary
This document outlines lecture 3 on biological macromolecules, including lipids, carbohydrates, nucleic acids, and proteins. It also covers energy, and how cells obtain and use energy.
Full Transcript
We will first complete our discussions of the few remaining slides at the end of the Lec 2 slide deck Four types of biological macromolecules 2. Lipids A large group of nonpolar biological molecules -composed mainly of C,H and O -dissolve in organic solvents but not...
We will first complete our discussions of the few remaining slides at the end of the Lec 2 slide deck Four types of biological macromolecules 2. Lipids A large group of nonpolar biological molecules -composed mainly of C,H and O -dissolve in organic solvents but not in water Lipids with important cell functions: -Fats -Steroids -Phospholipids, Glycolipids Lipids: Fats Fats = triacylglycerol = glycerol + 3 fatty acids Fatty acids linked by ester bonds Fatty acids: long hydrocarbon chains with a single carboxyl at one end Lipids: Fats -fatty acids vary in length -no double bonds =saturated Double bonds =unsaturated Lipids: Steroids -Complex ring structures -4 hydrocarbon rings -Ex: Cholesterol - important animal plasma membrane component -building blocks of many steroid hormones Lipids: Phospholipids -composed of glycerol + 2 fatty acids + phosphate + head group -major component of plasma and organelle membranes -hydrophilic on one end and hydrophobic on the other = amphipathic Lipids: Glycolipids Lipids form biological membranes -arrange into a bilayer -hydrophobic regions point inwards (interior); hydrophilic regions on the outside http://www.biologycorner.com/resources/lipidbilayer.gif http://www.bioteach.ubc.ca/Bio-industry/Inex/graphics/phospholipid.gif Four types of biological macromolecules Nucleic Acids -DNA (deoxyribonucleic acid) and RNA (ribonucleic acid) -DNA: genetic material; governs all cellular activities; codes for proteins required for the cell’s functions -RNA: many roles; central to the synthesis of proteins; regulates expression of genes; genetic material of some viruses Types: transfer RNA (tRNA); messenger RNA (mRNA); ribosomal RNA (rRNA) -polymers of nucleotides Nucleic Acids: nucleotides Nucleic Acids: nucleotides Nucleic Acids: nitrogenous bases Nucleic Acids: base pairing in DNA The structure of the bases dictate the specificity of pairing: A pairs with T G pairs with C Hydrogen bonds between the bases hold together the 2 strands of DNA in the double helix. Adapted from Fig. 16.8 Biology (8th Ed.) Campbell, Reece. Nucleic Acids: polynucleotide strands 5’ Nitrogenous base -nucleotides are joined by sugar- phosphate linkages -3’ hydroxyl attached to 5’phosphate of the adjoining nucleotide -phosphodiester bond Sugar-phosphate backbone DNA strand 3’ Adapted from Fig. 16.5 Biology (8th Ed.) Campbell, Reece. 5’ 3’ H-bonds 5’ 3’ Fig. L Adapted from Fig. 16.7 Biology (8th Ed.) Campbell, Reece. Four types of biological macromolecules We have been building macromolecules à creating order in cells How do cells obtain and use energy? The second law of thermodynamics states the degree of disorder, or entropy, increases in any isolated system in the universe. How then do cells appear to defy this law by generating order? Cells take in energy from the environment and use it to generate order within it. The many chemical reactions that create this order in cells, converts some of this energy to heat. The heat released then creates disorder in the cell’s environment, increasing entropy and hence abiding by the second law of thermodynamics. Heat generating reactions within the cell are coupled to those that generate molecular order. Figure 2-14 How do cells obtain and use energy? Each cell is a tiny factory and carries out millions of chemical reactions every second. -catalyze the oxidation of organic molecules in small steps à allows useful energy to be harvested -the vast majority of these chemical reactions only occur in cells under normal temperatures because of proteins known as enzymes. These catalysts increase the rate of reactions by lowering the activation energy required. Figure 2-21 How do cells obtain and use energy? Enzymes can speed up reactions, but they cannot force energetically unfavourable ones to occur. DG is a measure of the change in the amount of energy available to do work. Favourable reactions decrease the DG (negative DG) and increase the disorder of the universe. Figure 2-28 The cell uses reaction coupling to drive energetically unfavourable reactions. We use the standard free energy change, DGo (standard conditions, same concentrations of reactants), of reactions to predict the course of biological reactions. Figure 2-29 How do cells obtain and use energy? Cells catalyze the oxidation of organic molecules in small steps à allows useful energy to be harvested This energy is stored in a small set of the activated “carrier molecules” which diffuse through the cell through sites in which they are generated to sites in which biosynthesis will occur. Cells use these carrier molecules like money to pay for reactions that would not otherwise occur. Figure 2-31 The activated carrier molecules are formed by coupling to favourable reactions. The carrier molecule (bucket) picks up sufficient energy to power an unfavourable reaction elsewhere. Figure 2-32 How do cells obtain and use energy? Glucose and other food we eat are broken down by stepwise oxidation reactions to produce chemical energy in the form of ATP and NADH. These reactions also help produce many of the small molecules that are the substrates for biosynthesis of macromolecules. Macromolecules like glycogen and fats can be stored in cells as a major source of energy. Four types of biological macromolecules Proteins -About 1x 104 proteins made in every mammalian cell! -Carry out almost all cellular functions. Examples: -Enzymes - accelerate chemical reactions in the cell -Signaling - kinases, phosphatases are involved -hormones - long range messenger molecules -growth factors -membrane receptors - communication between cells -cell movement - cytoskeleton Proteins: amino acids -polymers of amino acids - 20 different types Amino Acids: -Amino (NH2) and Carboxyl (COOH) groups -These groups are separated by a single carbon (a-carbon) -R groups (side chains) give amino acids their variability Karp’s Karp’s Cell and Molecular Biology: Concepts and Experiments.” 8th edition. Janet Iwasa & Wallace Marshall. Proteins: R groups of amino acids Proteins: R groups of amino acids 4 categories of R groups: -Polar charged -Polar uncharged Arginine (+) in histones (proteins that coil DNA) -Nonpolar can bind DNA (-) Other (glycine, cysteine and proline) 1. Polar charged: - can form ionic interactions Only partially charged at physiologic pH Karp’s Karp’s Cell and Molecular Biology: Concepts and Experiments.” 8th edition. Janet Iwasa & Wallace Marshall. Proteins: R groups of amino acids 2. Polar uncharged: - can form H bonds Karp’s Karp’s Cell and Molecular Biology: Concepts and Experiments.” 8th edition. Janet Iwasa & Wallace Marshall. Proteins: R groups of amino acids 2. Nonpolar: - mostly hydrocarbons -hydrophobic -usually in the core of protein structure (away from water) Karp’s Karp’s Cell and Molecular Biology: Concepts and Experiments.” 8th edition. Janet Iwasa & Wallace Marshall. Proteins: R groups of amino acids 4. Others: reactive sulfhydryl group Karp’s Karp’s Cell and Molecular Biology: Concepts and Experiments.” 8th edition. Janet Iwasa & Wallace Marshall. Proteins: R groups of amino acids Cysteine residues can from disulfide bonds with each other under oxidizing conditions: Karp’s Karp’s Cell and Molecular Biology: Concepts and Experiments.” 8th edition. Janet Iwasa & Wallace Marshall. Proteins: peptide bonds Peptide bonds: Carboxyl group of one amino acid becomes attached to the amino group of another -forms polypeptide chains = proteins Figure 3-3 Figure 3-1B Protein structure Protein structure Protein structures are defined by 4 different levels of organization. Primary structure: (1°) -specific sequence of amino acids -determined by the sequence of the gene encoding the protein (DNA) -20n variations of proteins (20 amino acids) -n = the number of amino acids in the chain -typical protein is over 100 amino acids long - infinite number of sequences possible! -sequence contains most of the information needed to specify 3-D shape and function of protein Protein structure -Changes in the primary structure can have dire consequences for protein function - Ex: sickle cell anemia Karp’s Karp’s Cell and Molecular Biology: Concepts and Experiments.” 8th edition. Janet Iwasa & Wallace Marshall. -affects red blood cell shape- sickle shaped -clogs arteries https://image.slidesharecdn.com/05thestructureandfunctionoflargebiologicalmolecules-130311053304- phpapp01/95/05-the-structure-and-function-of-large-biological-molecules-69-638.jpg?cb=1362980123 Protein structure 3 further levels of organization: -Secondary structure -Tertiary structure -Quaternary structure Proteins must fold in order to have the next 3 levels of organization Protein folding: chaperones While some proteins do fold by a process of simple self-assembly, most proteins need some help! Help = chaperones = proteins that aid in the folding of newly made proteins by preventing inappropriate interactions Protein folding: chaperones Chaperones: -prevent inappropriate interactions with other cellular components -bind hydrophobic segments of proteins -are single proteins or a large protein complex with intricate structure -in cytosol of mammalian cells Karp’s Karp’s Cell and Molecular Biology: Concepts and Experiments.” 8th edition. Janet Iwasa & Wallace Marshall. Protein structure Secondary structure: (2°) -conformation of portions of the polypeptide chain -arranged to maximize the number of H-bonds made between neighboring amino acids – of peptide backbone only -2 common folding patterns: a-helix and b-pleated sheets Protein structure a-helix: -hydrogen bonding that links the C=O of one to the N-H of another (between every fourth amino acid) -cylindrical twisting spiral -R groups project outwards Figure 3-6, A&B Protein structure b-pleated sheets: -hydrogen bonds extend from one part of the chain to another -polypeptide segments lie side by side Figure 3-6, C&D b-pleated sheets and orientation: Protein structure 2° structure: -a helices -b-pleated sheets -loops and turns b-pleated sheets: arrows point from N (start) to C (end) directions Karp’s Karp’s Cell and Molecular Biology: Concepts and Experiments.” 8th edition. Janet Iwasa & Wallace Marshall. Protein structure Tertiary structure: (3°) -conformation of the entire protein -interactions between R-groups in the protein -hydrophobic interactions -Van der Waals interactions -disulfide bridges -3 types: fibrous, globular and intrinsically disordered proteins Protein structure Non-covalent bonds that hold protein tertiary structure together Protein structure Fibrous proteins: -elongated shape -usually structural materials outside of cells -keratin, collagen (hair, skin, fingernails) -form long strands or flattened sheets that resist shearing forces Karp’s Karp’s Cell and Molecular Biology: Concepts and Experiments.” 8th edition. Janet Iwasa & Wallace Marshall. Protein structure Globular proteins: -compact shape -polypeptide chain folds into complex shapes -usually have functions within the cell -enzymes, hormones -most proteins! Karp’s Karp’s Cell and Molecular Biology: Concepts and Experiments.” 8th edition. Janet Iwasa & Wallace Marshall. Protein structure Intrinsically disordered proteins: -loops or tails (or entire proteins) that remain disordered in structure -contain repeated sequences of amino acids -have important functions e.g. elastin Protein structure -Parts of a protein folded into the tertiary structure can have distinct functions from other regions -modules or domains of proteins -substructure produced by any continuous part of a polypeptide -can function in a semi independent manner -can be swapped between proteins -allow for unique protein activities -Ex: move independently; bind different molecules -domains can be constructed from alpha-helices, beta-sheets or various combinations of fundamental folding elements Figure 3-10 Protein structure Quaternary structure: -linking of multiple proteins to form large complexes with multiple “subunits” -multiprotein complexes -intermolecular interactions of R groups -disulfide bonds -noncovalent interactions -mostly Cro repressor protein Homodimer Hemoglobin Heterotetramer Protein structure is stabilized by covalent cross-linkages Figure 3-25 Proteins can be modified & regulated -cleaved into smaller polypeptides -“pro-form" cut into smaller fragments -sugar chains can be added -“glycoproteins” -lipids added -anchored to cell membranes -metal/ions added - important for function -phosphate groups added -signaling functions; alters structure or interactions -GTP or calcium binding -alters protein activity -degradation -controls protein lifespan Regulating proteins -Regulation by degradation -The life span of proteins differ a great deal - few minutes = mitotic cyclins - your whole life = crystallin in lens of the eye -Controlled by regulated degradation pathways Many degradation pathways exist in the cell: -Lysosomes -Autophagy -Proteasomes = 90% of protein degradation in mammalian cells Regulating proteins: degradation Proteasomal degradation: In a “cellular chamber of doom” proteins suffer “a death by a thousand cuts” http://www.igakuken.or.jp/pro-meta/eng/research/images/fig_02.png Regulating proteins: degradation The proteasome degrades proteins for turnover (regulates life span) but also degrades misfolded proteins How can it tell which ones it needs to degrade? Mark = ubiquitination Ubiquitin (Ub) = 76 residue protein Typically, multiple copies of Ub are attached to a protein tagged for proteasomal degradation = polyubiquitination (4 or more Ub) Requires the activity of E1, E2 and E3 enzymes that work in sequence to bring about polyubiquitination Lysine side chains on proteins are Figure 3-66 polyubiquitinated Figure 3-65 Regulating proteins: GTP binding Non-covalent binding to GTP GEF = Guanine Exchange Factor can act as a “switch” to regulate GAP = GTPase Activating Protein protein activity GTP binding proteins = GTPases GTP bound = active = regulate the activity of target proteins (effectors) in this state GDP bound = inactive https://www.intechopen.com/source/html/44095/media/image1_w.jpg Regulating proteins: GTP binding The rate of GTPase activity GEF = Guanine Exchange Factor differs between proteins GAP = GTPase Activating Protein GEFs and GAPs aid in regulating the “switch” that regulates these proteins GEF = Guanine Exchange Factor GAP = GTPase Activating Protein Regulating proteins: phosphorylation Phosphorylation is the most common covalent modification that is used to regulate protein activity. -the reversible addition of a phosphate group to the hydroxyl groups on the side chains of a serine, threonine or tyrosine residue. Kinases = add phosphates Phosphatases = remove phosphates The activities of kinases and phosphatases is hence the “switch” Figure 3-58B Regulating proteins: phosphorylation Phosphorylation can alter the conformation and charge of a protein: -can allow for protein-protein interactions -can allow for altered intrinsic activity (e.g,. enzymes) -can cause a change in the localization of a protein Can impact large signaling pathways in cells. http://physiologyonline.physiology.org/content/22/3/193 Regulating proteins: phosphorylation Individual protein kinases can act as microprocessors – integrate multiple upstream signals -signal integration is required for kinase activation Figure 3-61 Regulating proteins: phosphorylation Figure 3-62 A spectrum of protein modifications Figure 3-81 Something else to think about Something else to think about “Creutzfeld-Jacob disease” (CJD) - protein aggregates seen in brain - neuronal death = fatal disease http://www.cjdinsight.org/Deana/Fig_2.jpg normal CJD Something else to think about CJD is a prion disease Prion protein: PrP PrPc = normal prion protein PrPsc = misfolded, disease-causing prion protein Change in secondary structure between PrPc PrPsc normal and disease form α-helix β-sheet Something else to think about PrPsc: -is a misfolded from of the normal prion protein -can aggregate (seen in brains of CJD patients) = amyloid fibers or plaques -creates a continuous stack of beta sheets to form a cross-beta filament -protein aggregates eventually kill neurons Something else to think about PrPsc is “infectious” “Protein only” hypothesis of disease -can convert PrPc into transmission misfolded protein (PrPsc) -can be transmitted -e.g. contaminated blood transfusions: hemophiliacs