Lecture 24 Chemistry, Functions and Metabolism of Carbohydrates PDF
Document Details
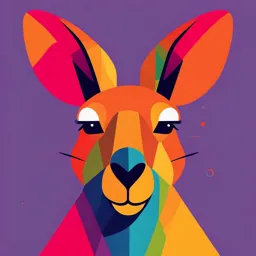
Uploaded by BelievablePraseodymium4425
AIMST University
2024
Tags
Summary
This lecture covers the chemistry, functions, and metabolism of carbohydrates. It details different types of carbohydrates and outlines various metabolic pathways.
Full Transcript
Chemistry, Functions and Metabolism of MBBS YEAR 1 2023 MIBS 65101 Carbohydrates ✔ CLASSIFICATION OF CARBOHYDRATES ✔ STRUCTURE AND FUNCTIONS OF...
Chemistry, Functions and Metabolism of MBBS YEAR 1 2023 MIBS 65101 Carbohydrates ✔ CLASSIFICATION OF CARBOHYDRATES ✔ STRUCTURE AND FUNCTIONS OF CARBOHYDRATES Learning ✔ GLYCOLYSIS MBBS YEAR 1 2024 MIBS 65101 Objectives ✔ TCA CYCLE ✔ METABOLIC PATHWAYS OF OTHER CARBOHYDRATES Carbohydrates “polyhydroxy aldehydes or ketones and their derivatives or as substances that yield one of these compounds on hydrolysis.” Carbohydrates consist of the elements carbon (C), hydrogen (H) and oxygen (O). Hence known as “hydrates of carbon” Carbohydrates are represented by ‘Saccharide’ comes from the word for table sugar in several the general formula languages : sarkara in Sanskrit, Cx(H2O)y. sakcharon in Greek, saccharum in Latin. Simple Complex 04 Classification of carbohydrates Disaccharides of physiological significance Pentoses of physiological significance Hexoses of physiological significance Glycoside Formation When hydroxyl group on the carbon of manosaccharides reacts with the OH group of another carbohydrate or non-carbohydrates leads to glycosides formation. Bonds joining the monosaccharides = glycosidic or glycosyl bonds. There are two types of glycoside bonds : - O-glycosidic bonds - N-glycosidic bonds Glycosidic Bonds If the group on the non-carbohydrate molecule to which the sugar is attached is an –OH group, the structure is an O-glycoside. Eg: Sugar + serine (amino acid) O-glycosidic bond If the group on the non-carbohydrate molecule to which the sugar is attached is an –NH2 group, the structure is an N-glycoside. Eg: Sugar + asparagine (amino acid) N-glycosidic bond Polysaccharides-Glycans MBBS YEAR 1 2024 MIBS 65101 Food storage polysaccharides – Starch, Glycogen Structural polysaccharides - Cellulose, Chitin Starch Storage form of glucose in plants; a polymer of glucose units Complexes with iodine to form a dark blue color Eg. Potato, roots like carrot, legumes and grains Composed of Amylose: Unbranched chain with α1→4 linkage and Amylopectin: Branched (every 24-30 units) with α1→6 linkage Glycogen Storage form of glucose in animals; a polymer of glucose units Eg. Liver and muscle. Branched (every 8-12 units), more highly branched Polysaccharides Cellulose (A linear string of glucose) A polymer of glucose units (hundreds to thousands) The most important structural polysaccharide found in plant cell walls and algae Linear polymer like amylose, but has β-(1-4) glycosidic linkages. In wood ~50% of dry mass (25-33% lignan not mentioned here for glue and water tightness) Cotton is 90% cellulose In humans it is ruffage, not easily digested, a constituent of dietary fiber. Not digested in animals that use cellulose for food, they have bacteria in their guts that have the enzyme to break it down Lower termites use protozoa in their guts and Higher termites Polysaccharides Chitin Units of N-acetylglucosamine β-1,4 linkages (similar to the linkages between glucose units forming cellulose). Chitin may therefore be described as cellulose with one hydroxyl group on each monomer substituted with an acetylamine group. Unmodified: (pliable, translucent, tough) like caterpillar skins Modified (with proteins, hard, with calcium carbonate, very hard) found in crustacean shells, insect exoskelotons Inulin long chain homoglycan of D-fructose with β-1,2 linkages Reserve carbohydrate in tubers, bulbs - chicor, dahlia, onion, garlic etc. Clinical use - Renal clearance test to assess glomerular filtration rate Polysaccharides Dextran Polysaccharide of glucose varying chain length Unbranched Chain α1→6 linkage Branched Chain α1→3 linkage Antithrombotic, reduce blood viscosity, lubricant in eye drops Dextrin Straight chain glucose polymer α1→4 or α1→6 linkage Carbohydrates in glycoprotein Functions of carbohydrates Energy stores - starch, glycogen, trehalose and dextrin Structural elements including mucoprotein matrix of mesenchymal tissues - cellulose, chitin hyaluronic acid, chondroitin sulfate Essential constituents of nucleotides and nucleic acids - ribose and deoxyribose Synthesis of fatty acids and amino acids Important nutrients - lactose Pharmacologic actions - Cardiac glycosides are carbohydrate –steroid complexes –heart - Antibiotics like streptomycin has carbohydrate residue in them. High degree of solvation, high viscosity, good "cushions" & lubricants in connective tissue, joints, etc. - Glycosaminoglycans - chondroitin sulfate, heparin, keratan sulphate Structures of carbohydrate portions provide specific binding determinants for other proteins Cell surface recognition, cell surface antigenicity, extracellular matrix, mucins - glycoproteins, glycolipids Very important in cell-cell adhesion and communication, including developmental events. Cellular Respiration Cellular respiration has three stages: – Glycolysis (breaks down glucose into two molecules of pyruvate) – Citric acid cycle (completes the breakdown of glucose) – Oxidative phosphorylation (accounts for most of the ATP synthesis) The process that generates most of the ATP is called oxidative phosphorylation because it is powered by redox reactions Oxidative phosphorylation accounts for almost 90% of the ATP generated by cellular respiration 1 A smaller amount of ATP is formed in glycolysis and the citric acid cycle by substrate-level phosphorylation Photosynthetic organisms use light E and convert it into ATP. Nonphotosynthetic organisms obtain E from ingested compounds which are metabolised to produce ATP PRIMARY SECONDARY METABOLISM METABOLISM TERTIARY DIGESTION INTERMEDIARY METABOLISM Carbohydrates → Glucose Acetyl CoA ATP Lipids → Fatty acid CO2 Proteins → Amino acids H 2O NADH STAGE 1 STAGE 2 STAGE 3 Digestion and Absorption of Carbohydrates Starch, Lactose, Sucrose, MOUTH Salivary α-amylase (α(1→4) bond Fructose Cl-, pH 6.6-6.8 STOMACH HCl (destroys amylase) Glycogen, Starch, Maltose, Limit INTESTINE Pancreatic amylase (pH 7.1) dextrins, lactose, sucrose, fructose Disaccharidase, Maltose, Limit dextrins, EPITHELIAL CELLS oligosaccharidase, limit lactose, sucrose, fructose Portal vein Transport dextrinase (pH 5-7) Glucose, galactose, fructose LIVER Galactose, fructose metabolism Glucose CIRCULATION Cellulose FAECES Transport 1. Facilitated diffusion (GLUT1-GLUT5) 2. Na+-dependent transport (low → high) 3. K+-ATPase pump (Passive diffusion) Glycolysis – Embden-Meyerhoff-Parnas Pathway Process of breakdown of glucose to pyruvate or lactate Site of glycolysis – cytosol Occurs in two phases ✔ Preparatory phase ✔ Pay off phase Purpose of glycolysis – to provide fuel in the form of ATP and intermediates for the metabolic pathways Preparatory phase 1. Glucose is converted to glucose 6-phosphate (G6-P) by the enzyme hexokinase and glucokinase - Irreversible and regulatory step Found in most tissues Predominantly in liver Acts when blood glucose level is low Acts when blood glucose level is high – ‘Glucostat’ Has a low Km (~ 0.1mM) Has a high Km (~ 10mM) Inhibited by G6-P (product inhibition) Not inhibited by G6-P at physiologic conc. 2. G6-P is isomerized to fructose 6-phosphate (F6-P) by the enzyme phosphoglucoisomerase – Reversible reaction. 3. F6-P is phosphorylated by ATP to fructose 1,6-biphosphate (F1,6-BP) with the help of the enzyme Phosphofructokinase (PFK I) – Irreversible and regulatory step 4. F1,6-BP is cleaved to form two triose phosphates, dihydroxyacetone phosphate (DHAP) and glyceraldehyde 3-phosphate (Gly3-P) by the enzyme aldolase. PFK I is activated by F6-P, AMP. Inhibited by ATP, citrate and glucagon PFK II is activated by F2,6-BP 5. DHAP is converted to gly3-P by triose phosphate isomerase. Aldolase is Zn-containing enzyme. Exists in number of isoenzymic forms. Aldolase A – most tissues; Aldolase B – liver and kidney Pay off phase 6. Gly3-P is oxidized to form a high energy compound, 1,3-biphosphoglycerate (1,3-BPG) by gly3-P dehydrogenase. Phosphorylation occurs at the expense of an inorganic phosphate - first energy conserving steps. Requires coenzyme NAD+ which is reduced in this step Reduced NADH+H+ is reoxidized via ETC where it yields 3 ATPs per molecule of NADH 7. High energy phosphate from 1,3-BPG is transferred to ADP by phosphoglycerate kinase – Reversible reaction and example for substrate level phosphorylation (SLP) 8. Phophoglycerate mutase shifts the phosphate group of 3-phosphoglycerate to carbon-2 to form 2-phosphoglycerate. 9. 2-phosphoglycerate is dehydrated to form a high-energy compound, phosphoenol pyrvate (PEP) by enolase Fluoride inhibits Enolase 10. High-energy phosphate from PEP is transferred to pyruvate by pyruvate kinase - Irreversible reaction and example for SLP Requires K+ along with Mg2+ as cofactors Activated by F1,6-BP, high carbohydrate intake and high insulin levels Inhibited by increased levels of cAMP, alanine, fatty acids, and acetyl CoA Regulated by covalent modification Significance of phosphorylation of glycolytic intermediates ❑ Phosphorylated molecules remain intact. The phosphate groups are ionized at pH 7.0 giving the intermediates a negative charge. Plasma membrane is impermeable to the charged molecules. Therefore, the phosphorylated molecules do not diffuse out. ❑ Phosphate groups conserve the energy. High-energy compounds such as 1,3-BPG and PEP donate groups to ADP to form ATP. ❑ Binding of phosphate groups to the active sites 9 of enzymes lowers the activation energy. The phosphate groups form complexes with Mg2+. The binding is specific for these complexes. Most of the reactions in glycolysis requires Mg2+. Anaerobic glycolysis Conversion of pyruvate to lactate by lactate dehydrogenase is an essential step Anaerobic glycolysis refers to ‘reoxidation of NADH without the requirement of oxygen – electron transport chain’ In exercising skeletal muscle, lactate is formed and released into blood. Difference between aerobic and anaerobic glycolysis Aerobic glycolysis Anerobic glycolysis Aerobic glycolysis occurs when oxygen is Anerobic glycolysis occurs when oxygen is plentiful scarce Muscle, RBCs, brain, gastrointestinal tract, Erythrocytes, lymphocytes, white blood cells, skin, kidney medulla, retina and heart. kidney medulla, tissues of eye and skeletal muscle Pyruvate is the final product Lactate is the final product Produces 8 ATPs Produces 2 ATPs Energetics (Refer next slide) Energetics (Refer next slide) 11 Energetics of glycolysis Aerobic glycolysis Anaerobic glycolysis Overall reaction Overall reaction Glucose + 2Pi + 2NAD+ + 2ADP → 2Pyruvate Glucose + 2Pi + 2ADP → 2Lactate + 2ATP + 2H2O + 2ATP + 2NADH+ + 2H+ +2H2O Formation and consumption of ATP Formation and consumption of ATP Regulation of glycolysis Hormonal Insulin stimulates glucokinase, PFK I and pyruvate kinase - favors glycolysis. Glucagon stimulates the reversal Availability of substrates + Supply of substrates such as glucose, glucose 1-phosphate, ADP, Pi and NAD affect glycolysis Oxidation-reduction + Reactions of glycolysis are dependent on ratios of NAD+: NADH + H and pyruvate:lactate, and availability of oxygen Key regulatory enzymes Glucokinase / Hexokinase Phosphofructose kinase (PFK) Pyruvate kinase ❑ Covalent modification (Reversible phosphorylation - Insulin dephosphorylates the key enzymes. Glucagon, epinephrine phosphorylates the key enzymes through cAMP dependent protein kinase.) Metabolic fate of glucose Glucose - The intracellular form of glucose is glucose-6- phosphate. Only liver cells have the enzyme glucose-6-phosphatase that dephosphorylates G-6-P and releases glucose into the blood for use by other tissues G-6-P can be oxidized for energy in the form of ATP and NADH G-6-P can be converted to acetyl CoA and then to fatty acids. Excess G-6-P is stored away as glycogen. G-6-P can be shunted into the pentose phosphate pathway to generate NADPH and ribose-5-phosphate. Disorders associated with glycolysis Heart muscle with poor glycolytic activity – ischemia Pyruvate kinase deficiency – hemolytic anemia PFK deficiency in skeletal muscle -fatigue Glycolysis occurs very rapidly in cancer cells – increased production of pyruvate – acidosis Hexokinase deficiency RBCs produces less amount of 1,3-BPG and 2,3-BPG. 2,3-BPG lowers oxygen affinity for Hb resulting in the release of oxygen in the tissue. Decrease in 2,3-BPG levels reduces the amount of oxygen available in the tissues Lactic acidosis – disease in which lactate is accumulated Lactate level is >5mM leads to decrease in blood pH to below 7.2 Occurs when there is collapse of the circulatory system, as in myocardial infarction, pulmonary embolism, and when there is a failure to provide enough oxygen to the tissues. Excess oxygen required to recover from a period of unavailability of oxygen is termed as oxygen debt. Lactate levels to measure oxygen debt and presence and severity of shock. 2,3-Bisphosphoglyceric acid Metabolic fate of pyruvate 1. Oxidative decarboxylation of pyruvate by pyruvate dehydrogenase complex an important pathway in tissues with a high oxidative capacity, such as cardiac muscle Pyruvate dehydrogenase irreversibly converts pyruvate, the end product of glycolysis, into acetyl CoA, a major fuel for the TCA cycle and the building block for fatty acid synthesis. 2.Carboxylation of pyruvate to oxaloacetate by pyruvate carboxylase is a biotin-dependent reaction. is important because it replenishes the citric acid cycle intermediates, and provides substrate for gluconeogenesis. 3.Reduction of pyruvate to ethanol (microorganisms) Conversion of pyruvate to ethanol occurs by the two reactions Decarboxylation of pyruvate by pyruvate decarboxylase occurs in yeast and certain other microorganisms, but not in humans. Enzyme requires thiamine pyrophosphate as a coenzyme, and catalyzes a reaction similar to pyruvate dehydrogenase. TCA (TriCarboxylic acid Cycle) – Krebs cycle – Citric acid cycle Requires acetyl CoA as substrate Acetyl group of the acetyl CoA is derived from the other pathways of metabolism such as oxidation of fatty acids, ketone bodies, proteins and most importantly, glycolysis Series of reactions through which the final oxidation of acetyl CoA to CO2 occurs. Site – Mitochondrial matrix Oxidation of pyruvate to acetyl CoA – oxidative decarboxylation Pyruvate is converted to CO2 and acetyl CoA and then acetyl CoA enters TCA cycle. This is the link between glycolysis and TCA Cycle Occurs in mitochondrial matrix Overall reaction is Pyruvate + NAD+ + CoASH →Acetyl CoA + NADH + H+ + CO2 Reaction is irreversible, rate-limiting and regulatory Regulation Increased by insulin Decreased by high ratios of ATP:ADP and NADH:NAD+, presence of less acetyl CoA and cAMP and conditions of starvation and diabetes mellitus Enzyme – Pyruvate dehydrogenase (PDH) multienzyme complex PDH is made up of 3 enzymes and requires 5 coenzymes –E1 – Pyruvate decarboxylase –E2 – Dihydrolipoyl transacetylase –E3 – Dihydrolipoyl dehydrogenase Coenzymes – TPP, Lipoic acid, FAD, NAD+ and CoA 1 Acetyl CoA combines with oxaloacetate (OAA) to form citrate with the help of citrate synthase Citrate inhibits the reaction 2 Citrate undergoes isomerization with the help of aconitase to produce isocitrate. 3 Isocitrate is oxidized to α-ketoglutarate (α-KG ) in an oxidative decarboxylation reaction forming CO2by isocitrate dehydrogenase (Key regulatory enzyme). Activated by ADP Inhibited by NADH 4 α-KG is converted to succinyl CoA by KG dehydrogenase multienzyme complex in the second oxidative decarboxylation reaction. CO2 is released and NADH + H+ are produced. 5 Succinyl CoA is cleaved to succinate by succinate thiokinase – Substrate level phosphorylation. GDP is converted to GTP 6 Succinate is now oxidized to fumarate by succinate dehydrogenase. FADH2 is the coenzyme – yields 2 ATPs – through ETC 7 Hydration of fumarate to malate occurs with the help of fumarase. 8 Malate is oxidized with the help of malate dehydrogenase. Oxaloacetate is regenerated. NADH+ is the coenzyme – yields 3 ATPs – through ETC 24 Energetics of TCA Cycle Reaction catalyzed by Method of ATP production No. of ATP formed Pyruvate dehydrogenase Oxidation of NADH via respiratory chain 3 Isocitrate dehydrogenase Oxidation of NADH via respiratory chain 3 α-KG dehydrogenase Oxidation of NADH via respiratory chain 3 Succinate thiokinase Oxidation at substrate level (GTP later 1 converted to ATP) Succinate dehydrogenase Oxidation of FADH2 via respiratory chain 2 Malate dehydrogenase Oxidation of NADH via respiratory chain 3 15 ATPs per pyruvate 2 pyruvate x 15 ATP = 30 ATP One turn of TCA Cycle produces 12 ATPs starting from acetyl CoA When starts from pyruvate, oxidative decarboxylation produces 3 ATPs. Therefore, 15 ATPs are produced Since two molecules of pyruvate enters TCA cycle, totally 24 ATPs from acetyl CoA and. 30 ATPs from pyruvate Disorders associated with TCA Cycle Defect in PDH is fatal – known as thiamine responsive PDH deficiency Symptoms include lactic acidosis and neurologic disorders Treatment involves large doses of thiamine, lipoic acid and ketogenic diet Fumarase deficiency - affects skeletal muscle and brain Symptoms include sever neurologic impairment, encephalopathy and dystonia Autosomal recessive disorder. Urine contains abnormal amounts of fumarate Pyruvate dehydrogenase Metabolism of fructose Fructose forms a very important part of our diet – fruits, table sugar and sweets Fruits contain sucrose which is digested by sucrase to form glucose and fructose. Fructose enters the epithelial cells by facilitated diffusion Fructose can also be synthesized in the body by the sorbitol pathway. It is the source of energy of sperms. Fructose metabolism occurs in liver, intestinal mucosa, and kidney Fructose is phosphorylated either by fructokinase or hexokinase to F1-P Phosphorylation by fructokinase is the primary mechanism F1-P formed is acted upon by aldolase B. Aldolase A cleaves fructose to Gly3-P and DHAP – enters glycolysis Metabolism of fructose Sorbitol pathway for D-glucose NADPH + H+ Aldose reductase NADP+ synthesis of fructose D-Sorbitol NAD+ Sorbitol dehydrogenase NADH + H+ D-fructose Diet Also called polyol pathway Sorbitol is formed from glucose by the action of aldose reductase – lens tissue, retina, placenta, RBCs, ovaries and spermatozoa. Enzyme, aldose reductase is not found in the liver. In liver, ovaries, sperm and seminal vesicles, sorbitol dehydrogenase converts sorbitol to D-fructose. Metabolism of galactose Lactose on hydrolysis by β–galactosidase produces glucose and galactose Lactose is an important component of milk and milk products Galactose is also obtained from the degradation of glycoproteins and glycolipids Galactose gets phosphorylated to galactose 1-Phosphate by the enzyme galactokinase Galactose 1-P is converted to UDP-galactose in exchange with UDP-glucose by the enzyme galactose 1-phosphate uridyltransferase. The UDP-galactose then gets converted to its C-4 epimer, UDP-glucose by UDP- hexose 4-epimerase This UDP-glucose is used for the formation of glycogen and UDP-glucuronate UDP-galactose and UDP-glucuronate are used for the synthesis of glycolipids, glycoproteins and glycosaminoglycans The enzyme, aldose reductase converts galactose to the alcohol, galactitol. Galactose 1-P is converted to UDP-galactose in exchange with UDP-glucose by the enzyme galactose 1-phosphate uridyltransferase. Metabolic defects in fructose and galactose metabolism Essential fructosuria - is due to the deficiency of fructokinase Hereditary fructose intolerance – absence of aldolase B – (Severe hypoglycemia, vomitting and jaundice – treatment is removal of fructose and sucrose from diet). Lactose intolerance – deficiency of galactosidase (Found in premature infants, in conditions of mucosal damage – also due to surgical removal of intestine). Non-classical galactosemia – galactokinase deficiency (in diabetes causes cataract – accumulation of galactose in lens of the eye). Classical galactosemia – deficiency of galactose – 1-phosphate uridyl transferase. (autosomal recessive disorder. – enlargement of liver, jaundice, vomitting, mental retardation and cataract). Alcohol metabolism Alcohol is metabolized in liver Alcohol is converted to acetaldehyde by alcohol dehydrogenase Acetaldehyde is then converted to acetate by aldehyde dehydrogenase. It causes accumulation of acetaldehyde in blood, results in flushing, tachycardia , hyperventilation & nausea. From above 2 reactions there is massive increase cytosolic NADH Abundance of NADH favors reduction of pyruvate to lactate & oxaloacetate to malate both are intermediates of gluconeogenesis. This can precipitate hypoglycemia, particularly in persons who have depleted liver glycogen stores. Alcohol consumption in individuals those fasted or in prolonged Strenuous exercise can produce hypoglycemia NAD + NADH + H+ Ethanol Acetaldehyde Alcohol NAD+ dehydrogenase Aldehyde dehydrogenase NADH + H+ Acetate Lactate dehydrogenase Pyruvate Lactate NADH NAD+ Gluconeogenesis + H+ Glucose References : Text Book of Biochemistry for Medical Students by DM Vasudevan. Harper’s Biochemistry 26th edition. Learning Outcomes MBBS YEAR 1 2024 MIBS 65101 At the end of the lecture, you should be able to Classify the types of carbohydrates. Outline the structure, and functions of lactose, maltose, sucrose, dextran, dextrin, inulin, starch, glycogen, cellulose. Describe the glycolytic pathway and its regulation. Describe the steps of the TCA cycle and the importance of the pathway. Describe the metabolism of fructose, galactose and alcohol and its clinical relevance Thank MBBS YEAR 1 2023 MIBS 65101 You LET ME KNOW IF YOU HAVE QUESTIONS OR CLARIFICATIONS.